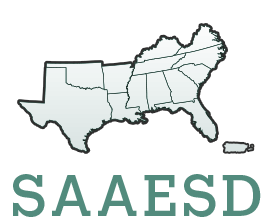
S1032: Improving the Sustainability of Livestock and Poultry Production in the United States (OLD S1032)
(Multistate Research Project)
Status: Inactive/Terminating
S1032: Improving the Sustainability of Livestock and Poultry Production in the United States (OLD S1032)
Duration: 10/01/2007 to 09/30/2013
Administrative Advisor(s):
NIFA Reps:
Non-Technical Summary
Statement of Issues and Justification
The Need for a Systems Orientation. The U. S. government and state governments in the major livestock- and poultry-producing regions of the U. S. have committed significant resources over the past 10+ years to the development, evaluation and adoption of best management practices (BMPs), advanced technologies and other science-based tools to reduce or prevent environmental pollution from concentrated animal production systems. Many of those tools have been validated at the laboratory, pilot and/or field scales in tightly controlled experiments, but their overall, dynamic impact at the ecological scale is not well understood. Even so, these technologies and practices, as well as policies and incentive structures conceived around them, are being routinely recommended and adopted.
Without a comprehensive, general understanding of the systems interactions that govern the overall effectiveness of a technology, a practice, an incentive structure or a policy, the American livestock and poultry industries and state and federal governments will continue to expend huge sums of public and private revenue on implementation of those tools without reasonable expectation of a particular cost/benefit threshold for any of them. It is one thing, for example, to show that a particular tactic is capable of reducing nitrogen requirements in feed without sacrificing mean animal performance (e. g., milk production, lean meat deposition). It is another thing to show that a suite of nitrogen-reduction tactics implemented together on a model farm will reduce net ammonia emissions to the atmosphere. But it is quite another thing entirely to illustrate that the overall impact of a proposed strategy on North American ecosystems (including energy and water resources, wildlife, water and air quality, human health and economic sustainability) will be a net positive and even more elusive is knowledge of what the true cost of that benefit is likely to be on the economic, political and social structures in which AFOs operate and on which they are dynamically interdependent.1
Figure 1 (adapted from Sweeten, 1999) provides a useful context for these systems considerations. This diagram is a model of the environmentally significant stocks, flows and transformations of matter in the North American beef-production system. Of course, any one of the elements in the diagram may be encircled and called an open system by itself, and that is indeed what has been happening over the past several decades as we have developed individual technologies and refined existing processes to increase the efficiency of those individual elements. It is becoming clear, however, that increasing efficiency at the process level does not necessarily reduce ecological stress overall.2 Because real ecological systems are characterized by feedback, human choice and other nonlinearities, changes in one element of the system may propagate through the entire system in an unpredictable or even counterintuitive way.
Figure 1. Schematic diagram of an environmental quality model of beef production in the United States, adapted from Sweeten (1999).SEE ATTACHMENT FOR SCHEMATIC GRAPH DIAGRAM
Another critical characteristic of ecological systems is that they provide very real, very important services that sustain life on the planet. The fact that these services do not have a cash value complicates the accounting and makes their inclusion in economic analyses difficult. But because of the complex nonlinear interactions described above as well as the global nature of todays human economic activity, including agriculture and the energy that supports it, the true value of ecological services must be included in future analyses. Researchers have developed several analytical tools and disciplinary paradigms to account for these services and the impact of human activity, including ecological footprint analysis (EFA), embedded energy (emergy) accounting, exergy, life-cycle analysis (LCA), ecological efficiency and industrial ecology.
FOOTNOTE:(1) To illustrate, what if the technologies are far more energy intensive than the status quo? Does an air-quality benefit in one livestock-intensive airshed simply export air pollution to another airshed in the form of increased demand for electrical power? How might widespread adoption of process-level tactics affect, and be affected by, market distortions resulting dynamically from those tactics or from externalities?
FOOTNOTE:(2) The accelerating depletion of the Ogallala Aquifer, which results in large measure from improved irrigation efficiency, is an important illustration of the sometimes perverse effect of technological advance (Marek, 2005; Allen, 2006). In that case, improved irrigation technologies are bringing dryland acreage back into irrigated production, increasing irrigated acreage and net aquifer withdrawals. This phenomenon is sometimes referred to as the "rebound effect".
Related, Current and Previous Work
The central motivation for EFA, emergy accounting and other analytical frameworks is that when we focus our attention only on open systems (systems that exchange matter and energy with their surroundings), we implicitly assume that the sources of matter and energy flowing into the system are infinite or, more damning, we choose to ignore the reality that those sources are finite. Wackernagel and Rees (1996) framed the issue in geopolitical terms by drawing system boundaries coincident with national boundaries, then demonstrating that when the EF of a highly advanced, energy-intensive nation exceeds its ecologically productive land and sea area, that nation necessarily appropriates ecologically productive areas from other nations via commerce. Because the earths ecologically productive resources are finite, trading for other nations surplus ecological resources artificially perpetuates the illusion that a highly sophisticated, energy-intensive society is sustainable merely through further technological advancement and free trade. Technology and free trade may, in fact, be central to ensuring sustainability, but they may not be sufficient to do so by themselves as the global economy nears its biophysical limits of energy available to do work.
In a similar vein, Manning (2004) chose to draw the system boundary around the earth and the sun, recognizing that all of the significant energy reserves potentially available on the earth derive from a finite combination of (a) net current solar energy flux, (b) net solar energy sequestered in the earth since its formation and (c) the initial energy (kinetic, enthalpic, chemical and nuclear) sequestered in the earth at its formation. So-called renewable energy sources, like solar, wind, tidal, biomass and hydroelectric power, derive principally from the interaction of global circulation and the net current solar energy flux, which drives climate, short-term weather phenomena and the hydrologic cycle. So-called non-renewable energy sources (e. g., fossil fuels and fissile materials) derive principally from historical solar energy fluxes and the energy originally stored in the earth. Ultimately, however, those sources are limited, and harvesting them requires ever-increasing expenditures of the available energy already at our disposal (Hubbert, 1949). Underscoring the point, Hubbert (1982) responded to a colleague with these observations:
If oil had the price of pharmaceuticals and could be sold in unlimited quantity, we probably would get it all out except the smell. However there is a different and more fundamental cost that is independent of the monetary price. That is the energy cost of exploration and production. So long as oil is used as a source of energy, when the energy cost of recovering a barrel of oil becomes greater than the energy content of the oil, production will cease no matter what the monetary price may be. During the last decade we have very large increases in the monetary price of oil. This has stimulated an accelerated program of exploratory drilling and a slightly increased rate of discovery, but the discoveries per foot of exploratory drilling have continuously declined from an initial rate of about 200 barrels per foot to a present rate of only 8 barrels per foot.
In summary, then, the long-term sustainability of agriculture will ultimately be governed by the sustainability of solar energy to the planet. If sequestered energy reserves (e. g., nuclear and fossil fuels) accessible to mankind exceed the balance of energy obtainable from the sun, we must simply make our sequestered reserves last as long as possible, preferably until the sun itself expires. But if the suns remaining energy exceeds our sequestered energy reserves, long-term sustainability demands that (a) we pursue a course in which our total energy use is less than that we can practically harvest from the sun or (b) we content ourselves with the prospect of a declining quality of life (or an equivalent decline in population for a given quality of life) as our sequestered sources are depleted. Solution (b) is achievable by default, by simply continuing on our present trajectory of total energy consumption. Solution (a), by contrast, requires a coherent, scientific framework for estimating, and then reducing if necessary, the net consumption of those ecological resources that are ultimately powered by the sun.
We propose to develop computer based mathematical descriptions of the animal production industries using measures of sustainability and environmental impacts that will help us describe and define that scientific framework. Although all aspects of animal production must be included, we propose to put special emphasis on evaluating manure management and utilization best management practices and their impact on sustainability and environmental impacts beyond the farm and field scale.
A number of interesting and useful analytical paradigms already exist for describing and modeling the sustainability of arbitrarily defined systems, and we do not intend to suggest that one of them is necessarily superior to the others in every conceivable use or context. Each of them has strengths and shortcomings that depend on the way in which it is used. For example, EFA is strongly intuitive and visual, lending itself well to technology transfer to non-technical or even non-specialist audiences. Emergy accounting, in contrast, is rooted in 2nd-law thermodynamics considerations (i. e., not all forms of energy have the same ability to do work) but is not easily translated into useful public language. Because EFA and emergy accounting can be configured with solar energy as their common referent,(3)however, it is conceivable that the scientifically rigorous analysis of animal-feeding systems could be conducted in emergy terms and then translated into eco-footprint language for public consumption. Similar observations could be made concerning LCA, eco-efficiency and other analytical paradigms. Consequently, we have framed our methods using the lexicon of EFA as shorthand for what we intend to be a fuller, more rigorous, multifaceted approach using all of the tools these emerging disciplines have to offer.
Ecological Footprint Analysis of AFO Systems. The concept of ecological footprint analysis (EFA), as applied to human populations, has been defined succinctly by Rees (1997) as estimating the total area of productive land and water required to produce on a continuous basis all the resources consumed and to assimilate all the wastes produced by [a] population, wherever on Earth the land may be located. Among the key principles underlying EFA are that (a) all stocks of material resources, including water, air, nutrients and energy, are finite; (b) where a local deficiency in any of those stocks is overcome by commerce, the transaction merely displaces the ecological stress associated with harvesting and using that stock but does not eliminate it; and (c) humans are integral rather than external to the ecosystems in which they operate. EFA purports to be a means by which to monitor progress toward sustainability; to compare the ecological impacts of cities, life-styles, or technologies; or to weigh aggregate human demand against available supply (Rees, 1997). It is precisely that suite of promises that the committee intends to explore and (if possible) exploit in the context of concentrated animal production.
Rees approach to EFA, described and exemplified in Rees (1997), essentially reduces all of the ecological costs being considered to a single unit of currency: (ecologically productive) land area per capita. If sustainability is viewed primarily in terms of long-term, global, net energy flow, a single land-area currency may be fully satisfactory. But we must also grapple with nearer-term sustainability issues at the regional and national level that can help us describe the contours of a transition from todays livestock production systems to tomorrows systems. To accomplish that, we will need to consider other ecological values (e. g., water and air quality, ground water quantity) that shape public discourse and policy. In regional application, the ecological footprint concept may be multi-dimensional.
Chen et al. (2006) analyzed Chinese agriculture using the emergy tools and techniques of Odom (1996). In spite of a long history of low input and localized production, this analysis showed that Chinese agriculture is beginning to look like agriculture in the developed world in terms of increased inputs, higher energy requirements, intensive economic investment, and reduced sustainability (Chen et al., 2006). However, while such an analysis is useful a broad picture of a large system, it alone does not suggest ways to practically and economically change production practices to improve sustainability.
Heller & Keoleian (2003) used the life cycle approach to assess the sustainability of the entire U.S. food system. This was an initial effort and broad in scope but showed there are benefits to a holistic approach including linking food consumption to production in the publics mind, identifying social issues that impact sustainability such as labor and food wastage, and identifying overlooked parts of the system such as energy use in the home as it relates to food consumption.
The aim in this 5-year research effort is to begin applying EFA (or other analytical paradigm) in a detailed fashion to the livestock sectors, extending its scope to include considerations of water, energy and nutrient stocks and flows as well as air and water quality. This system-oriented approach will not require abandoning process-oriented, reductionist research. Rather, we will attempt to synthesize it with, and integrate it into, broader considerations of the ecosphere and the development of new ecological currencies that could be used to mediate allocation of ecological goods and services within the livestock sector of the regional economy.
A comprehensive approach to ecological footprint modeling of concentrated livestock production would require, at a minimum:
1. Procedures for estimating the stress imposed by livestock production on the following, dynamic components of an ecosystem:
a. Quality of environmental media (air, surface water, ground water, soil)
b. Natural resources (populations, reservoirs and flows)
i. Recoverable, conserved resources (e. g., energy, water, nutrients)
ii. Non-conserved resources (e. g., species)
c. Climate
d. Markets for livestock products and by-products
e. Quality of life; and
2. A rational means of normalizing and integrating those model components to express the net change in cumulative ecological stress that would be expected as a result of implementing policy, technology or other measures in the concentrated livestock sector.
A CRIS search revealed five regional projects closely related to the proposed replacement project: WERA-103, Nutrient Management and Water Quality, whose objectives are more related to optimizing and minimizing crop nutrient application; S-1025 Systems for controlling air pollutant emissions and indoor environments of poultry, swine, and dairy facilities; SDC-321, Environmental issues affecting poultry production; NE-132, Environmental and Economic Impacts of Nutrient Management on Dairy Forage Systems, whose objectives are to study dairy forage systems primarily in the northern states; and NC-119, Management systems to improve the economic and environmental sustainability of dairy enterprises. These projects are largely focused either on specific regions, species, or environmental media and therefore would be largely complementary and do not represent duplication to the proposed project. One of the strengths of the terminating project, S-1000, for which this proposed project is a replacement is that it has established a track record of a multi-disciplinary, integrated project.
FOOTNOTE:(3) Indeed, it is tempting to suggest that because a finite area of ecologically productive land and ocean is capable of harvesting a finite proportion of the earths incoming solar radiation, there is a conceptual nexus at which, properly defined and comprehensively rendered, EFA and emergy accounting are but alternative expressions of the same fundamental ideas. We have not proved that conjecture, obviously, but it is a provocative, potentially satisfying property to be explored in our proposed work.
Objectives
-
Develop preliminary models of each animal industry that describe its cumulative ecological risk, energy flows or ecological footprint as a dynamic, nonlinear function of the stocks, flows and transformations of matter and energy comprising CAFO systems.
-
Continue the development and performance evaluation of process-level strategies and tactics to reduce environmental pollution at the process level from confined animal feeding operations. This work will include 1) management tools, strategies and systems for land application of animal manures and effluents that optimize efficient, environmentally friendly utilization of nutrients and are compatible with sustained land and water quality; 2) physical, chemical and biological treatment processes in engineered and natural systems for management of manures and other wastes; 3) methodology, technology, and management practices to reduce odors, gases, airborne microflora, particulate matter, and other airborne emissions from animal production systems; and 4) feeding systems for their potential to alter the excretion of environmentally-sensitive nutrients by livestock.
Methods
Objective 1. Develop preliminary models of each animal industry that describe its cumulative ecological risk, or ecological footprint, as a dynamic, nonlinear function of the stocks, flows and transformations of matter and energy comprising CAFO systems. This objective represents a significant change in how the project has functioned in the past. As such, we view it as a developing objective; one that will grow in its participation once project participants are more vested in the process and have a better understanding of how the data they collect and the interim conclusions they reach under Objective 2 contribute to (and are recursively refined by) this objective. Task A. Recruit participation in this group from a broader scope of scientists and disciplines. In order to truly evaluate the total ecological impact of discipline work, we must extend our group of participants to represent a broader array of disciplines. The most recent project consisted of largely agricultural engineers, animal scientists, and agronomists. Under this proposed new project, success will be dependent on participation from social scientists (economists, modelers) as well as a broader scope of biological scientists (ecologists) and enhanced participation from soil and water scientists. All project participants will be asked to help in this recruitment process, which has already begun and will continue throughout the term of the new project. In particular, the following states have indicated that this will be one of their project foci: MI, MN, NC, CA, TX, IN, MD, GA. Recruitment will be directed towards both USDA-ARS and university scientists, alike, to include universities and research foundations that have not traditionally partnered with our sector of the land-grant university system. Task B. Develop a conceptual framework for a model that describes each animal industry at the national scale and its ecological footprint, emergy ledger or life-cycle profile. The purpose of the model is to discern and direct the true impacts of our research and our national policy. Sub-Task i. Identify information needs to contribute to the conceptual model, including data, stocks, flows, and indices. Information needs are dictated by the conceptual/mathematical models that are developed. At a minimum, these models must describe and account for nutrient flows from the crop systems (feed input) all the way through the livestock systems and out again. Researchers in CA will lead the effort to ensure that developed models are appropriate for the intended purpose and are including necessary components. Researchers in MN and NC will assist in identifying these needs. As discipline-oriented research is conducted, data will be contributed to the team of researchers that are working under this objective so that the type of data needed (units of input, and specified inputs) can be clearly defined. Researchers in MD will contribute mainly by supplying data related to energy costs and interactions of management changes for the broiler chicken industry. These management options will include diet changes and their impact on litter quality and moisture, the impact of litter moisture changes on emissions and costs of changing litter moistures (cost/benefits), and assessment of the management options impacts on overall energy costs, productivity and emissions of changes in animal density. Researchers in MI will provide parallel information for the swine industry and contribute data for the laying hen and cattle industries. Researchers in NC will contribute data for the swine, broiler and turkey sectors. Researchers in TX and CO will assemble data from the major cattle-feeding states (TX, KS, NE, CO, IA, ID) for the fed-beef industry. Many evaluations of manure treatment technologies have at best included energy in an economic analysis. Although a good start, this assumes that energy costs will remain constant. Clearly when the price of energy is volatile the economic analysis of a treatment technology is altered. As energy prices increase, the cost to run a technology increases. The cost of synthetic fertilizer also increases. Energy is but one example of the effect of radical changes in a volatile parameter as the assumptions for input costs can clearly bias policy. If installation costs are high and energy costs increase sufficiently to require halting the operation of the technology a farm may need to address significant financial hardship. Sub-Task ii. Develop a framework for reporting the identified information A reporting framework is necessary in order that the analytical models are linked to the database of information that is gathered from all project participants. After workers under Sub-Task i are able to evaluate the inputs available and develop recommendations on how data should be reported, the framework for future reporting can be developed and made available to all project participants. We anticipate this process taking at least the first year of the project to evaluate inputs and the second year to develop the reporting framework. The critical issue includes spatial and temporal resolution, and having enough detail to assign flows to the appropriate segments of each production system. Participants from CA, NC and TX will lead this effort. Sub-Task iii. Identify critical control points (those points and processes in animal production that, when subjected to change will significantly impact the ecological footprint of animal agriculture) in mass, energy and contaminant flows in animal agriculture. To identify the critical control points accurately, the entire production chain must be considered; from cropping systems, feed storage and transportation, and the actual livestock system per se. Livestock production is essentially the conversion of feed nutrients into animal products, but the efficiency of conversion will vary depending on a large number of factors, including the optimization of feed composition. Participants from CA will be heavily involved in this portion of the project. Nutritionally-based models can be used for such optimization and will be used as the basis for contribution to this objective from researchers in MD, MI, and IN. Work previously conducted to assess dietary P content and bird performance will be re-evaluated to address the broader picture of the energy costs related to production of inorganic P and the costs at the feed mill to use inorganic P. This work will also address the costs/benefits of removing or reducing inorganic P in the diet, and costs (not price) of different sources of phytase that are used in conjunction with reduction of inorganic P. Work conducted in MD will also address litter quality in the house, animal density, nutrient changes by incorporating consideration of the real costs of strategies related to minimizing the environmental impact of animal production as it relates to N in the diet(to include not price but relative costs of production of grains/beans/meat meal and synthetic amino acids), including the impact of litter N content on its use as fertilizer. Beyond identifying the critical control points for animal production, researchers will use existing tools to evaluate factors that drive pollution potential. Researchers in MN, for example, will utilize an existing facility citing tool based on odor to identify critical control points for contaminant flow from animal production sites. Researchers in CA will use a production facility mass balance tool to identify critical control points related to nutrient sequestration at facilities. Task C. Define the measurable quantity(ies) that properly describe(s) the ecological footprint of animal agriculture, which is its true impact. This task must be undertaken in conjunction with the conceptual development of the model construct such that commonality of approaches and definable units are determined. We anticipate that this portion of the project will occur no earlier than the third year. All participants contributing to this objective will be involved in this process, with leadership provided by TX and NC. Task D. Assessment of the model structure through application to feed-to-field situations. Researchers involved in each of the tasks under objective 2 will have the opportunity to participate in evaluation of the model. A coordinated evaluation will occur between participants from MI, MN, CA, and GA to address the feed-to field components under a single application. These participants represent agronomists, engineers, and animal scientists. Solicitation of assistance from economists and ecologists will occur prior to the start of the evaluation. Objective 2. Continue the development and performance evaluation of process-level strategies and tactics to reduce environmental pollution at the process level from confined animal feeding operations. This work will include 1) management tools, strategies and systems for land application of animal manures and effluents that optimize efficient, environmentally friendly utilization of nutrients and are compatible with sustained land and water quality; 2) physical, chemical and biological treatment processes in engineered and natural systems for management of manures and other wastes; 3) methodology, technology, and management practices to reduce odors, gases, airborne microflora, particulate matter, and other airborne emissions from animal production systems. As an integrated project, each sub-task will include research and outreach efforts over the course of the project term from participating institutions and agencies. Task A. Develop management tools, strategies and systems for land application of animal manures and effluents that optimize efficient, environmentally friendly utilization of nutrients and are compatible with sustained land, air and water quality. Sub-Task i. Methods to reduce nutrient movement from land application sites into surface and groundwater. Planned efforts will address current research interest in alternative energy sources and the resulting byproducts. Work, originating in GA, will quantify on-site and off-site environmental effects of herbaceous bio-energy crop production systems using chicken litter as fertilizer while work in AL will determine fertilizer value of poultry litter ash resultant of ethanol production. Research will continue to address nutrient accumulations in soils as a result of manure applications. This work includes projects in GA to determine the effect of strip versus conventional tillage systems receiving poultry litter on N cycling and C accretion rates in Coastal Plain soils and projects to determine mineralization rates of poultry litter in Coastal Plain soils (GA). Recognizing the importance of balancing manure nutrients with crop needs, MN will conduct experiments involving solids/liquid separation and pretreatment of manure using chemicals or biological methods to conserve nutrients and prevent runoff. Sub-Task ii. Quantify gaseous emissions into the air from land application sites. Gaseous emissions following land application of manure is expected to undergo greater scrutiny as air emissions regulations are further developed. In order to develop management practices that effectively reduce emissions, the impacts of current practices must be addressed. Under this project data will be gathered that quantifies ammonia emissions following big gun and drag hose application of swine lagoon effluent to Coastal bermudagrass (NC). Efforts lead by participants in AL will determine the impact of landscape position and manure management on GHG emissions from agroecosystems while TX will lead a federal multistate project directed at odor, dust and gaseous emissions and abatement strategies for open lot feedlots and dairies. Sub-Task iii. Reduce movement of zoonotic pathogens and antibiotics from land application sites. Pathogen, pharmaceutical, and endocrine disruptors have received increasing attention from consumers and regulators alike. To be sustainable and a good environmental citizen it is imperative that animal agriculture respond to the increased attention and that research address strategies to reduce any negative contributions that animal production systems make. Therefore, work will be conducted under this project to evaluate management strategies for minimizing off-site transport of antibiotics and antibiotic resistance genes (CO) including evaluation of several chemical treatments of broiler poultry litter to reduce or eliminate zoonotic pathogens in the litter during the poultry production cycle. Researchers in CO will also measure antibiotic concentrations in manure stockpiles and lagoons. Pathogen monitoring will include E. coli, Salmonella, and the LT virus (laryngotrachitis). The litter treatments also will be evaluated for the control of ammonia in the poultry house (AL). The anticipated outcomes of these efforts are: 1) reduced transmission/incidence of disease among animals in production units, 2) reduced incidence of foodborne illnesses-associated with consumption of poultry products, 3) development of a strategy to control the LT virus, and possibly the poultry influenza virus, in poultry production units, and 4) elimination of pathogens in poultry litter prior to land spreading or transporting offsite. In addition GA will lead a project to evaluate the effects of farm ponds on the survival and transport of pathogens in runoff from beef cattle pastures where cattle use some of the ponds as a water source and for cooling. The project will include indicator organisms naturally present in the ponds and pathogens within diffusion bottles held in pond water. Sub-Task iv. Improve accuracy of manure land application in accordance with best management practices for nutrient planning. As part of efforts to ensure that nutrients are applied appropriately (not over-applied) a number of projects will be conducted to address land application from manures from multiple species. Researchers in CO will lead efforts to evaluate the N mineralization rates for cattle manure and compost top-dressed onto pasture or hay while NC will coordinate the determination of swine lagoon liquid nutrient concentrations variation with time and other factors such as pump-down levels. The fertilizer value of compacted poultry litter transported from areas of high CAFO concentration will be determined with efforts lead by researchers at AL. Extension education efforts to package GPS based manure application into an affordable, sufficiently accurate system for livestock producers and custom manure haulers, and demonstrate technologies wherever appropriate will be headed by IL. Task B. Development, evaluation, and implementation of methodology, technology, and management practices to reduce water pollution potential from animal production systems. Sub-Task i. Develop and evaluate innovative applications of engineered biological treatment processes to stabilize waste, reduce odor, and manage nutrients. There remains a tremendous need to develop systems that can economically treat manure to minimize environmental impact. As part of this project, systems will be developed at lab-, pilot-, and field-scale. Systems to be evaluated include solids/liquid separation systems (MN), aerobic/anoxic/anaerobic sequencing batch reactors (MN), anaerobic digestion (MN, CA), and surface aeration for odor control. Work will address design and operational criteria and economic evaluation of anaerobic processes (CA, HI) for stabilization and reuse of livestock waste/wastewater and demonstration and evaluation of additional selected new and innovative technologies specific for treatment of dairy manure effluent for waste pollution control (TX). Economic evaluations will be lead by NC participants. Sub-Task ii. Develop and evaluate vegetated or aquaculture-based treatment systems for treating wastewater or runoff from concentrated feeding operations or land application sites. In addition to engineered technologies, participants in the project will evaluate natural systems as options for animal production systems. Specific efforts will include data collection from vegetative filters from Midwest beef feedlots (MI, MN, NE). IL will study vegetative filters for treating runoff from CAFO feedlots, focusing on nutrients and pathogen transport/removal. Development of strategies to reduce NPS pollution of land applied manure P with phyto-remediation in Bosque and Leon River Watersheds will result from workers in TX. Hydroponic forage production appears to warrant further research as a means of recovering nutrients from biogas digester effluent, while producing feed and purifying the water. After further refinements to a small scale system, GA will lead attempts to develop and demonstrate a field scale system. Participants from GA will also lead efforts to determine the biomass production rates of grass, wetland, or horticultural species grown as floating vegetative mats in animal wastewater lagoons and evaluate the value of this material as soil amendment following composting, or, for the grasses, the feasibility of sod production and value of the sod. Sub-Task iii. Develop and evaluate physical and chemical treatments for recovering or stabilizing manure solids or manure treatment by-products for improved utilization alternatives. In addition to work involving solids/liquid separation with or without addition of chemicals (MN), participants working under this objective will evaluate the mass balances of C, N, P, and salts that result from quasi commercial treatment technologies to determine alterations in manure nutrient flow (CA) as well as the potential for P removal from dairy effluent through struvite production (CO). A surface draining settling basin (Oyzboyd Dry Bed) will be evaluated by GA participants as a potentially improved technology for solids recovery from the waste stream of flush cleaned animal facilities. Workers in NC will continue development of alternative swine manure management systems that facilitate utilization of separated feces and urine without using water wash techniques. TX will lead efforts to evaluate combustion/gasification ash recovery processes. Participants from CO will evaluate the potential for composting to convert antibiotic-laced manure and mortalities treated through alkaline digestion into high quality horticultural compost. Sub-Task iv. Develop and evaluate biological or thermochemical treatment of animal manures and mortalities for conversion into renewable energy and/or value-added products As technologies are developed to provide alternative energy sources there will be continued need to conduct mass balance (C, N, P, S, salts) and energy efficiency analyses from these systems. CA and HI will lead evaluations for on-farm dairy biogas systems including design/operational criteria for anaerobic processes to enhance methane gas production and maximization of the bio-production of animal/agricultural feed ingredients using animal/fruit processing wastes . TX researchers will determine the effects of feedlot manure and open-lot dairy manure management practices on manure characteristics for biomass conversion systems including reburn (NOx or Hg control) or co-firing with coal/lignite. Design/operational criteria for anaerobic Bio-nest process to enhance methane gas production. MN will also be involved in this objective evaluating fermentation to produce biohydrogen and anaerobic digestion to produce methane. Both MN and IL will conduct projects with the thermochemical treatment of manure solids to produce bio-oils and other value-added product. IL will plan and build pilot scale facility for commercializing thermochemical treatment of swine manure and other ag byproducts into oil and separating the plant fertilizer constituents from the post-processed water. GA researchers will study the black soldier fly which holds promise to efficiently and economically convert manure into valuable feed ingredients (protein) and energy (oil for biodiesel production). Research will focus on developing procedures, equipment, and facilities that assure reliable cold weather production of soldier fly prepupae. Sub-Task v. Develop and evaluate feeding strategies as a means of reducing water quality impacts and transfer findings to industry for implementation. Development of mitigation strategies includes use of feeding programs as a means to reduce environmental impact. A number of participating states will engage in this aspect of the project, addressing diet modification options for both ruminants and non-ruminants alike. Specific projects will include but not be limited to determination of optimal combinations of feedstuffs and feed additives to maximize nutrient utilization while assessing nutrient mass balance (IN, MI, KY, MD), determination nutrient mass balance in laying hens during a laying cycle when fed a range of dietary phosphorus (IN), implementation and standardization of an ileal amino acid digestibility determinations in poultry (IN). Nutrition models will be adapted in order to better predict the impact of diet modification on nutrient losses in excreta. Task C. Develop and evaluate methodology, technology, and management practices to reduce odors, gases, airborne microflora, particulate matter, and other airborne emissions from animal production systems. Sub-Task i. - Develop standard methods of collection, measurement, and mitigation of airborne emissions (odors, gases, particulates, endotoxins, pathogens, and other materials) from animal production operations Several participating state will continue to work on developing methods for airborne emission measurements from animal production systems, including hazardous and greenhouse gases, particulate matter, and odor (MN, MI, TX). This includes development of ammonia, hydrogen sulfide and reactive VOC measurement protocol and emission factors from open-lot and free-stall dairies as well as from confined facilities. An additional component of this objective is the determination of the impact of odor and other hazardous gases on local communities and neighbors. Sub-Task iii. - Emission control technology development, evaluation and selection for site-specific cases. To address continued focus on air emissions from animal production systems citing tools will continue to be developed (MN, MI). Continued development of alternative manure management practices on odor generation & abatement will emphasize cattle feedlots (TX) while CO will evaluate the efficacy and cost of ammonia BMPs for feedlots and dairies. Researchers in TX and MN will provide leadership in the development and demonstration of an efficient aeration technology to control odor from poultry layer manure (TX) and swine lagoons (MN). Sub-Task iv. Develop and evaluate feeding strategies as a means of reducing water quality impacts and transfer findings to industry for implementation. Diet modification studies will be conducted to determine baseline emissions and mitigation options for air emissions. Components of the task include determination of the effect of diet modification on form and fate of nutrients in manure and air emissions (MI, MD, IN), quantification of the influence of formulation to a digestible amino acid basis and dietary supplementation zeolites and acidulants on excreta pH and reduction of ammonia and other gaseous emissions in multiple species (IN, MI, NC), determination of mass balance and nutrient volatilization in male turkeys during a growing cycle when fed an industry diet versus that of a lowered nutrient diet (IN, MI, MD), quantification of baseline emissions from growing and lactating cattle when fed different rations (MI, CA), and evaluation of feeding distillers grains to ruminants and non-ruminants on air emissions from animal production systems (MI, MN). evaluation of the impact of dietary acidification on excreta pH and ammonia/ammonium ratio from turkeys and laying hens (IN).Measurement of Progress and Results
Outputs
- Professional development at our annual meetings. Submission of a proposal to EPA for ecological footprint conference. Proposed symposium on Environmental Footprint Analyses at professional society meetings to increase awareness (ASABE, ASAS, ASA). Annotated bibliography of the key literature for individuals interested in concept (scientists, policy makers, animal operators). Publications: White paper(s) to describe various measuring sticks, data collection needs to accomplish analysis, curriculum for college course. Outreach activities: articles in popular press/trade association outlets and mini briefing documents.
Outcomes or Projected Impacts
- Verified systems-level model(s) are published that can be used to evaluate the ecological impacts of changes in the animal agriculture industry. These models and the research they will inspire will lead to reduced inputs to food animal production, greater reuse and recycling of manure resources and improved environmental quality.
Milestones
(2008): Within the first year, we will develop a conceptual model structure (obj. 1) for future reporting of data generated under objective 2. This structure will enable those participants working on model development to identify un-addressed needs in this systems approach. We will submit a manuscript describing this structure for peer review in late 2008.(2009): By July 2009, we expect to have a working example of the model structure that includes data generated under objective 2.
(0):sequent years will see continued refinement of the model as well as development of computer code to implement the model. Published data and data shared from other project participants will be used to validate and test model components. Annual meetings will provide all researchers a chance to interact and contribute to project objectives.
Projected Participation
View Appendix E: ParticipationOutreach Plan
As a result of the five-year plan, the committee will develop and publish a white paper describing the economic and ecological implications of the methodology used in waste management research and the inherent variability of sampling, analysis, and calculations. The examples used and conclusions reached will be based on information collected from the process-oriented research conducted under this project followed by modeling of the research findings to assess the total ecological impact. In addition, each participating scientist will develop research papers and outreach tools and information individually or as part of a team to address state-specific or regional needs. The USDA/EPA Livestock and Poultry Environmental Stewardship Curriculum will be updated with BMPs (best management practices) and economic data provided funding becomes available. University web sites will summarize project results. Refereed, peer-reviewed and Extension publications will be published, with emphasis on information applicable by the end user. The project will produce one or more Southern Regional publications summarizing the conclusions of various aspects of the project. Demonstration field days will be held showcasing many of the facets of the project throughout the region. Implementation of best management practices by producers, with resultant improvements in water and air quality, will have a positive impact on the environment and the sustainability of the animal industry. Appropriate site-specific nutrient management plans will be written for farms and maintained. Technologies will be adopted by producers for economical treatment of manure and production of value added commodities.
The primary outcome of objective 1 will be a greater awareness of the need to work more broadly and share data with those that can evaluate impacts on a systems level, whereby system is beyond the farm boundary. Findings will contribute to curriculum for college courses, further increasing the impact of our work. Specific outputs for objective 1 will include professional development opportunities at our respective disciplinary annual meetings including a proposed symposium on Environmental Footprint Analyses at professional society meetings to increase awareness (ASABE, ASAS, ASA). In addition we plan to submit a proposal to EPA for ecological footprint conference (2007). Print outcomes include an annotated bibliography of the key literature for individuals interested in concept (scientists, policy makers, animal operators) and white paper(s) to describe various measuring sticks and data collection needs to accomplish analysis, articles in popular press/trade association outlets and mini briefing documents.
Outputs that result from objective 2 will include peer review publications, a 2-page fact sheet for each task, to be updated annually, presentations at conferences and scientific meetings, popular press articles, a web-based curriculum, identification of technologies which provide environmental improvement, methodology for technology evaluation and comparison, contribution to national effort to estimate air emissions from various species and components of AFO, proposed standard methods for testing and monitoring protocols and laboratory methodology procedures where appropriate. Criteria to evaluate process efficiencies, energy efficiencies, mass balance calculations, net/gross resource consumption, land base needs, and additional data from objective 2 will provide input into objective 1.
Organization/Governance
Chair: The chair of the committee is responsible for organizing the meeting agenda, conducting the meeting, and assuring that task assignments are completed. The chair is elected for a one-year term. Chairs are eligible for reelection.
Chair-elect: The chair-elect normally succeeds the chair, and is expected to support the chair by carrying out duties assigned by the chair. The chair-elect serves as the chair in the absence of the elected chair. The chair-elect is elected for one year. The chair-elect is eligible for reelection.
Secretary: The secretary is responsible for the distribution of documents prior to the meeting. The secretary is also responsible for keeping records on decisions made at meetings (a.k.a. keeping the minutes), maintaining an updated roster of participants (as a list server), and assisting in the preparation of the accomplishments report (i.e., the SAES-422). The secretary normally succeeds the chair-elect. Secretaries are eligible for reelection.
Members: In addition to carrying out the agreed research collaboration, research coordination, information exchange, or advisory activities, project members are responsible for reporting progress, contributing to the ongoing progress of the activity, and communicating their accomplishments to the committee's members and their respective employing institutions.
Chair, Todd Applegate, Purdue University.
Chair-Elect, John Classen, North Carolina State University.
Secretary, Ted Funk, University of Illinois.
Literature Cited
Allen, V. G. 2006. Using grazing animals to restore resilience in our agricultural systems. Proceedings of the John M. Airy Symposium: Visions for Animal Agriculture and the Environment, Kansas City, MO, January 5-7.
Costanza, R. (ed.). 1991. Ecological Economics: The Science and Management of Sustainability. New York: Columbia University Press. 525 pp.
Hall, C. A. S. (ed.). 1995. Maximum Power: The Ideas and Applications of H. T. Odum. Niwot, CO: University Press of Colorado. 393 pp.
Hubbert, M. K. 1949. Energy from fossil fuels. Science 109:103-109.
Hubbert, M. K. 1982. Personal annotation to L. F. Buzz Ivanhoe. In: NBS Special Publication 631, pp. 140-141.
Lifset, R., et al. (eds.). 2005. Special Issue on Eco-Efficiency. Journal of Industrial Ecology. Cambridge, MA: Massachusetts Institute of Technogy Press. Volume 9, Number 4.
Manning, R. 2004. The oil we eat. Harpers 308:37-45.
Marek, T. H. 2005. Personal communication. Texas Agricultural Experiment Station, Amarillo, TX.
Odum, H. T. 1996. Environmental Accounting: Emergy and Environmental Decision Making. New York: John Wiley and Sons, Inc. 370 pp.
Rees, W. E. 1997. Urban ecosystems: the human dimension. Urban Ecosystems 1(1):6375.
Sweeten, J. M. 1999. Production and environmental quality model for beef production operations. Report provided to the Consortium for Cattle Feeding and Environmental Sciences (CCFES), Amarillo, TX.
Wackernagel, M. and W. E. Rees. 1996. Perceptual and structural barriers to investing in natural capital: economics from an ecological footprint perspective. Ecological Economics 20(1):3-24.