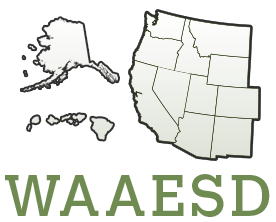
W181: Modifying Milk Fat Composition for Improved Manufacturing Qualities and Consumer Acceptability
(Multistate Research Project)
Status: Inactive/Terminating
W181: Modifying Milk Fat Composition for Improved Manufacturing Qualities and Consumer Acceptability
Duration: 10/01/1999 to 09/30/2004
Administrative Advisor(s):
NIFA Reps:
Non-Technical Summary
Statement of Issues and Justification
STATEMENT OF THE PROBLEM:
The committee members will address cooperatively modifying milk fat composition to enhance manufacturing qualities and to address consumer concerns. To accomplish this, efforts will be coordinated to 1) to characterize metabolic regulation of milk fat synthesis and identify those factors, including genetic, that influence functional and nutritional attributes of milk fat; 2) to document changes in milk fat composition induced by manipulating the diet and environment of the cow; and 3) characterize the quality of modified milk fat for manufacturing, sensory, and nutritional properties.
JUSTIFICATION:
Dairy products are an important source of vital nutrients in the human diet. Nevertheless, many health-conscious consumers perceive dairy products to contain excessive amounts of total fat, saturated fat, and cholesterol. Butter and other high-fat dairy products are excluded from diets designed to decrease blood cholesterol and prevent or treat coronary heart disease (Ney, 1991). Dairy products provide only 15% of the total fat in the diet, but 25% of the total saturated fat (ODonnell, 1993).
This proposed project addresses four goals/objectives outlined by FAIR 95 (1993) which address enhancing the quality of dairy products for human consumption. They are:
Goal 1. Identify and quantify societal concerns about food products from animals and production systems to enhance communication between consumers and the food industry.
Objective 1. Identify societal concerns that affect the marketplace through food choices.
Goal 2. Meet consumer needs in domestic and international markets for competitive and high quality food products from animals.
Objective 2. Enhance the quality of food products from animals.
Goal 3. Develop integrated food animal management systems and animal health systems that support efficient, competitive, and sustainable production of safe and wholesome food consistent with animal and environmental well-being.
Objective 2. Develop research data bases and integrate them into decision-support systems for producers.
Goal 5. Improve industry wide, quality control systems for food products from animals.
Objective 3. Identify human nutrition needs for specific consumer populations in relationship to the composition of food products from animals.
Further, the proposed project addresses priority research initiatives on 1) nutrition, food safety, and health; 2) processes and products; and 3) animal systems identified by the Strategic Agenda for the State Agricultural Experiment Stations, Opportunities to Meet Changing Needs (ESCOP, 1994).
Approximately 64% of the fatty acids in milk are saturated (Palmquist et al., 1993). Recent research has shown, however, that not all saturated fatty acids increase blood cholesterol in humans. Fatty acids of less than 12 carbon atoms are neutral or actually may decrease cholesterol. Stearic acid (C18:0) acts similarly to oleic acid (cis-C18:1) to decrease cholesterol (Ney, 1991). Only three saturated fatty acids (lauric, C12:0; myristic, C14:0; and palmitic, C16:0) now are considered to be hypercholesterolemic. These three fatty acids constitute about 44% of total milk fatty acids. According to a group of nutritionists from industry and academia (ODonnell, 1989), the ideal milk fat for human health would contain < 10% polyunsaturated fatty acids, < 8% saturated fatty acids, and 3 82% monounsaturated fatty acids. Fatty acids with less than 12 carbons are not included in this total. Concerns about the negative effects of trans-isomers of unsaturated fatty acids (Ney, 1991) indicate that any increases in poly- or monounsaturated fatty acids in milk should be primarily in the cis configuration. However, recent research has shown that the predominant trans isomer in milk fat (t-11 octadecenoic) is an important precursor of conjugated linoleic acid (CLA) (Santora et al., 1998).
The role of CLA (active isomer believed to be cis-9, trans-11 octadecadienoic acid) in diet and health has become an important issue recently upon recognition of its role as a potent anticancer agent, and perhaps many other physiological effects (Chin et al., 1992; Clement et al., 1994; Ip et al., 1994; Jiang et al., 1996; Parodi, 1997). Recently, it has been shown that trans-11 octadecenoic acid, an important fatty acid in milk fat, is efficiently converted to CLA in the body (Corl et al., 1998; Griinari et al., 1998; Santora et al., 1998). Thus, the trans-11 monoene content enhances the value of milk fat as a source of CLA.
Real or perceived concerns about the effects of milk fat on health and well-being not only decrease the economic value of dairy products (and thus producer incomes), but more importantly may compromise consumption of highly nutritious foods. Dairy products contribute the following percentages of total intakes for adults: Ca, 42-46; P, 18-23; K, 13-15; Mg, 10-13, and Zn, 10-12. For adolescents and children, dairy foods contributed much greater percentages of these nutrients (ODonnell, 1993). The importance of full fat dairy products in the diet is heightened further by the discovery of the role of CLA in health. Thus, it is important for public health and well-being that consumption of dairy products be maintained or increased so that intake of important nutrients is not compromised.
Modification of the fatty acid profile of milk should be beneficial to human health and improve the image of dairy products to health-conscious consumers (Noakes et al., 1996). As a consequence, sales of dairy products should increase, which would be of direct benefit to dairy producers and processors. Research to this end has been encouraged in several forums on research priorities, including the NRC Board on Agriculture Committee on Technological Options to Improve the Nutritional Attributes of Animal Products (Designing Foods) (1988), the FAIR 95 agenda (1993), ESCOP (1994), and a round table discussion by prominent nutrition researchers (Berner, 1993b). Although it is not likely that the ideal milk fat composition could be achieved, manipulation of the composition of milk fat is possible through feeding practices for dairy cows (Grummer, 1991; Palmquist et al., 1993). For example, feeding supplemental fats may increase contents of C18:0 and C18:1 while decreasing contents of C14:0 and C16:0; at the same time, however, content of more desirable short-chain fatty acids also may be decreased. Karijord et al. (1982) reported the composition and its variance of milk fat from Norwegian dairy herds. The coefficients of variation for individual fatty acids of milk fat ranged from 9 - 22%. Stage of lactation and month of season accounted for 10 - 25% of the variance, with the remainder being attributed to individual animal variation. Stage of lactation was more important than season with regard to variance. Nutritional inputs were not considered in the model. Karijord et al. (1982) concluded that genetic approaches might be used to alter milk fat composition. Although Gibson (1991) concluded that practical possibilities are limited to make changes through traditional breeding approaches or transgenic technologies, more recent research has documented differences among breeds in extent of unsaturation of dietary fatty acids (Beaulieu and Palmquist, 1995; DePeters et al., 1995). Further, progress in inducing transgenic animals with unique capability to secrete specific agents in milk is developing rapidly (Proceedings, Lactation Workshop, ADSA/ASAS annual meeting, 1998). Increased knowledge of the control and regulation of milk fat composition by mammary tissue is needed in order to develop, through rational scientific approaches, new dietary strategies for alteration of milk fat. Quantifying changes produced by defined nutritional and environmental manipulations in carefully designed and coordinated scientific experimentation will allow prediction of changes in milk fat composition that could be expected by feeding specialized diets to dairy cows.
Milk fat composition also can be altered by manufacturing processes, such as fractionation, blending, or interesterification. These practices, however, may compromise flavor, mouthfeel, or other physical properties of the modified dairy products (Berner, 1993a). Increased unsaturation of milk fat may cause problems with oxidative stability (Charmley and Nicholson, 1994; Granelli et al., 1998) which may or may not be alleviated by supplementing with vitamin E (Focant et al., 1988) and may cause rejection of milk by consumers (Palmquist, 1997). Also, the variation in milk fat composition which now exists in commercial milk causes difficulty to produce consistent milk fat fractions. Consistent, high quality milk fat fractions are required to develop some new dairy foods. Technologies also exist or are being developed to remove cholesterol from milk fat; these technologies may increase consumer acceptance but have limited nutritional impact because dairy products are a minor source (5%) of dietary cholesterol (Berner, 1993a).
A coordinated effort to study nutritional regulation and manipulation of milk fat composition offers the best opportunity for successfully producing milk of altered fat composition. Such an ambitious goal likely will not be achieved by a single investigator or institution. Cooperative research through the regional research system is a rational approach to focus attention and progress on this important topic. Usefulness of the data generated by this approach will be extended by incorporation into models of feeding and metabolism of dairy cattle. Specialized technologies to evaluate the composition and functionality of milk, such as determination of positional isomers and manufacturing characteristics, would best be shared through cooperative research to avoid unnecessary duplication of expensive equipment or specialized labor. Furthermore, it is essential that any changes in milk fat composition be evaluated for resultant effects on flavor, texture, and processing characteristics of milk and dairy products. Because few experiment stations possess dairy products research centers with such capabilities, a cooperative approach will be necessary to properly evaluate milk with altered fat composition. Inclusion of the Cooperative Extension Service in the activities will facilitate and enhance communication of progress to the public.
Related, Current and Previous Work
Genetic selection for increased milk fat percentage leads to increased proportions of short-chain fatty acids in milk fat and decreased proportions of long-chain fatty acids (Karijord et al., 1982). Consistent with this, milk fat from Jersey cows has higher amounts of short and medium chain fatty acids (Beaulieu and Palmquist, 1995; DePeters et al., 1995) and lower ratios of cis 9 18:1/18:0 than milk fat from Holsteins. Milk fat composition is strongly influenced by stage of lactation; proportion of short chains is low initially and increases until at least 8 to 10 wk into lactation. Seasonal and regional differences in milk fat composition are measurable, most likely because of local differences in feed supplies (Palmquist et al., 1993).
The fundamental processes of milk fat synthesis are well established and explain the occurrence of high amounts of saturated fatty acids in milk. Milk fat is synthesized from fatty acids (FA) which are obtained from blood or by de novo synthesis in the mammary gland. Fatty acids synthesized de novo contain 4 to 16 carbons and are saturated. Blood FA are derived from diet or from lipolysis in adipose tissue. Approximately 50 to 60% of milk FA are of dietary origin; therefore, FA composition of milk can be influenced by diet. Modifications of dietary FA prior to incorporation into milk fat include biohydrogenation of unsaturated FA in the rumen and desaturation of stearic acid by intestinal, adipose, or mammary tissue. Consequently, milk FA tend to be lower in polyunsaturated FA and higher in oleic acid than is dietary fat.
The most thorough modern summaries of the distribution of FA in milk fat and dairy products are by Jensen et al. (1991) and Jensen (1992, 1995). A CRIS search revealed 50 projects related to milk production and/or feeding effects on milk composition, and/or milk fat quality. Of these, 23 were projects of members of the present W-181 Committee; 13 were from non-members in project states, and 14 were from non-project states. The Committee is making an effort to include more investigators, as well as the Cooperative Extension Service who have similar interests from both project and non-project states. Other dairy-related regional research projects include NC-185, ""Metabolic Relationships in Supply of Nutrients for Lactating Cows"", which
is focused on rumen metabolism and supply of amino acids for milk protein synthesis. Two W-181 members (OH and SD) are also active members of NC-185, and both committees share the same CSREES representative, which should enhance coordination and reduce duplication between the committees. A second regional committee, NE-132 ""Environmental and Economic Impacts of Nutrient Flows in Dairy Forage Systems"" concerns forage use and the environment; therefore it does not address issues of product composition and quality.
Metabolic Regulation.
German et al. (1997) have provided a succinct, current summary of milk fat biosynthesis. The physical properties (primarily fluidity, or melting point) of milk fat are critical as the fat must be liquid at body temperature. Three metabolic processes within the mammary gland influence the fluidity of the milk fat: 1) chain length of FA synthesized de novo; 2) desaturation of stearate to oleate; 3) positional distribution of FA on glycerol. Considerable information on the metabolic processes and regulation of milk fat synthesis is available, with comprehensive studies from the laboratories of Kinsella, and Knudsen and colleagues. Pathways and regulation of de novo synthesis are by the classical pathways (Dils, 1983), with acetate, lactate, and b-OH-butyrate as primary carbon sources, and glucose, acetate and lactate as the primary sources of reducing equivalents (Forsberg et al., 1985 a,b).
Regulation of the pattern (chain length) of FA synthesized de novo is not well-defined. Many non-ruminant mammalian species regulate synthesis of short- and medium-chain fatty acids (SCFA and MCFA, respectively) by a specific enzyme (thioesterase II) which cleaves the MCFA from the fatty acid synthetase (FAS) enzyme complex (Smith, 1980). However, ruminants do not possess this enzyme; rather, the mammary gland FAS exhibits both medium-chain thioesterase and transacylase activity (Knudsen and Grunnet, 1982). The transacylase which transfers the activated primer chain to the FAS complex has broad chain-length specificity, so that there is competition both for transfer to and removal from the FAS of acyl chains containing from 2 to 12 carbons. Relative distribution is influenced by the affinity of the transacylase for substrates [highest affinity is for butyrate (Knudsen and Grunnet, 1980)] and seems to be modified by the concentration of malonyl CoA (Hansen and Knudsen, 1980). Malonyl CoA concentration, in turn, is regulated to some extent by the concentration of long-chain acyl CoA in the cell (Bauman and Davis, 1974). Relative supply of palmitic (C16:0) vs oleic (C18:1) acids may influence the pattern of SCFA and MCFA synthesized (Hansen and Knudsen, 1987). Although uptake of long chain fatty acids in themselves inhibit de novo synthesis of shorter chain fatty acids (Enjalbert et al., 1998), the trans monoene fatty acids have received extensive attention recently as the central agent in depressed milk fat synthesis (Wonsil et al., 1994; Gaynor et al., 1995). More recently, the trans-10 monoene was implicated, rather than trans-11 (Griinari et al., 1998). Conjugated linoleic acid also has been implicated as a potent inhibitor of de novo fatty acid synthesis (Loor and Herbein, 1997).
Saturated LCFA absorbed from the intestine would cause milk fat to be solid if excessive amounts were incorporated. Melting point is lowered by activity of stearoyl CoA desaturase (Kinsella, 1972) to convert stearic acid (m.p. 700C) to oleic acid (m.p. 5-70C); to a lesser extent, palmitic acid is desaturated by the same enzyme. Trans monoenes may inhibit the desaturase activity (Enjalbert et al., 1998). As very little polyunsaturated FA (typically high in fluidity-sensitive membranes) are available for synthesis of milk fat triglyceride, synthesis of SCFA and MCFA and stearoyl-CoA desaturase activity to modify milk fat fluidity is critical. Contributions of SCFA, MCFA, and LCFA to milk fat synthesis seem to be regulated in ruminants, but not tightly so, as variations in fluidity of milk fat are documented (Banks et al., 1989).
The third factor which influences physical properties of milk fat is the distribution of the various FA on the glycerol molecule (Palmquist et al., 1993). Although specificity of acyltransferases has been demonstrated (Marshall and Knudsen, 1977), there is little documentation as to whether transferase activity can be modified. Feeding C18:2 protected from ruminal biohydrogenation decreased the proportion of C16:0 and increased the proportion of C18:2 at the sn-2 position of glycerol (Jensen et al., 1991). Trans monoenes are equally distributed at sn 1 and 3, but are not found at the sn-2 position (Woodrow and DeMan, 1968).
Dietary Regulation
Extensive information on the effects of diet on milk fat composition were reviewed by participants in this proposed regional project (Grummer, 1991; Palmquist et al., 1993, Kennelly, 1996). Milk fat composition (distribution of the individual fatty acids) varies by breed of cow, stage of lactation, and diet (Palmquist et al., 1993). Only the last is readily manipulated by management. The most dramatic changes in milk fat composition are brought about by feeding supplemental fat. Feeding fat that is rich in 18 carbon FA increases C18:0 and C18:1 content of milk fat and reduces the SCFA content of milk via a reduction of de novo fatty acid synthesis (Enjalbert et al., 1998). Oleic acid (C18:1) content of milk can be increased substantially if the cow is fed high levels of substrate (C18:0) for stearoyl-CoA desaturase (Bickerstaffe et al., 1972). Palmitic acid (C16:0) content of milk fat is typically reduced when feeding supplemental fat unless the supplement is rich in C16:0. Increases in C18:0, C18:1, and decreases in C16:0 content of milk fat are considered to be positive changes in the milk fatty acid profile and can be achieved by changing the diet of the cow. However, although Jenkins (1998) reported 48% oleic acid in milk when oleamide was fed, the extent to which milk fat can be manipulated toward a more desirable fatty acid profile has not been determined. For example, very few dose response curves to supplemental fat have been generated. The few that have been reported have indicated that maximum changes in fatty acid profile have not been obtained within the levels of supplemental fat tested (LaCount et al., 1994). There are limitations to the amount of fat that can be supplemented to dairy diets due to inefficiencies in postruminal lipid digestion (Palmquist, 1994). Methodologies for increasing absorption of fat from the small intestine need to be identified. Although several methods for dietary manipulation of milk fat are available, the magnitude of change that can be achieved by each method is largely undefined. Combining methods that take advantage of different biological processes have not been attempted but should be. A model to predict composition of milk fat from diet composition has been published (Hermansen, 1995) and has been used to develop modified fat dairy products for human metabolic studies (Tholstrup et al., 1998). A data base to refine and challenge the model needs to be developed.
In addition to feeding supplemental fat, milk unsaturated FA content (particularly C18:1) can be increased by reducing biohydrogenation in the rumen. Reducing ruminal pH by feeding high levels of nonstructural carbohydrate will limit microbial conversion of unsaturated fatty acids to C18:0 in the rumen (Latham et al., 1972). Encapsulation of fat to prevent biohydrogenation is a strategy that was examined in the early 1970s (McDonald and Scott, 1977) and needs further examination now that there is increased emphasis on modifying milk fat composition. Chemical modification of polyunsaturated fatty acids to reduce microbial hydrogenation has been developed (Jenkins et al., 1996; Jenkins, 1998), but needs to be refined and approved for commercial use. Linoleic acid can be doubled in milk fat by feeding whole soybeans (Tice et al., 1994). Computer models of ruminal lipolysis and biohydrogenation need to be developed in order to predict responses to changed feeding inputs.
Product Quality
The most significant changes in milk fat quality relate to rheological (melting) properties, which influence numerous aspects of character and quality of manufactured dairy products (Mortensen, 1983; German et al., 1997). The types of fatty acids present in milk fat can influence the flavor and physical properties of dairy products, as well as results of analytical tests for determination of milk fat (Barbano and Lynch, 1987; Stegeman et al., 1992). The mid-infrared spectroscopic method underestimated the fat content of milk that was higher in unsaturated fatty acids (Stegeman et al., 1991). The unique SCFA of milk fat, particularly butyric acid, are important for flavor development in some cheese and fermented dairy products (Barbano and Lynch, 1987). The ratio of saturated to unsaturated fatty acids and the ratio of short- to long-chain fatty acids can affect the meltability and spreadability of butter, and also may affect the body/texture and meltability of cheese. Changing fatty acid composition affects milk fat melting directly, because each fatty acid has a characteristic melting point, and indirectly, by influencing the array of fatty acids in the various positions of the glycerol backbone. This structure is the most important determinant of crystallization behavior and hardness of milk fat, as well as melting point (Connolly and Murphy, 1994). Currently, variability of triglyceride structure in commercial milk fat compromises quality of products produced by crystallization/fractionization of milk fat (Laakso et al., 1992).
Sensory evaluation indicated that butters produced from cows fed high oleic sunflower seeds and regular sunflower seeds were equal or superior in flavor to the control butter (Middaugh et al., 1988). The high oleic sunflower seed and regular sunflower seed treatment butters were softer, more unsaturated, and exhibited acceptable flavor, manufacturing, and storage characteristics. Sensory evaluation of Cheddar cheese indicated that extruded soybean and sunflower diets yielded a product of quality similar to that of the control diet (Lightfield et al., 1993). Cheese made from milk obtained with extruded soybean and sunflower diets contained higher concentrations of unsaturated fatty acids while maintaining acceptable flavor, manufacturing, and storage characteristics. Baer (1991) anticipated that the total unsaturated fatty acid content could be increased beyond the amounts achieved to date when feeding unsaturated fat sources and without adversely affecting flavor or product processing properties. However, recent industry complaints of oxidized flavor have led to investigation of the role of supplemental fat. Feeding whole soybeans increased 18:2 and 18:3 by 60 and 100%, respectively, and was associated with development of oxidized flavor (Palmquist, 1997).
Research is limited concerning the processing and sensory properties of milk and dairy products containing higher concentrations of unsaturated fatty acids produced by cows fed supplemental fat. Wong et al. (1973) processed milk from cows fed a protected safflower and oil-casein fat source to produce a Cheddar cheese containing 30% linoleic acid. Experimental cheese possessed body and flavor defects; however, an acceptable Cheddar cheese was produced from polyunsaturated milk.
Objectives
-
To identify and characterize important regulatory steps in fatty acid synthesis and desaturation and their positional distribution on glycerol in milk fat
-
To quantify modification of milk fat composition by manipulating the diet of the cow
-
To characterize the effects of modified milk fats on physical, chemical, manufacturing, and sensory properties of dairy products
-
Methods
Objective 1: Progress was made in the past 5 years to identify important regulatory steps in milk synthesis, for example the importance of stearoyl-CoA destaurase activity in the mammary gland, and the role of trans fatty acids to regulate fatty acid synthesis, however, much remains to be learned about regulation of these activities and their interaction with different sources and types of dietary fat. We have only begun to attack the laborious task of identifying effects of dietary fats on positional distribution of fatty acids on glycerol. There is a particular need to develop sound biochemical bases to understand metabolic regulation of the synthesis of individual milk fatty acids, in particular 12:0, 14:0 and 16:0, which are of specific public concern today. Objective 1 addresses these needs. Regulatory aspects of fatty acid synthesis and incorporation into triacylglycerol will be studied in mammary tissue explants, in isolated cells, in cell culture, or by preparing subcellular components of mammary tissue using standard enzymatic procedures (IL, VA). In certain unique studies, metabolic limits for incorporation of specific fatty acids (varying in unsaturation or chain length) in vivo will be characterized by infusing these intestinally or intravenously (IL). Kinetic characteristics of stearoyl-CoA desaturase will be determined in microsomal fractions of mammary tissue (IL), and factors regulating SCFA synthesis will be determined in homogenates or explants (IL). In addition to desaturase activity in mammary cells, changes in activities of fatty acid synthetase and acetyl-CoA carboxylase, and quantities of mRNA for all three enzymes will be determined (VA). It is important to determine whether inhibition of milk fat synthesis is regulated at the level of fatty acid synthesis or at the level of glyceride synthesis. Influence of dietary trans-18:1 on fatty acid synthesis by an established mouse mammary epithelial cell line, which produces neutral lipids when stimulated by lactogenic hormones during growth on an extracellular matrix, will be studied. The effects of cis and trans isomers of unsaturated fatty acids on the promoter region of the stearoyl-CoA desaturase gene will be evaluated in bovine mammary cell cultures (VA). This group is studying rates of fatty acid elongation and fatty acid incorporation into triacylglyceride, activities of acetyl-CoA carboxylase and stearoyl-CoA desaturase, and plasma membrane fluidity in response to varying amounts of 18:0, cis-18:1, and trans-18:1 in the incubation medium. These studies will be developed further in a bovine mammary cell (MAC-T) line to determine the extent to which concentrations of lipid precursors regulate rates of de novo fatty acid synthesis and desaturation of exogenous stearic acid. Collaboration (IL and VA) to develop isolated cells (Hansen et al., 1986) as a metabolic model to study regulation of milk synthesis will be developed. Factors influencing desaturation of trans-11 18:1 by stearoyl-CoA desaturase to form CLA will be investigated. The role of trans-10 18:1 in inhibition of fatty acid synthesis will be investigated (MD, NY). Objective 2: Greatest progress in the past 5 years was toward this objective. Nevertheless, new understanding of ruminal and mammary lipid metabolism influence how different fats are used in feeding systems. We will need to characterize these in terms of feeding management. Further, this objective is integral to achieving progress toward objectives one and three. Several investigators will use dietary fat supplements to manipulate milk fat composition, with particular emphasis to decrease 12:0, 14:0 and 16:0, and to increase cis 18:1 and CLA (CA, ID, IL, OH, SC, SD, UT, VA). Lipolysis of glycerides and biohydrogenation of unsaturated fatty acids by ruminal microorganisms will be quantified by in vitro techniques, and the data will be used to develop simulation models of these processes, to improve prediction of feeding effects on unsaturation of milk fat. Experimental designs will utilize multiple levels of fat supplementation to develop dose response curves so that diet fat effects on milk fat composition may be quantitated. Stage of lactation of cows will be defined in order to remove confounding effects of adipose mobilization on milk fat composition. Correlary studies will examine effects of adipose tissue mobilization on milk fat composition. Factors influencing the mammary uptake of individual fatty acids will be determined by arterio-venous difference across the mammary gland (VA). Dietary influence on sphingomeyelin content of milk will be studied (IL). Effort to define the role of different fats on regulation of feed intake, including function of CCK, will be made (IL, AB). Objective 3: As described in our summary of the initial project, progress toward achieving Objective 3 was limited because of limited participation of lipid chemists. Additional milk chemists have indicated their intention to join the project and new knowledge has caused investigators to become more focused on particular questions of milk chemistry. Much is to be gained by maintaining this objective in the project. Milk and fat from the core group of feeding studies (Objective 2) will be sent to several of the cooperating milk processing research groups to evaluate changes in milk fat composition on manufacturing quality and consumer acceptance. These interactions will be developed and strengthened through the regional process. Milk obtained from studies addressing Objective 2 will be utilized to examine effects on flavor of milks with increased CLA, trans fatty acids, or n-3 fatty acids and on yield and flavor of low fat cheese (SD). Influence of increasing milk fat unsaturation on susceptibility to spontaneous flavor (SOF) will be quantified (OH). Positional arrangement of fatty acids on the glycerol molecule will be determined when appropriate (CA). Effects of increased fat unsaturation on milk fat globule size and physical characteristics will be determined (IL, NY). Milk from all studies will be used to update a national data base on seasonal, regional, and feeding effects on composition of the milk supply (NY). Regionality: D. Regionality will bring together scientists competent in all aspects of our concern for modifying milk fat: 1) metabolic regulation of synthesis; 2) predicting milk composition change from feed inputs; 3) evaluating effects of changing milk fat composition on product quality, and applying new knowledge to product development. Cooperation among investigators will be encouraged in many ways: 1) methodologies and gene probes will be standardized among groups studying metabolic regulation at the cellular level; 2) feeding studies will be regionalized to provide a sufficiently large data base to develop appropriate response curves to fat supplementation; 3) unique methodologies will be shared to obtain the maximum information from each study; e.g., some laboratories lack the capability to separate the cis/trans isomers of unsaturated fatty acids; this can be provided by other labs through sharing of samples; 4) milk from feeding studies will be provided to cooperating laboratories to determine effects of changed milk fat composition on product quality. Regional cooperation will enhance exchange of milk products for study. Thus, utilization of sophisticated equipment (NMR) and methodologies (triacylglycerol structure, sensory studies) will be enhanced and applied to a wider range of modified milk fats than would be possible in any individual laboratory. E. Interdependency of states is illustrated by sharing of information from methodologies unique to certain laboratories: a. intestinal cannulae (IL) and postruminal infusion (IL, MD, VA) b. separation of cis/trans isomers of milk fatty acids (CA, MD, OH) c. positional analysis of glyceride structure (CA) d. collaborative development of mammary cell culture systems (IL, VA). Uniform Methodologies Feeding trials will be designed to develop dose response curves to diet manipulation. All fat quantification will include total fatty acid analysis. Milk fatty acid analysis will utilize techniques that preserve and quantify short chain fatty acids (from butyric), and will include separation of the cis/trans isomers of C18:1 and of CLA.Measurement of Progress and Results
Outputs
- As documented in our Critical Review, important progress has been made by our Committee during the past five years in understanding the regulation of milk fat synthesis and composition, and efforts have been initiated to bring this knowledge to commercial practice. Techniques and understanding developed during the first five years of this project will be used to gain knowledge at an even accelerated pace. Interest by industry to commercialize dairy products with modified fat composition has increased and we expect to see more of these products available in the market place. Further, the committee has contributed to documentation of the important role of milk fat in a healthy diet. Through public information systems developed by this Committee, (Scientific Symposia, WEB page) public distribution of this information will be increased.