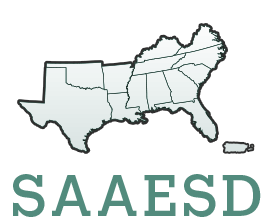
OLD S1071: A framework for secondary schools agriscience education programs that emphasizes the STEM content in agriculture
(Multistate Research Project)
Status: Inactive/Terminating
OLD S1071: A framework for secondary schools agriscience education programs that emphasizes the STEM content in agriculture
Duration: 10/01/2017 to 09/30/2022
Administrative Advisor(s):
NIFA Reps:
Non-Technical Summary
Statement of Issues and Justification
While many colleges of agriculture have experienced an increase in student enrollment over the past decade, fewer students maintain their agricultural focus through successful placement in an agriculturally-based scientific position upon graduation (Dyer, Lacey, & Osborne, 1996). The focus of this multistate research project is to revitalize an interest in agriculture as a career path and ensure secondary school students have the requisite competencies to succeed in college and careers. The end result will be an abundant supply of well-educated workers in careers that require agricultural scientific knowledge.
Currently, a shortage of scientists for agricultural positions exists throughout the country. Employment data from CSREES Employment Opportunities for College Graduates in the U.S. Food, Agricultural, and Natural Resources System (CSREES, 2005) projected a deficit of nearly 3,000 graduates per year for 2005-2010. The subsequent report of career opportunities for 2010-2015 (NIFA, 2010) projected an even greater deficit. Additionally, CSREES reported 25% of all job openings in the agricultural industry require scientific and engineering expertise. These projections have come to fruition as leading agriculture-based corporations indicate they cannot find suitable graduates with an agricultural background and scientific expertise. Industries seeking skilled workers for scientific agriculturally-based positions have been presented with application pools that contain a bi-polar skillset; approximately 40-45% of applicants have been graduates from “allied higher education programs such as biological sciences, engineering, business, health sciences, communication, and applied technologies (CSREES, 2005, p. 3), while just over half of the applicants had degrees in agriculture (CSREES, 2005).
Compounding the issue of recruiting and preparing qualified graduates to enter careers in agricultural sciences is the increasing demand for workers with scientific expertise by numerous career areas. Science, technology, engineering, and math (STEM) occupations are critical to the continued economic competitiveness of the United States (Carnevale, Smith & Melton, 2011). The demand for traditional STEM workers will continue to grow. But the increasing demand for STEM talent allows for and encourages the disbursement of students and workers with STEM competencies across various career paths. Some workers will voluntarily be diverted to other career areas, some may be diverted to other areas involuntarily (by not meeting expectations), and some will continue their education in a STEM field. However, those career paths cannot necessarily be predicted, so it is paramount for STEM-related programs to be on the cutting edge in terms of the skills and abilities needed to perform at some level of occupation and education.
Opportunities for educators and industry leaders to expose potential employees to the benefits of and skills used in the diverse array of agricultural careers are great, and occur across a broad timeline, both before and after entering the adult workforce. In order to increase the number of students transitioning into post-secondary education and employment, many secondary schools focus on career exploration and preparation, often mimicking colleges and universities by requiring students to choose a career pathway or major. Career and Technical Education (CTE), including agricultural education, focuses heavily on career exploration in order to help students better understand the skill, knowledge, and education expectations of specific careers (DeLuca, Plank, & Estacion, 2006). While no direct link has been established to connect successful secondary experiences in agricultural education all the way through the human capital pipeline to successful employment in STEM-based agriculture careers, studies have shown that “students’ course taking during high school plays a critical role in their ability to transition to postsecondary education and pursue a range of postsecondary majors and degree options” (Laird, Chen, Levesque, & Owings, 2006, p. 1). Dyer, Lacey, and Osborne (1996) found that while the percentage of University of Illinois College of Agriculture freshman students with secondary-level agricultural education was declining, the percentage of students intending to graduate with a major in agriculture was much higher among students with secondary agricultural education experience than among those with no previous agricultural education. Dyer, Breja, and Wittler (2002) found enrollment in a high school agricultural education program to be one of the most influential factors in whether students completed a degree in a college of agriculture. Enrollment in agriculture courses at the secondary level has also been found to correlate with more positive perceptions of agriculture (Smith, 2010). However, greater effort is needed to increase the number of high school agricultural education students who pursue higher-level agricultural careers through postsecondary education; one study found these students lacked understanding regarding the importance of postsecondary education for careers in agriculture (Smith, 2010). A growing body of literature supporting exposure to high-level careers in agriculture before college to increase the number of graduates skilled in agricultural sciences has led the National Research Council to recommend “colleges and universities…reach out to elementary-school and secondary-school students and teachers to expose students to agricultural topics and generate interest in agricultural careers” (2009, p. 9), specifically suggesting partnerships with secondary agricultural education programs.
The issues of recruitment and preparation for careers in agricultural sciences overlap. Thus the goal of this project is two-fold: 1) to create awareness and interest at the middle and high school levels for careers in the agricultural sciences, and 2) to prepare students for success in college, leading to a sustainable supply of well-educated agricultural scientists.
Within agricultural education there is little research examining effective teaching practices regarding the emphasis of STEM content naturally contained within the agriculture curriculum. Therefore, it is critical that researchers explore current teaching practices implemented by exemplary agriscience instructors. Furthermore, it is critical to explore innovative approaches for teaching STEM within agriculture curriculum in a variety of environments, including rural and urban schools. In combination, such efforts will inform a conceptual model of teaching STEM in agriculture which can be leveraged to support agriculture learning and career preparation among a wider population of students.
Although it is important for agricultural educators to be able to discuss the application of principles from all aspects of STEM, the science and math concepts in the context of agricultural education have garnered the most attention in the literature base because of their direct application to agriculture. Therefore, science and math will be the primary focus of this project.
Guidance on how STEM education – science education in particular – should be designed has been provided by the recent publication of A Framework for K-12 Science Education (NRC, 2012). In this report, it is recommended that science education be built around three major dimensions: practices, crosscutting concepts, and core ideas. Any model for secondary school agriscience programs that emphasize STEM content must take this framework and the Principles and Standards for School Mathematics (National Council of Teachers of Mathematics, 1998, 2000) into consideration.
The National Council of Teachers of Mathematics (NCTM) provides six principles as an outline to ensure school mathematics achieves the goals of developing students’ confidence, mathematical thinking and reasoning, and ability to communicate mathematically (NCTM, 2000). Students are expected to regularly explore interesting contexts and problems using mathematical concepts. It is through this exploration, connections with prior knowledge, and experience that students build meaningful connections to mathematical ideas and procedures in authentic situations.
Knowing the importance of preparing teachers to emphasize the science and mathematics of agriculture within a curriculum, it is important to understand what the practices entail. Crawford (2000) conducted a case-study of one high school biology teacher in the Pacific Northwest. This teacher had been noted for his outstanding use of inquiry-based learning when designing lesson plans. The aim of the research was to gain qualitative data that described what made this teacher’s lessons so effective. The results identified six characteristics of the teacher that allowed for successful use of inquiry-based instruction. Those six characteristics were: 1) situating the instruction in authentic problems, 2) grappling with data, 3) fostering collaboration between teachers and students, 4) connecting the students with their community through the lesson, 5) teacher modeling the behaviors of a good scientist, and 6) fostering student ownership in the project and the results.
Within agricultural education, Myers and Thompson (2009) conducted a study to determine what teachers needed in order to successfully emphasize STEM concepts in their classrooms. The responses of the National Agriscience Teacher Ambassador Academy participants were categorized as the following: 1) curriculum, 2) professional development, 3) teacher preparation programs, 4) philosophical shift, and 5) collaboration. Teachers in the study desired an agriculture curriculum written to be aligned with state and national standards for science and math along with a national database of lesson plans containing explicit emphasis of STEM concepts made available to teachers. Teachers also desired continuing instruction on how to highlight science and math principles found in the agriculture program. The teachers in the study believed pre-service teachers should be required to take coursework at their university to strengthen their knowledge of such a curriculum. The teachers also reported desiring a shift in philosophy regarding agricultural education. Teachers in the study believed transforming the view of agricultural education would help teachers of all disciplines understand the role agriculture can take in increasing student achievement. The teachers also valued collaboration, and believed team-teaching across disciplines would help to reinforce the importance of agricultural education and make agricultural educators a more valuable part of the education community (Myers & Thompson, 2009).
The profession needs to develop a model of effective practices emphasizing STEM concepts to prepare a future of agricultural scientists who are highly trained. If the profession is to develop a curriculum framework and further prepare teachers of agricultural education to explicitly highlight STEM principles in agriculture, the current teaching practices that are most effective for accomplishing this goal in secondary school agricultural education need to be identified.
The Concerns-Based Adoption Model, a research-based model, was designed to help facilitate change and provide diagnostic means of measuring implementation of an innovation (Hall & Hord, 2006) and provides a framework to guide this project. The model consists of the environment, the user system culture, resource system, change facilitator team, interventions, users and nonusers, and three diagnostic measures: stages of concern, levels of use, and innovation configurations (Hall & Hord, 2006). Hall and Hord (1987) define an intervention as “any action or event that influences the individuals involved or expected to be involved in the process” (p. 143). Interventions can range from training workshops to short conversations about the innovation called one-legged interviews (Hall & Hord, 2006).
Advantages of a Multi-state Effort
Advantages of the multi-state effort include an effective forum for building collaboration among agricultural scientists. The effort expands the ability to investigate the feasibility of differing curricula in rural and urban centers and across economic, demographic, and commodity focuses. Collaborative approaches are likely to formulate stronger solutions when compared to a single state or single researcher effort. Collaboration in a multi-state effort has and can continue to streamline and focus research in agriscience education. This project has enabled researchers from multiple institutions to meet regularly in an effort to pursue research activities that contribute to the project’s goal and objectives. A continuation of the project will enable researchers to continue to build these collaborative relationships, as additional experts have joined the project throughout its duration. In the future, this project will also allow for longitudinal data and replication of studies across the United States. Previous attempts at collaborative research have shown that without a multi-state effort, a proposed research focus can be difficult to manage and produces a greater burden on the faculty involved, thereby reducing their ability to enhance the project’s impact. The project is significant to the national agricultural education research agenda (Roberts, Harder & Brashears, 2016) that called for enhanced program delivery models and an abundance of highly qualified agricultural educators. The research agenda set forth by the USDA NIFA complements the National Research Agenda for the American Association for Agricultural Education (Roberts et al., 2016) by providing a priority area of creating the next generation of scientists.
Likely Impacts from Successful Completion
The successful completion of this research project will likely yield several impacts crucial to the continued success of agricultural education and the industries for which graduates are prepared to enter. Agricultural educators will have an increased awareness of the practices, cross-cutting concepts, and disciplinary core ideas included in the agriscience program. This increased awareness will be accompanied by modified curricula to guide secondary agriscience teachers in the highlighting of STEM concepts and ideas through articulated competencies. By providing teachers with education and guidance in highlighting STEM competencies in agriculture, the teachers will be more effective, agriscience programs will be of higher quality, and the components leading to high quality programs will be clarified for use across the nation. Finally, as students are exposed to STEM concepts in high quality agriscience programs led by prepared, effective teachers, their interest and engagement in agriculture-related STEM careers will increase. The end result contributes to an abundant supply of an educated workforce in agricultural careers that require scientific knowledge.
Related, Current and Previous Work
A search of the NIMSS systems revealed only one active multistate research coordinating committee and one advisory committee related to agricultural education. NCDC231: Collaborative for Research on Food, Energy, and Water Education focuses on research surrounding science literacy as developed in educational programs at the food, water, and energy nexus. Members of NCDC231 and the Agriculture Education Research advisory committee (NCAC-024) are included in the membership of this project. A previous research project, W-1006 Agricultural Literacy, focused specifically on evaluation of the Agriculture in the Classroom program and was terminated in 2013 and, to date, has not been renewed.
The integration of science into agricultural education has been a part of educational reform since the US industrial revolution. Managers, policymakers, and communities indicated they had a need for scientific advancement that was led by agriculture (Hillison, 1996). The Hatch Act of 1887 provided a mechanism to utilize federal dollars to fund scientific research to increase production. Later, the Smith-Hughes Act of 1917 brought those findings into the public school setting with the establishment of school-based agricultural education across the United States. School based agricultural education was formed from a need for vocational training for the rural males engaged in agricultural production. A hands-on curriculum created value for rural students to remain in school and receive an education while becoming established in farming. The high school agriculture instructor provided a way to move research into practice for local farmers, educating students and adult farmers on new innovations. Agricultural instructors helped the farmers understand the use of scientific discoveries as well as new farming practices with sound explanations based in the biological and physical sciences.
Due to changes in education and societal needs, the role of school-based agricultural education shifted to focus primarily on vocational training. Agricultural education lost grip of the authentic science investigations that drove its creation. From the 1950s through the late 1980s, agricultural education was well-focused on vocational training but strayed from the scientific concepts behind the hands-on skill set. During the early 1980s, a paramount publication by the National Commission on Excellence in Education titled A Nation At Risk (NCEE, 1983) laid the groundwork for the next two decades of focused science principles across all curricula. During the mid-1980s, agricultural education began to integrate science principles back into high school agricultural programs (Phipps, Osborne, Dyer, & Ball 2008).
Since the shift back to teaching the science of agriculture, research has been conducted both within and outside of agricultural education focused on the topic of integration across disciplines. Studies outside of agricultural education have reported that students who experience an integrated curriculum tend to have higher achievement and interest in learning than students who experience a curriculum focused only on one subject (Beane, 1995; Brago, Gragow, & Smith, 1995; Stevenson & Carr, 1993). One recent study has been conducted examining practical, student centered integration of math and science into the classroom across disciplines (Treacy & O’Donoghue, 2014). In agricultural education, research has been conducted that focuses on stakeholder perceptions of integration (Thompson, 1998). Some studies investigated scientific-focused curricula with differing teaching methods (Dyer, 1995; Flowers, 1986; Myers, 2004; Thoron, 2010), while few studies investigated math-focused agriculture curriculum (Stone, Alfeld, & Pearson, 2008). The need still remains for the development of a common definition and guiding model for integrating science and mathematics. Stakeholders have identified a deteriorating workforce lacking agricultural technical skills, scientific knowledge, and quantitative reasoning that places our nation’s national security and nation’s economy at risk (Steen, 2004; Thoron & Myers, 2008).
Significant trends in the US educational system prompt the need for a science-based investigative curriculum in agriculture (Thoron & Myers, 2008). Agriculturists, policy-makers, and community leaders agree there are societal benefits when agriscience education extends the knowledge of agriculture to urban and rural communities. With more diverse demographic characteristics and higher levels of participation, there is a growing challenge to provide and manage authentic agriscience investigations and experiences for school-based agricultural students. Demographic changes from rural to urban have affected student interest and career choice. This has led to an increased need for educating talented students who are well-equipped with agricultural knowledge that is based in science to meet the demands of scientific discovery in the agricultural industry.
Transforming Agricultural Education for a Changing World (2009), sponsored by the National Research Council, called for action to meet the need of professional education in agriculture of a diverse student body for the largest food producer in the world, the United States. Woven across this need for professional undergraduate programs that are attractive to, supportive of, and challenging for the students is the foundational need for career discovery at the high school and middle school levels. The simple understanding of how agriculture affects daily lives of all Americans must be expanded to include authentic science-based, research-driven curricula. The curricula must then focus on critical thinking, argumentation skills, and scientific reasoning of students through research-supported, issues-based, authentic assessments. This must occur while supporting the career and technical structure of the local program as students continue to focus on the applied portion of becoming successful in the workplace and in their career.
School-based agricultural education holds significant potential for helping to alleviate the shortage of agricultural scientists. A majority of high school agricultural programs are located in rural areas, providing the best opportunity to attract students who have some experience in agriculture. In addition, secondary agricultural education has a significant number of programs in suburban and urban schools, providing access to a large number of students who enjoy science and could be attracted to an agriscience field. Traditionally future scientists have not been actively pursued through these programs; with a new focus, these programs can provide value to industry beyond agricultural awareness. Secondary school agriscience programs exemplify a new biology approach in that integrated sciences are connected to agricultural problems and practices through a formal classroom and laboratory instructional program (NRC, 2009). One mission of the multi-state project will be to develop a curriculum framework and programs that set high ability students on an agriscience research track.
Specifically, the multistate project will focus on the areas of deepest concern in agriculture. For example, crop sciences and areas of food production, agronomy, and bio-research companies are faced with a severe shortage of highly qualified scientists (NRC, 2009). Leaders from Monsanto and ADM as well as crop science programs at universities experience serious concern when seeking candidates who can effectively connect their knowledge to field/growing conditions and aspects unique to agricultural industry, production practices, and producers. Decades have passed as this erosion of talent has increased and inaction has led to a lack of a productive pipeline for attracting and preparing future crop science researchers. The time for action is immediate and a call for a formal preparation strategy is needed (APLU, 2009).
An implication of the trends the crop science industry is facing is alarming and requires immediate, proactive efforts to significantly increase the number of high school students who enter college with an interest in crop sciences research. The continued shortage of researchers who can effectively connect their biology and chemistry competence to field conditions will slow the pace of innovation and discovery and place our food system at risk at a basic level (APLU, 2009). This leads to the importance for this multistate project to create a proactive, comprehensive recruitment and preparation strategy that begins at the high school level. High school remains the vital time when students make their initial career and college major decisions (Helwig, 2004). Action is needed now to maintain global leadership (Vilsack, 2009).
Rampant changes in the agriculture industry require a workforce with the ability to solve problems associated with scientific content. The United States Department of Agriculture recommended that students seeking future employment in the agriculture industry have “basic science skills and the ability to solve problems with scientific applications” (CSREES, 2005, p. 12). Agricultural education is in an ideal position to teach scientific content through an agricultural context (NRC, 2009; Washburn & Myers, 2010).
To provide students with valuable experiences that enhance their scientific content knowledge and problem solving skills, enhanced facilities, equipment, and materials will maximize student learning and emulate industry standards. The emergence of integrating scientific principles through agricultural education may provide opportunities for facilities to become a keystone in the teaching of scientific skills and problem solving (Shoulders & Myers, 2012a).
Experiential learning has shown to be an effective approach to teaching across multiple disciplines (Kolb, 2014; Kolb & Kolb, 2005). Furthermore, experiential learning in agricultural laboratories has been established as an ideal method to teach scientific content and problem solving skills to agriculture students in an effort to better prepare them for careers in the science-based agriculture industry (Myers & Washburn, 2008; NRC, 2009; Washburn & Myers, 2010, Shoulders, Blythe, & Myers, 2012). Providing students with experience in laboratory activities was the most important lesson-based attribute supported by previous research which found that teachers viewed their role during experiential learning as that of facilitator, responsible for guiding student learning experiences (Warner et al., 2006).
Facilities, equipment, and materials are important components of secondary agricultural education, and provide students with opportunities to develop problem-solving skills through experiential learning (Shoulders & Myers, 2012a). By designing agricultural education facilities to focus on scientific problem solving, teachers can enhance student experiences to prepare them more effectively for scientifically-based careers in agriculture (Parr & Edwards, 2004). Research to determine facilities and resources necessary to enhance the educational experiences will provide opportunities for teachers to improve student achievement in scientific problem solving through experiential learning.
Several self-reporting studies have provided a baseline of understanding of the importance and use of facilities and equipment. Research will help to identify barriers to utilizing facilities, equipment and resources, and help develop strategies to maximize student learning. Availability of a wide variety of agricultural laboratories was found to be common among secondary agricultural education programs. In a national study of agricultural educators (Shoulders & Myers, 2012b), respondents reported having access to a range of agricultural laboratories. Teachers also reported the frequency with which agricultural laboratories are utilized in secondary agricultural education. Over half of teachers with access to an aquaculture tank/pond (57.8%), biotechnology/science laboratory (63.0%), food science laboratory (50.0%), garden (61.1%), greenhouse (90.6%), livestock/equine facility (69.7%), meats laboratory (62.5%), mechanics/carpentry/welding facility (90.6%), nursery/orchard/grove (68.1%), small animal/veterinary laboratory (94.4%), or turf grass management area (54.5%) reported utilizing the laboratory at least once per week.
Results of First 5-Year Multistate Effort
The project focuses on three objectives in the effort to develop a model of effective practices, curriculum, and settings which can assist teachers in integrating STEM content within their agriscience programs. These three objectives are as follows:
- Identify practices, cross-cutting concepts, and disciplinary core ideas to be included in a secondary school agriscience program;
- Identify teaching methods, resources (facilities, equipment, materials, etc), and techniques currently utilized by exemplary teachers; and
- Develop an innovation configuration for implementing an agriscience program.
Objective 1: Identify practices, cross-cutting concepts, and disciplinary core ideas to be included in a secondary school agriscience program.
The National Research Council's (NRC) Framework for K-12 Science Education describes a vision of what it means to be proficient in science; it rests on a view of science as both a body of knowledge and an evidence-based, model- and theory-building enterprise that continually extends, refines, and revises knowledge. It presents three dimensions that will be combined to form each standard: Practices, Crosscutting Concepts, Disciplinary Core Ideas. Agriculture is a science, and the S1057 project seeks to strengthen the relationship between the science of agriculture and principles taught in science courses.
To address Objective 1 of the S1057 project, the following activities have been accomplished.
- A three-round survey, identifying the Disciplinary Core Ideas that should be included in each of the eight Agriculture, Food and Natural Resources (AFNR) Career Pathways, was conducted in 2014. Panelists included selected high school agriscience teachers, teacher educators in agriculture, and state agricultural education directors/supervisors.
- Panel participants proposed and then agreed on the disciplinary Core Ideas that should be included in a secondary school agriscience curriculum. Results of survey:
- Food products and processing systems = 23 core ideas
- Plant systems = 22 core ideas
- Animal systems = 19 core ideas
- Biotechnology systems = 14 core ideas
- Power, structural and technical systems = 20 core ideas
- Natural resources systems = 24 core ideas
- Environmental service systems = 20 core ideas
- Agribusiness systems = 19 core ideas
- An alignment with the Agriculture, Food and Natural Resources (AFNR) Career Pathways Performance Indicators was established by members of the AG-STEM Education Research Lab at the University of Florida.
- An alignment with Next Generation Science Standards (NGSS) Performance Expectations was established by members of the AG-STEM Education Research Lab at the University of Florida.
- A document was prepared that indicates the integration across the Disciplinary Core Ideas identified by the expert panel, the AFNR Performance Indicators, and the NGSS Performance Expectations.
- The report of the curriculum integration framework study can be found at:
http://aec.ifas.ufl.edu/docs/AGSTEM.pdf
- Journal articles and presentations based on the findings related to Objective 1 AG-STEM curriculum framework study:
- Barrick, R. K., Heinert, S. B., Myers, B. E., Thoron, A. C., & Stofer, K. (in review). Integrating disciplinary core ideas, agriculture, food and natural resources career pathways, and next generation science standards. Journal of Agricultural Education.
- Barrick, R. K., Myers, B. E., Heinert, S. B., & Thoron, A. C. (2015, November). Speaking their language: The science in agriculture. NAAE Annual Conference, New Orleans, LA.
- Barrick, R. K., Heinert, S, & Myers, B. (2015, May) Disciplinary core ideas in the agriculture, food and natural resources career pathways. Poster presentation. AAAE Conference, San Antonio, TX.
- Barrick, R. K., Heinert, S. B., & Myers, B. E. (2015, February). Disciplinary core ideas in the agriculture, forestry and natural resources career pathways. Southern Region AAAE Conference, Atlanta, GA.
- Wilcox, A. K., Shoulders, C. W., Myers, B. E., & Barrick, R. K. (2015, February). A description of CASE participants’ perceptions toward integration of science, technology, engineering, and math concepts. Poster presentation. Southern Region AAAE Conference, Atlanta, GA.
- Myers, B. E., Thoron, A. C., & Barrick, R. K. (2013, December). STEM in CDEs: Making a difference in your FFA chapter and classroom. NAAE Annual Conference, Las Vegas, NV.
- Barrick, R. K., Myers, B. E., & Thoron, A. C. (2013, June). Crossing disciplines: AG-STEM education research lab. NACTA Conference, Blacksburg, VA.
- The curriculum integration document will be used as the basis for Objectives 2 and 3 of the S1057 project.
Objective 2: Identify teaching methods, resources (facilities, materials, etc), and techniques currently utilized by exemplary teachers.
Team members carried out the methods outlined in the proposal. However, unanticipated results within the sampling led to delays in meeting the objective. Specifically, the three methods by which the team was to identify a comprehensive sample of exemplary teachers yielded great overlap, leading to a limited sample of teachers. Attempts to broaden the sample were met with the additional difficulty of identification; no set criteria exists for defining or identifying “exemplary” teachers. Several grant proposals were submitted in order to obtain funding to observe teachers, each denied due to the lack of a multi-state system for identifying teachers who are exemplary STEM integrators. The team determined that a pilot study that used observations of teachers identified as exemplary by the researchers would be an appropriate starting point, and is in the process of collecting data within classrooms currently.
Objective 3: Develop and innovation configuration for implementing an agriscience program.
The success of Objective 3 largely depended on the success of the first two objectives, as results would inform the development of the Innovation Configuration Map. Due to the delays occurring within Objective 2, development within Objective 3 was delayed. However, the team is currently in the process of developing the Innovation Configuration Map as prescribed by the project plan. The team has enlisted the services of a facilitator to guide the group through the Innovation Configuration Map development process. Thus far, the team, consisting of a subset of S1057 members, has met virtually to create initial themes and categories, collect relevant supporting literature and topic area tables were created. The team is currently working on developing draft narratives for each topic area. The draft Innovation Configuration Map is expected to be completed in December of 2016.
Objectives
-
1. Facilitate the adoption of an agriscience curriculum framework that is grounded in the concepts and approach of the Next Generation Science Standards.
Comments: a. Identify practices and cross-cutting concepts from the Next Generation Science Standards to be included in a secondary school agriscience program. b. Disseminate the curriculum framework to secondary agriculture teachers and teacher educators. -
2. Identify teaching methods, resources (facilities, equipment, materials, etc), and techniques currently utilized by exemplary teachers.
-
3. Develop an innovation configuration for implementing an agriscience program
Methods
Objective 1:
Previous work in Phase I relevant to Objective 1
A national panel was appointed to propose a set of disciplinary core ideas that could be included in the agriscience curriculum. The panels included representatives from the following groups:
- Agriscience teachers:
- participated in the National Agriscience Teacher Ambassadors Academy (n=18)
- nominated by experts in the field (have not completed the NATAA) (n=13)
- lead or master teachers for the 2013 cohort of Curriculum for Agricultural Science Education (n=48)
- Agriculture teacher educators (n=24), selected based on authorship of research articles and papers that addressed STEM education in agriculture
- State agriculture education staff (n=15), selected because of previous involvement with agriscience programming
A three-round modified Delphi Techniques process was utilized. Participants were asked to list disciplinary core ideas for the Agriculture, Forestry, and Natural Resources (AFNR) career pathway in the first round and then the subsequent two rounds served to narrow the list to those that were in widespread agreement. This research project resulted in a list of agricultural STEM disciplinary core ideas for each pathway, which were subsequently crosswalked with the National Council for Agricultural Education’s ANFR content standards and the Next Generation Science Standards (NGSS) performance expectations to produce a suggested Framework for Agricultural STEM Education (Heinert & Barrick, 2015).
Proposed Work
The work completed previously is an important first step, but significant effort remains to move toward a framework that is fully aligned with the NGSS and used by agriculture teachers, state supervisors, and teacher educators.
Expand and Refine the Framework for Agricultural STEM Education
Faculty from Virginia Tech, Michigan State U., U. Florida, and the U. Arkansas will provide leadership for this part of the project. These institutions have been involved in the project planning committee, have innovative relevant programs, and indicated interest in this phase of the work.
- National Delphi Panel of Agricultural Scientists
The Framework will be expanded to include crosscutting concepts and math concepts along with previously identified disciplinary core ideas. A national panel of 10 purposively selected experts in the agricultural sciences will be convened at a central site to participate in this phase of the study. The Delphi methodology will be utilized. Panelists will engage in dialogue regarding the lists of disciplinary core ideas that were previously generated. Additionally, they will develop cross-cutting concepts and identify math concepts that are important for agricultural science. During this session, panelists will discuss, refine, and agree upon the final set of items to be included in the agriscience curriculum. A timeline will be established, and efforts will be made to hold the panel in tandem with natural gathering events and locations, such as the NAAE Conference or National FFA Convention. If hosting the panel during a relevant simultaneous event is not feasible, funding will be sought to bring all panel members together to a university campus in a location central to where the panelists are located. In the absence of funding, the panel will be hosted electronically through a Blackboard Collaborate (or similar) virtual meeting room in which panel members can communicate, utilize a whiteboard, and share documents.
- National Panel of Science and Math Education Experts
A panel of 20 experts representing science teachers, math teachers, science and math teacher educators, and/or science and math state education agency consultants will be selected from nominations by the national science and math teacher organizations, universities, and state education agencies. Participants will verify that the science practices, cross-cutting concepts, and disciplinary core ideas established by agricultural STEM experts are consistent with the national standards for each. The panel will meet at a central location to conduct the verification of items task. Panel location selection will occur in a similar fashion to that in Part 1. Events for consideration will be the National Math and Science Partnership Conference and the National School Science and Mathematics Association Convention.
- Input from School Administrators
The final part of this effort will include local school administrators (e.g. principals, CTE directors, other administrators). 50 participants will be selected from schools where agricultural education programs exist and 50 participants will be selected from schools where they do not exist. A purposeful sample will be selected to ensure representation from schools in diverse settings (e.g., rural, urban, comprehensive public high schools, career and technical education centers, magnet schools, etc.). Participants will complete an electronic survey instrument regarding their opinion of the extent to which the cross-cutting concepts, disciplinary core ideas, and math concepts are and should be part of a total agriscience education instructional program and whether a STEM-based approach to agriculture would meet the needs of their students.
Objective 2:
Previous Work in Phase I Relevant to Objective 2
Faculty at Virginia Tech have worked with seven local communities in rural Virginia to develop contemporary curriculum modules for integration of agriculture innovations to build capacity for increasing the trained agriculture workforce across the Commonwealth, with an emphasis on the anticipated recommendations in the 2013 report, A Strategic Review of Agricultural
Education: Preparing Students for Successful Transition to College and Careers conducted for the Commonwealth of Virginia. This work emphasizes the integration of STEM concepts within the curriculum. To date lesson plans have been developed for new modules in each of the six primary areas of agricultural education curriculum. The modules emphasize the use of new technology and teaching practices to encourage student centered instruction in content that is relevant for the workforce needed in the various regions of Virginia.
Conference calls were offered to all teachers interested in completing a proposal to participate in the project to assist in project planning, goal setting, and proposal development. Three workshops were conducted at the Virginia Association of Agricultural Educators Summer Conference to allow three teachers in the program to teach other teachers the new lesson plans, demonstrate new technology used in the lessons, as well as demonstration of student centered teaching practices used to engage students in the new curriculum content. Two workshops were conducted at the National Association of Agricultural Educators Annual Conference to allow one of the teachers and a graduate student engaged in the project to teach other teachers the new lesson plans, demonstrate new technology used in the lessons, as well as demonstrate student centered teaching practices used to engage students in new curriculum content.
A two-day professional development program for early career agriculture teachers was conducted in December, 2015 and another is planned for January, 2017 to provide
participates an opportunity to engage with experienced teachers to discuss a variety of organized/facilitated topics to assist with the continued improvement in their teaching practices.
All project activities have been shared with the more than 300 secondary agricultural education teachers of Virginia via the statewide listserv as well as the state agricultural teachers’ association website. A representative of The National Council for Agricultural Education serves on the coalition and is therefore a liaison to the national leadership for agricultural education. An annual report of the project activities is sent to the Virginia Department of Agriculture and Consumer Services and a member of that department serves in the project coalition. A member of the Virginia Department of Education, Career and Technical Education staff team serves on the coalition and serves as a communication link to the Department.
Proposed Work
A convergent parallel mixed methods design will be employed to permit the research team the ability to mix the results from separate quantitative and qualitative components during the interpretation of the results. This will enable the team to confirm, cross-validate, or corroborate findings within the single study (Creswell & Plano Clark, 2011). Qualitative methods will include primary data from interviews, program site visits, and document analysis. Quantitative methods will include primary data from the academic records of agricultural science program concentrators and secondary data from local school student records and national data for CTE program concentrators and non-concentrators. For the purposes of this study, the two methods will be treated equally and independently from each other throughout the data collection and analysis process. Triangulation of data will take place during the interpretation of the findings.
The purpose of this design is to obtain different yet complementary data on the same topic to best understand the research problem presented above (Creswell & Plano Clark, 2011; Morse, 1991). Bringing together strengths and weaknesses of the quantitative and qualitative nature will enable the research team to compare and contrast the quantitative results with the qualitative findings to develop a clearer picture of exemplary teaching techniques, student career readiness, and knowledge acquisition for both populations (Creswell & Plano Clark, 2011). Using purposive sampling, the team will look to generate data for the quantitative strand using comparative and correlational statistics. Implementing both quantitative and qualitative methods will enable the research team to triangulate the data from multiple collection methods and obtain triangulated results about the topic for interpretation (Teddlie & Tashakkori, 2009).
The design features a series of seven data collection points: (a) semi-structured interviews with the participating teachers, (b) site/program visits of teachers using an observation protocol, (c) document analysis of program curriculum and associated materials, (d) longitudinal data released from partnering schools on program concentrators, (e) teacher survey of program concentrators, (f) national CTE student data from the National Center for Educational Statistics, and (g) follow-up telephone interviews with participating teachers to verify the themes developed during preliminary data analysis of the qualitative strand of the study.
Sample
The teacher population will include five teachers from each of five states for a total of 25 exemplary agriculture science teachers. Teachers identified as exemplary have been recognized by the National Association for Agricultural Education (NAAE) as “Teachers Turn the Key” award recipients. One teacher per state is selected annually for this recognition. Widely recognized by their peers as an exceptional early career teacher, recipients are selected and provided professional development activities to further their teaching acumen. The criteria used to identify the exceptional teachers through the NAAE Teachers Turn the key program include a professional teaching philosophy, examples of innovative instruction, examples of experiential learning in their teaching, Evidence of a strong Career and Technical Student Organization (CTSO), FFA for agricultural sciences, examples of partnerships in the school and community, evidence of program marketing efforts, and evidence of continuing professional growth in teaching. Additionally, we will include five science teachers who have been awarded the DuPont Pioneer Excellence in Agricultural Science Education Award from the National Science Teachers Association. This group of teachers was chosen because they are expected to be an “information rich” (Patton, 2002, p. 231) group with little comprehensive data of their teaching practices as a profession paired with the student populations they serve. Teachers will be asked to provide anonymous data for 25 recent graduates who are program concentrators for the study.
Data Analysis
Qualitative data analysis
The interview transcriptions will be completed using Windows Media Player© and Express Scribe© transcription software. In addition to the transcriptions, there will be a collection of documents examined within the study. The researchers will use the Atlas ti© software package to store, manage, code into categories, and aid in the analysis of the transcripts and related documents. Atlas ti software will be especially helpful since it has the capability of exporting coded data into SPSS (Gibbs, 2007).
Upon completion of the transcriptions, the researchers will review the data and began initial coding informed by the literature outlined in the propositions, the words of the participants, and the researcher’s interpretation of the investigation (Constas, 1992). The data analysis process will follow the constant comparative method in which “joint coding and analysis” (Glaser & Strauss, 1967/1995, p. 103) is conducted where “each incident” is “compared with other incidents for similarities and differences.…to identify properties and dimensions” (Corbin & Strauss, 2008, p. 73) that are specific to each category as they are developed. Document analysis will provide data on the context within which the research participants are operating. Information gleaned in the documents can help suggest questions that need to be asked during the interviews and focus the direction of the other aspects of data collection.
Quantitative data analysis
For research question four, descriptive methods will be used to summarize data, with the intention of identifying potential trends and patterns in order to summarize results for understanding in numerical form (Frankel, Wallen, and Hyun, 2012). Data will be analyzed using the Statistical Package for the Social Sciences (SPSS) version 24. Frequency tables, means, and correlations will be conducted in order to enhance the qualitative data collected and describe the student population from each state and as a population as a whole. Descriptive statistics will be used to compare mean scores between states and compare scores to the National Center for Educational Statistics data. Inferences will be used to establish relations between variables while providing reasonable certainty that relationships did not happen by chance.
Merging data
Following the collection and analysis of both quantitative and qualitative data, the research team will determine the strategies to be used to merge the data analysis. The results will be linked, combined, or integrated into meta-inferences that will help inform the research questions. While the two sets of analyses will be conducted separately, this will enable the research team to shape their analysis of the other by allowing the data to be examined together for convergent or divergent results (Teddlie and Tashakkori, 2009). According to Creswell and Clark (2013) there are three options for merging data analysis, the first is a side-by-side comparison where the quantitative and qualitative results are presented and discussed in a format that allows them to be compared to determine if one set of results confirms or disconfirms the other. The second option would be the use of a joint display that allows for direct comparison based on the development of a set of dimensions in which to compare the findings. For example, a table that presents the themes or categories of the qualitative findings along one axis and the themes or categories of the quantitative findings along the other. Finally, the third option is, “through data transformation merged analysis,” where the researchers would “transform one type of data into the other type so that both databases can be easily compared and further analyzed,” (p. 228). For example, with the use of the Atlas ti© software the qualitative codes can be converted to “numeric formation” and transferred for use is SPSS software based on the researchers’ determination of, “what aspect of the qualitative data to quantify and how to quantify it,” (p. 231).
The following states will be included in the completion of this component: FL, KY, SC, VA, WV
Objective 3:
The Concerns-Based Adoption Model presented by Hall and Hord (2006) contains three diagnostic instruments to measure implementation of an innovation. Each instrument addresses a different aspect of the change process. Innovation Configurations (IC) clarify what full implementation should look like. Levels of Use (LoU) chart individual's’ behaviors in regard to the change. Stages of Concerns (SoC) measure people’s feelings and perceptions of change. These three diagnostic instruments can be used separately or in combination with others to assess the status and success of implementation of an innovation (Hall & Hord, 2006).
Innovation Configurations (IC) describe what the change should look like when it is properly implemented (Hall & Hord, 2006). An Innovation Configuration map will be created, based upon the findings of Objective 1 and 2, to develop a common understanding of what is expected and to measure how an innovation has been implemented. Hall and Hord (2006) note, “the innovation in action can take on many different operational forms or configuration;” in addition, “the tendency to adapt, modify, and/or mutate aspects of innovations is a natural part of the change process” (p. 113).
The development of Innovation Configuration maps is a very time consuming and laborious process. It will require the development and articulation of a clear description of the appearance of the desired implementation as well as various differing configurations which may occur. Developers will decide what the most desirable implementation is and how much fidelity to that conception is required for implementation to be satisfactory. Throughout the process all parties will be able to view and contribute to the Innovation Configuration map. However, an Innovation Configuration map requires all parties to decide on a consensus and operationally define an innovation. Once the Innovation Configuration map is completed, it will provide a valuable tool in identifying what components are being implemented well and which components need additional work. The Innovation Configuration map makes implementation more effective and efficient. It also documents the extent and quality of implementation for evaluation studies.
A draft of an Innovation Configuration map was developed in 2016 by a team of researchers to help reflect upon the recommended practices to better clarify and define high quality agriscience programs. The draft map will provide a starting point for further review to come to consensus and operationally define implementation of a high quality agriscience program. Once the draft Innovation Configuration map has been reviewed and prepared as a final document, the map can be released for adoption by state agriscience programs. As states begin to adopt and utilize the map, agriscience programs can begin to be evaluated and further research can be conducted to guide the overall framework for secondary agriscience programs that emphasize STEM content in agriculture. In addition to providing an implementation and evaluation tool for high quality agriscience programs, an overarching, long term goal of the review and implementation of the Innovation Configuration map will be to increase student engagement in STEM careers.
The following states will be included in the completion of this component: FL, SC, AR, OK, PA, WV, KS, MI
Measurement of Progress and Results
Outputs
- Validated practices, cross-cutting concepts, and disciplinary core ideas (Obj. 1)
- Public web site that includes related materials and results (Obj. 1, 2, & 3)
- Publication of Modified Delphi and Delphi results (Obj. 1)
- Series of reports reflecting recommended practices resulting from project’s efforts (Obj. 1, 2, & 3)
- Workshops, webinars, e-learning materials and other delivery methods to disseminate findings to teachers and teacher educators (Obj. 1 & 2)
- List of teaching methods, resources, and techniques currently utilized by exemplary teachers (Obj. 2)
- Publication of innovation configuration for implementing an agriscience program (Obj. 3)
Outcomes or Projected Impacts
- Increased awareness of the practices, cross-cutting concepts, and disciplinary core ideas included in the agriscience program (Obj. 1)
- Modified secondary school curricula that include agriscience competencies (Obj. 1)
- Increased teacher effectiveness and higher quality agriscience programs (Obj. 2)
- Clarification within the agricultural education profession of what constitutes high quality agriscience programs (Obj. 3)
- Long term – Increased student engagement in STEM careers (Obj. 3)
Milestones
(2018):● Appointment of four national panels to participate in the modified Delphi study of potential practices, cross-cutting concepts, and disciplinary core ideas (Obj. 1). ● Identification of exemplary secondary school agriscience teachers (Obj. 2). ● Establish partnerships with the school districts of the exemplary teachers willing to participate in the study (Obj. 2). ● Develop and begin implementation of a plan to collect agricultural science student data at each participating school (Obj. 2)(2019):● National panel of experts appointed and convened to prepare final list of practices, cross-cutting concepts, and disciplinary core ideas; Verification of math and science competencies by content experts (Obj. 1). ● Make classroom visits and observations (Obj. 2).
(2020):● Continue classroom visits and observations (Obj. 2). ● Needs assessment instrument administered to agriscience teachers (Obj. 1). ● Workshops conducted to disseminate proposed practices, cross-cutting concepts, and disciplinary core ideas (Obj. 1). ● Analyze data and identify teaching methods, resources, and techniques utilized by exemplary teachers (Obj. 2). ● National study of local school educators regarding the extent to which the competencies are and should be part of the agriscience curriculum model (Obj. 1).
(2021):● Additional workshops conducted to disseminate proposed practices, cross-cutting concepts, and disciplinary core ideas (Obj. 1 & 2). ● Draft innovation configuration for implementing agriscience programs (Obj. 3).
(2022):● Release innovation configuration for adoption by state agriscience programs (Obj. 3).
Projected Participation
View Appendix E: ParticipationOutreach Plan
Identify In-service Needs of Agricultural Education Instructors
Upon completion of project components, an electronic, survey-based study will be conducted of agricultural education instructors nationally. A random sample of 600 instructors will be asked to participate. The survey will be based on components of the final Innovation Configuration. Participants will be asked to indicate the importance of, their knowledge of, and their ability to teach each of the practices, cross-cutting concepts, and disciplinary core ideas in the Framework. Using the Borich Model for conducting a needs assessment, items will be rated by the instructors to determine areas that teachers indicate a need for in-service education. The Dillman Tailored Design Method will be used to ensure an acceptable response rate, typically after six contacts. Non-response follow-up will be conducted to reduce error attributed to non-response.
Faculty from U. Florida, the U. Arkansas, and Virginia Tech will provide leadership for this survey. These institutions have been involved in project planning, have innovative programs related to this effort, and have indicated an interest in this phase of the work.
Develop Train-the-Trainer Program
To facilitate widespread adoption of the new Framework and Innovation Configuration, funding will be sought to develop a train-the-trainer program for agriculture teacher educators and state staff. A 1-day workshop with follow-up webinars will be planned and implemented to introduce the framework to state leaders and support them in planning for disseminating it to agriscience teachers in their state.
The project’s results will be disseminated through the project’s website, presentations at research conferences (i.e. American Association of Agricultural Education), publications in research journals (i.e. Journal of Agricultural Education) and practitioner journals, the development of technical reports, and the delivery of workshops for both researchers and practitioners.
Organization/Governance
An executive committee consisting of a chair, vice-chair, and three (3) other project members will be established to maintain the direction of this project. The executive committee will meet quarterly (either face-to-face or via conference call) to assess progress. Further, an annual meeting of all participants will be held
Literature Cited
APLU. (2009). Human Capacity Development The Road to Global Competitiveness and Leadership in Food, Agriculture, Natural Resources, and Related Sciences (FANRRS).Washington, DC. Retrieved from http://www.aplu.org/NetCommunity/Document.Doc?id=1639.
Barrick, K., Heinert, S., & Myers, B. E. (2015, January). Disciplinary core ideas in the agriculture, forestry and natural resources career pathway. Paper presented at the Southern Region meeting of the American Association for Agricultural Education, Atlanta, GA.
Carnevale, A. P., Smith, N., & Melton, M. (2011). STEM. Georgetown University, Center on Education and the Workforce. Retrieved from cew.georgetown.edu/stem/. January 9, 2012.
Crawford, B. A. (2000). Embracing the essence of inquiry: New roles for science teachers. Journal of Research in Science Teaching, 37(9), 916-937.
Corbin, J. & Strauss, A. (2008). Basics of qualitative research: Techniques and procedures for
developing grounded theory. Third Edition. Sage Publications, Inc.: Thousand Oaks,
CA.
CSREES. (2005). Employment Opportunities for College Graduates in the U.S. Food, Agricultural, and Natural Resources System. USDA, Cooperative State Research, Education, and Extension Service. Retrieved from http://www. faeis.usda.gov.
DeLuca, S., Plank, S., & Estacion, A. (2006). Does career and technical education affect college enrollment? Columbus, OH: National Dissemination Center for Career and Technical Education.
Doerfert, D. L. (Ed.) (2011). National research agenda: American Association for Agricultural Education’s research priorities for 2011-2015. Lubbock, TX: Texas Tech University, Department of Agricultural Education and Communication.
Dyer, J. E. (1995). Effects of teaching approach on achievement, retention, and problem solving ability of Illinois agricultural education students with varying learning styles. Unpublished doctoral dissertation, University of Illinois at Urbana-Champaign.
Dyer, J. E., Breja, L. M., & Wittler, P. S. (2002). Predictors of student retention in colleges of agriculture. Paper presented at the 27th Annual National Agricultural Education Research Conference, p. 490-501.
Dyer, J. E., Lacey, R., & Osborne, E. W. (1996). Attitudes of University of Illinois College of Agriculture freshmen toward agriculture. Journal of Agricultural Education, 37(3), 33-42.
Enderlin, K. J., & Osborne, E. W. (1992). Student achievement, attitudes, and thinking skill attainment in an integrated science/agriculture course. Paper presented at the 19th Annual National Agricultural Education Research Meeting, St. Louis, MO.
Flowers, J. L. (1986). Effects of problem solving approach on achievement, retention, and attitudes of vocational agriculture students in Illinois. Unpublished doctoral dissertation, University of Illinois at Urbana-Champaign.
Hall, G. E., & Hord, S. M. (2006). Implementing change: Patterns, principles, and potholes (2nd ed.). New York: Pearson Education.
Hall, G. E., & Hord, S. M. (1987). Change in schools. Albany, NY: SUNY Press.
Helwig, A. A. (2004). A ten-year longitudinal study of the career development of students: Summary findings. Journal of Counseling & Development, 82(1), 49-57. Retrieved from http://aca.metapress.com/media/524c86ttyk1ynvlxgndm/contributions/n/k/5/2.
Heinert, S., & Barrick, K. (2015). A framework for agricultural STEM education. Retrieved from http://aec.ifas.ufl.edu/media/aecifasufledu/Framework_for_AgSTEM_Education.pdf
Hillison, J. (1996). The origins of agriscience: Or where did all that scientific agriculture come from? Journal of Agricultural Education, 37(4), 8-13.
Laird, J., Chen, X., Levesque, K., & Owings, J. (2006). The postsecondary educational experiences of high school career and technical education concentrators. US Department of Education, Report No. NCES 2006-309rev.
Myers, B. E. (2004). Effects of investigative laboratory integration on student content knowledge and science process skill achievement across learning styles. Unpublished doctoral dissertation, University of Florida.
Myers, B. E., Thoron, A. C., & Thompson, G. W. (2009). Perceptions of the National Agriscience Teacher Ambassador Academy toward integrating science into school–based agricultural education curriculum. Journal of Agricultural Education, 50(4), 120-133.
Myers, B. E., & Washburn, S.G. (2008). Integrating science in the agriculture curriculum: Agriculture teachers’ perceptions of the opportunities, barriers, and impact on student enrollment. Journal of Agricultural Education, 49(2), 27-37.
National Center for Excellence in Education. (1983). A Nation At Risk. (NCEE Publication No. 065-000-00177-2). Gardner, DP: Author. Washington, DC.
National Council of Teachers of Mathematics. (1989). Curriculum and evaluation standards for school mathematics. Reston, Va.: The Council.
National Council of Teachers of Mathematics (2000). Principles and Standards for School Mathematics. Reston, Va.: The National Council of Teachers of Mathematics, Inc.
National Governors Association Center for Best Practices, Council of Chief State School Officers. (2010). Common Core State Standards. National Governors Association Center for Best Practices, Council of Chief State School Officers: Washington, DC.
NIFA. (2010). Employment Opportunities for College Graduates in the U.S. Food, Agricultural, and Natural Resources System. USDA, National Institute of Food and Agriculture. Retrieved from http://www. ag.purdue.edu/usda/employment.
National Research Council. (2009). Transforming Agricultural Education for a Changing World. The National Academies Press, Washington, D.C.
National Research Council. (2012). A framework for K-12 science education: Practices, Crosscutting Concepts, and Core Ideas. Committee on a Conceptual Framework for New K-12 Science Education Standards. Board on Science Education, Division of Behavioral and Social Sciences and Education, Washington, DC: The National Academics Press.
Parr, B. & Edwards, M. C. (2004). Inquiry-based instruction in secondary agricultural education: problem-solving – an old friend revisited. Journal of Agricultural Education, 45(4), 106-116.
Phipps, L. J., Osborne, E. W., Dyer, J. E., & Ball A. L. (2008). Handbook on Agricultural Education in Public Schools. (7th ed.). Clifton Park, NY: Thomson Delmar.
Shoulders, C. W., Blythe, J. M., & Myers, B. E. (2012). Teachers’ perceptions regarding experiential learning attributes in agricultural laboratories. Paper presented at the Association for Agricultural Education Meeting, Asheville, NC.
Shoulders, C. W., & Myers, B. E. (2012a). Teachers’ use of experiential learning stages in agricultural laboratories. Paper presented at the Southern Region Association for Agricultural Education Meeting, Birmingham, AL.
Shoulders, C. W., & Myers, B. E. (2012b). Teachers’ use of agricultural laboratories in secondary agricultural education. Journal of Agricultural Education, 53(2).
Smith, E. (2010). High school students’ perceptions of agriculture and agricultural careers as delineated by presence of an agriculture program and rural/urban categorization. Unpublished undergraduate honors thesis, Cornell University.
Steen, L. A. (2004). Achieving quantitative literacy: An urgent challenge for higher education. Washington, D.C.: The Mathematical Association of America.
Stone, J. R., Alfeld, C., & Pearson, D. (2008). Rigor and relevance: Enhancing high school students' math skills through career and technical education. American Educational Research Association, 45(3), 767-795.
Thompson, G. (1998). Implications of integrating science in secondary agricultural programs. Journal of Agricultural Education, 39(4), 76-85.
Thoron, A. C. (2010). Effects of inquiry-based agriscience instruction on student argumentation skills, scientific reasoning, and student achievement. Unpublished doctoral dissertation, University of Florida.
Thoron, A. C., & Myers, B. E. (2008). Agriscience: Sustaining the future of our profession. The Agricultural Education Magazine, 80(4), 9-11.
Vilsack, T. J. (2009). Letter to USDA Employees. February 12, 2009. As cited in Human Capacity Development The Road to Global Competitiveness and Leadership in Food, Agriculture, Natural Resources, and Related Sciences (FANRRS).Washington, DC. Retrieved from http://www.aplu.org/NetCommunity/Document.Doc?id=1639.
Warner, W., Arnold, S., Jones, D. W., & Myers, B. E. (2006, February). The use and design of laboratory instruction in secondary agriscience classrooms. Presented at the Southern Region Meeting of the American Association for Agricultural Education, Orlando, FL.
Washburn, S. G., & Myers, B. E. (2010). Agriculture teacher perceptions of preparation to integrate science and their current use of inquiry based learning. Journal of Agricultural Education, 51(1), 88-98.