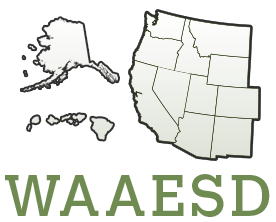
W1150: Exotic Germplasm Conversion and Breeding Common Bean (Phaseolus vulgaris L.) for Resistance to Abiotic and Biotic Stresses and to Enhance Nu
(Multistate Research Project)
Status: Inactive/Terminating
W1150: Exotic Germplasm Conversion and Breeding Common Bean (Phaseolus vulgaris L.) for Resistance to Abiotic and Biotic Stresses and to Enhance Nu
Duration: 10/01/2005 to 09/30/2010
Administrative Advisor(s):
NIFA Reps:
Non-Technical Summary
Statement of Issues and Justification
STATEMENT OF THE PROBLEM. Dry bean (Phaseolus vulgaris L.), introduced by the Native Americans from Mexico, Central America, and South America, has been grown in the U.S. for millennia. However, compared to corn, soybean, and wheat, the average national yield of dry bean is low (=1700 lbs/acre). Several abiotic and biotic stresses can severely limit both dry and snap (vegetable form) bean production. Furthermore, several diseases can simultaneously reduce dry and snap bean yield and quality within and across different production regions and yield losses can range from 10-90%, depending on the diseases involved. For example, in the Western U.S., Beet curly top virus (BCTV), Bean common mosaic virus (BCMV), Fusarium root rot (caused by Fusarium solani f.sp. phaseoli), and white mold (caused by Sclerotinia sclerotiorum) can simultaneously attack susceptible cultivars in the same field. Similarly, in Michigan, Minnesota, North Dakota, and Wisconsin, anthracnose (caused by Colletotrichum lindemuthianum), bacterial brown spot [caused by Pseudomonas syringae pv. syringae (Psp)], BCMV, common bacterial blight [caused by Xanthomonas campstris pv. phaseoli (Xcp) and X. campestris pv. phaseoli var. fuscans (Xcpf)], halo blight (caused by Pseudomonas syringae pv. phaseolicola), root rots, rust (caused by Uromyces appendiculatus), and white mold can be sympatric and cause severe yield losses. The recent introduction of soybean rust into the southeastern U.S. has serious potential implications for dry and snap bean as they are susceptible to the soybean rust pathogens, Phakopsora pachyrhizi and P. meibomiae. Furthermore, many of these diseases are seed-borne, are caused by pathogenetically variable organisms, and their chemical control can be expensive or not practical. Fungicides increase production costs and can result in environmental and human health hazards if improperly used.
The genetic base of dry bean cultivars within most market classes in the U.S. is narrow (McClean et al., 1993; Miklas, 2000; Silbernagel and Hannan, 1992; Sonnante et al., 1994). As only a very small number of wild beans have been domesticated (Gepts et al., 1986), useful traits such as resistance to bruchids (Zabrotes subfasciatus) are not found in cultivars (Schoonhoven et al., 1983) supporting the evidence that a large reduction in genetic diversity occurred early during domestication (Gepts et al., 1986; Koenig et al., 1990). Furthermore, resistance to heat, drought, and diseases such as Bean golden yellow mosaic virus (BGYMV), common bacterial blight, and white mold are inadequate in most cultivars grown in the U.S.
This interdisciplinary, multi-state, collaborative W-150 project proposal comprises several complementary sub-projects (see Appendix Table 1). Collaboration among participants in these sub-projects is designed to achieve our overall goals and objectives of developing high yielding cultivars with enhanced culinary and nutritional qualities and resistance to major abiotic and biotic stresses. These cultivars will help reduce production costs and pesticide use, increase yield and competitiveness of the U.S. bean growers, and sustain production for domestic consumption and export. Researchers participating in each sub-project have complementary expertise and represent two or more institutions. They have jointly prepared their sub-project documents and are committed to collaborating with each other to achieve the overall objectives. For simplicity, these projects are grouped into: (1) exotic germplasm conversion, (2) abiotic stresses, (3) biotic stresses, and (4) nutritional value. Details of each sub-project listed in Appendix Table 1 will be provided upon request.
Exotic Germplasm Conversion. Most tropical and subtropical dry bean germplasm are highly sensitive to long days in the U.S. mainland bean growing environments. This sensitivity inhibits flower and seed formation. Consequently, photoperiod-sensitive tropical germplasm must be converted to temperate adaptation before it can be used to broaden the genetic base and improve cultivars in the U.S. for yield, enhanced culinary and nutritional qualities, and resistance to major biotic and abiotic stresses.
Abiotic Stresses. Although drought, high temperatures (>30 degrees C.), and soil mineral deficiencies and toxicities can adversely affect bean production in the U.S., the primary emphasis in this project will be on breeding for resistance to high temperatures. Evaluation for other abiotic stresses will be limited to only the most promising breeding lines and cultivars through regional and national nurseries. High temperatures reduce yield, quality, and geographic adaptation of cultivars. Production areas in the U.S. occasionally experience seasonal heat waves during flowering, resulting in blossom drop and a split time period for pod set. Heat stress also increases pollen sterility and distally located ovules often fail to develop, reducing seed number per pod in dry bean and pod quality in snap bean.
Biotic Stresses. Most cultivars are highly susceptible to numerous diseases caused by bacterial, fungal, and viral pathogens. In this project, we will focus on common and halo bacterial blights, Fusarium root rot and wilt (caused by F. oxysporum f. sp. phaseoli), geminiviruses such as beet curly top (in the Western U.S., Larson and Miklas, 2004) and bean golden mosaic (in southern Florida and Puerto Rico; Blair et al., 1995), rust (east of the Continental Divide), and white mold. Yield losses from these diseases can vary from 10% to 90%. In the case of white mold, the fungus produces sclerotia that can survive in soil for years and can be spread from field to field by internally infected seeds, sclerotia mixed with seed, contaminated soil on equipment, and irrigation runoff water, in addition to wind-blown ascospores. Common and halo bacterial blights are seed-borne, thus restricting free movement of seed from one production region to another and limiting seed production in other regions. Moreover, halo blight and rust are caused by variable pathogens, such that cultivars that are resistant in one location or year may be susceptible in another location or year. Cultivars that contain single gene resistance are vulnerable to new pathogen race. Chemical control for most of these diseases is difficult and sometimes impractical because the pesticides are expensive, ineffective, and damaging to the environment.
Nutritional Value. Dry beans have been widely shown to have significant health benefits, however they are under-utilized in the diets of people worldwide. Health benefits from eating beans are numerous and include the provisions of low fat and high protein, calories, dietary fibers, vitamins, iron, potassium, calcium, iron, zinc, phosphorus, and other essential nutrients. Several of the most severe chronic health problems (e.g., certain types of cancer, Type 2 diabetes, and cardiovascular diseases) in the U.S. could be prevented or alleviated by eating more beans (Andersen et al., 1984; Tietyen-Clark, 1986). Therefore, identification and promotion of the health-benefits of dry beans are extremely important. Moreover, a greater emphasis must be placed on providing more diversified bio-fortified bean-based foods and convenience snacks with improved nutrition and consumer appeal.
JUSTIFICATION. Exotic Germplasm Conversion. An important limitation to the genetic improvement of bean cultivars in the U.S. is the narrow genetic diversity currently available to researchers. Most of the tropical and sub-tropical germplasm are highly photoperiod sensitive such that they may not flower and produce seed on the U.S. mainland. Conversion of useful exotic germplasm for adaptation to the U.S. bean growing environments, and introgression and pyramiding of favorable alleles and QTL for resistance to major abiotic and biotic stresses, and enhanced yield and nutritional value are essential for broadening the genetic base of cultivars. Also, it will permit the introduction of new market classes of beans to the U.S. farmers. The study of the genetic and molecular basis of the principal domestication traits will speed the introgression and pyramiding of favorable alleles and QTL especially from wild bean.
Abiotic Stresses. Resistance to high temperatures and drought occurs in a few tropical cultivars, although tepary bean (P. acutifolius) possesses much higher levels of heat and drought resistance (Thomas et al., 1983). Resistance to heat and drought stresses in bean are heritable (Schneider et al., 1997; Shonnard and Gepts, 1994; Singh, 1995). Thus, introgressing and pyramiding heat (and drought) resistance from other Phaseolus species into dry and snap bean cultivars will increase yield and yield stability, and extend adaptation to marginal environments.
Biotic Stresses. We have limited information regarding the nature of the viruses that cause curly top disease in the Western U.S. Concurrent pathogen surveys are also needed for other geminiviruses, Fusarium root rot and wilt, common and halo bacterial blights, rust, and white mold. Knowledge of pathogen diversity is critical for the identification and deployment of appropriate specific resistance genes and QTL to combat these diseases on a regional and national basis. There is a need to carry out comparative studies of Agrobacterium-mediated inoculation (agroinoculation) and vector-based inoculation methods for geminiviruses to assure that resistant cultivars developed using agroinoculation in the lab and greenhouse are also resistant in the field under vector pressure. Similarly, for white mold, yearly testing of resistance donor and improved germplasm using different screening methods in greenhouse, laboratory, and multiple field environments is essential to identify both disease avoidance mechanisms and true resistance. In the past, W-150 researchers have developed germplasm and cultivars resistant to anthracnose, BCMV, BGYMV, Fusarium root rot and wilt, rust, and white mold. However, in some cases the genetic base of resistance is narrow, vulnerable to new pathogen strains, and/or is inadequate to reduce crop losses. At present, only one or two major Andean resistance genes for anthracnose and rust have been identified and deployed (Kelly and Vallejo, 2004; Pastor-Corrales, 2003). There is an urgent need to identify and introgress additional Andean resistance genes for these diseases caused by highly variable and virulent pathogens. Similarly, introgression and pyramiding of high levels of resistance from other Phaseolus species for bean golden mosaic, white mold, and other diseases are essential. Development of common bacterial blight resistant breeding lines from crosses of common bean with tepary and runner beans was a major milestone (Miklas et al., 1994; Singh and Muñoz, 1999). Similarly, identification of molecular markers (BC420, SAP6, SU91) linked with each of the three major QTL imparting resistance to common bacterial blight has facilitated breeding for resistance (Kelly et al., 2003; Yu et al., 2000). Nonetheless, molecular markers for other major and minor QTL for resistance to anthracnose, BCMV, common and halo bacterial blights, rust, white mold, and other diseases must be identified to facilitate cultivar development.
Nutritional Value. The potential for beans to prevent and alleviate chronic diseases is not well known. Research to identify bean components that mitigate chronic diseases is essential as are outreach efforts to promote awareness and acceptance of beans as a healthy food. Therefore, both bean genetics and food science must be utilized to reduce constraints that contribute to low per capita consumption of beans in the U.S. The number of convenience entrees and snack items prepared from beans should be increased considerably. Also, avoidance of beans because of flatulence continues to be a major constraint and must be addressed in the future.
Need for a Multi-State Collaborative Project. For conversion of useful tropical and sub-tropical germplasm that are poorly adapted to the U.S. bean growing environments it is necessary to make adapted x exotic crosses and backcrosses during short-days in the tropics (e.g., Mayaguez, PR) (or in the greenhouse during the winter months). Furthermore, crossing and backcrossing in the tropics should be alternated by selection for photoperiod insensitivity on the mainland during the summer months. The use of winter nurseries in Puerto Rico complements and expedites germplasm conversion, and the mainland U.S. breeding for other constraints.
Anthracnose, curly top, halo blight, rust, and other diseases caused by variable pathogens, require testing using different screening methods and multi-location field and greenhouse environments. Also, for white mold field and greenhouse data from a single location often do not permit the identification of avoidance and physiological resistance with any degree of assurance. It is therefore essential to continue to characterize and monitor genetic variability of bacterial, fungal, and viral pathogens causing major bean diseases in the U.S. Also, it is imperative to determine the reaction of useful germplasm to the pathogenic diversity to identify complementary resistance genes and mechanisms for broadening the genetic base and development of improved cultivars. Introgression and pyramiding of favorable alleles and QTL from across races, gene pools, and related wild and cultivated Phaseolus species into cultivars is often achieved only through a tiered breeding approach (Kelly et al., 1998; Singh, 2001; White and Singh, 1991). Most researchers often work at one or two tiers, and depend on other collaborators for complementary germplasm and information to accomplish development of successful cultivars (Kelly et al., 1998). Inter-disciplinary and inter-institutional collaborative research must also continue to find alternative recombination and selection methods and identify and use molecular markers to facilitate efficient introgression and pyramiding of favorable alleles and QTL into improved cultivars for a diverse cropping system. Thus, to develop germplasm and cultivars with multiple-disease resistance and abiotic stresses, researchers with limited expertise and facilities share responsibilities and exchange segregating populations and breeding lines to complement screening and selection in contrasting field environments, laboratories, and greenhouses regionally and nationally.
Because exotic germplasm is increasingly being used to broaden the genetic base and breed cultivars for higher yield, enhanced nutritional quality, and resistance to abiotic and biotic stresses, it is essential to evaluate new breeding lines and cultivars across production regions for broad adaptation and stability of performance. Regional and national germplasm development and testing are also important because only one field crop/year is feasible in the continental U.S. Although not described separately, multi-location testing is accomplished through the national Cooperative Dry Bean Nursery (CDBN), Bean Rust Nursery (BRN), White Mold Nursery (WMN), Midwest Regional Performance Nursery (MRPN), and Western Regional Bean Trial (WRBT). These nurseries are essential to identify high yielding broadly adapted cultivars and breeding lines with durable resistance, and to detect pathogen diversity in the shortest time possible. These nurseries therefore form an integral part and sound basis for strong collaborative efforts within the W-150 project. No single state or institution can conduct all the research necessary to develop improved cultivars for sustainable production, consumption, and export. This is especially true when most programs are grossly under-funded and have inadequate resources and personnel to carryout a relevant and efficient breeding program for their own state. Certain expertise is available in a few states (e.g., nutritionists and pathologists), but there are some states (e.g. Arizona, Montana, New Mexico, and Wyoming) that do not have dry or snap bean breeding programs. As in the past, researchers in these states also will have access to new breeding lines and cultivars of all market classes through the W-150 project. Moreover, research and outreach efforts of agronomists, breeders, geneticists, food scientists, human nutritionists, and plant pathologists must be coordinated to improve domestic consumption and export. No single research institution is capable of providing the breadth of expertise necessary to achieve regional and national impact. Thus, additional resources and multi-state regional and national collaboration are essential to solve major abiotic and biotic stresses, yield, and food quality problems that currently limit the yield potential and domestic consumption and export of dry and snap bean. This comprehensive, multidisciplinary, and multi-state collaborative project is essential to convert useful exotic germplasm; and to maintain and exchange pathogens, parental stocks and improved breeding lines and cultivars, and research data. The collaborative project provides a broad range of selection environments whereby researchers can share and complement responsibilities that could not be achieved by any single state or institution alone. Moreover, a coordinated, multidisciplinary effort will allow the most efficient use of genetic resources, avoid duplication of research, and maximize efforts to increase bean production, consumption, and export.
Related, Current and Previous Work
Exotic Germplasm Conversion. The traits that distinguish wild and domesticated beans have been described (Gepts and Debouck, 1991). They include growth habit, seed dispersal and dormancy, pod and seed size, pigmentation, and photoperiod sensitivity. Alleles and QTL controlling these traits have been identified and mapped (Koinange et al., 1996). Thus, alleles and QTL that should facilitate exotic germplasm conversion are the St for pod string, a QTL for seed dormancy, fin for growth habit, and ppd and hr for insensitivity to photoperiod. The ppd is among the main alleles (Gu et al., 1998) to be used for recurrent backcrossing to exotic germplasm (see Appendix Table 2).
Abiotic Stresses. Heat resistant snap beans Cornell 502 and Cornell 503 were crossed with 'Venture' and HB 1880 for breeding and genetic studies. The recombinant inbred lines (RIL) have been evaluated in greenhouse and field environments (Rainey and Griffiths, 2005). The RIL from dry bean population A55 x G122 also have been evaluated for heat resistance (Porch, 2001). Data from these populations will be used for the genetic dissection of heat resistance during the reproductive phase into its components, such as bud abscission and pod set, for quantitative genetic analysis, and for the identification of molecular markers.
Of the 25 P. acutifolius accessions tested, PI 200902, PI 312637, PI 440785, PI 440788, and PI 440789 exhibited the highest yield and yield component stability under high temperatures (35°C/32°C) (Rainey and Griffiths, 2004). These accessions were crossed with the dry bean cultivar ICA Pijao using embryo rescue, and backcrosses are being made.
Biotic Stresses. Common bacterial blight. A rapid PCR-based detection system was developed for Xcp and Xcpf that greatly reduces the time needed for detection of bacteria recovered from seed and other sources. Also, repetitive element PCR (rep-PCR) was used to examine pathogen diversity. These studies supported the concept that Xcp and Xcpf are genetically distinct bacteria. Xcp was strongly associated with Andean common bean, whereas Xcpf was associated with Andean and Middle American genotypes. The development of host differentials is being investigated for Xcp. Common bacterial blight resistant breeding lines such as XAN 159, VAX 1, VAX 3, VAX 4, VAX 6, and Wilkinson 2 have been crossed with black, navy, great northern, pinto, dark red kidney, cranberry, yellow, and snap bean breeding lines and cultivars. In some crosses, molecular markers, namely SAP6, SU91, and/or BC420 are being used to facilitate the backcrossing of specific resistance QTL. Early generation segregating families and breeding lines are being selected for plant type, maturity, and seed characteristics in addition to resistance-linked markers and direct selection for resistance to the pathogen itself. Also, RIL from each of 'Othello'/VAX 1, Othello/VAX 3, 'Red Hawk'/VAX 3 crosses are being developed to study the inheritance of resistance present in VAX 1 and VAX 3, and to find and tag new QTL. Fusarium root rot and wilt. Cramer et al. (2003) found low genetic diversity among 166 isolates of the Fusarium wilt pathogen from the U.S. Central High Plains using RAPD markers. Resistance to Fusarium wilt in race Durango dry beans CO 33142 and 'Fisher' were controlled by a single dominant gene, whereas polygenic control (h2 ranged from 0.25 to 0.60) was found for resistance in race Mesoamerica cultivars Rio Tibagi and Jamapa (Cross et al., 2000; Velasquez and Schwartz, 2000). Nonetheless, Fall et al. (2001) found that breeding line A 55 of Mesoamerica race possesses the U20.750 RAPD marker on linkage group (LG) B10 which accounted for 63.5% of the variance for Fusarium wilt resistance observed in RIL. Heritability estimates for Fusarium root rot in FR266 ranged from 0.48 to 0.71 (Schneider et al., 2001). Several RAPD markers were identified that demonstrated significant associations with resistance in greenhouse and field.
Halo blight. A recent survey of Psp revealed that Race 6, which is virulent on most host-differentials, was most prevalent in North Dakota. Ariyarathne et al. (1999) identified a QTL in BelNeb-RR-1/A55 that conditioned resistance to halo blight. Subsequently, resistance genes Pse-1 and Psp-4 (on linkage group B4) were identified in the same population. Recombination within Psp-1 for resistance to Psp Races 1, 7, and 9 was observed, suggesting the gene itself represents a gene cluster (Fourie et al., 2004). Susceptible Canadian Wonder has been crossed with resistant A 52, A 43, and UI 3 to tag and map all major genes for halo blight resistance present in the host differentials. Other RIL populations for identifying resistance QTL from CAL 143 and PI 150414 are in development.
Geminiviruses. Recent genetic diversity studies of bean-infecting geminiviruses separated the BGMV occurring mostly in Argentina, Bolivia, and Brazil from the BGYMV found in Central America and the Caribbean. Furthermore, bean golden mosaic in Chiapas, Mexico is caused by a strain of BGYMV, BGYMV-MX. Infectious clones of BGYMV-MX were generated and an agroinoculation method was developed. Three inoculation methods for BGYMV-MX, namely agroinoculation, inoculation with sap, and particle bombardment were compared. Results differed depending on genotype, inoculation method, and stage of plant growth; results for Andean beans were more consistent compared with Middle American beans (Garrido-Ramirez et al., 2000). Recessive resistance genes, bgm-1, bgm-2, and bgm-3, reduce leaf chlorosis caused by BGYMV (Osorno et al., 2003; Velez et al., 1998), whereas the dominant Bgp-1 prevents pod deformation (Acevedo Roman et al., 2004). Dominant resistance, widely distributed within the Middle American gene pool, blocks the long-distance movement of the Bean dwarf mosaic virus (BDMV) (Seo et al., 2004).
Rust. The search for novel Andean and Middle American sources of rust resistance that will contribute to broadening the genetic base of snap and dry bean cultivars against the highly variable rust pathogen continues. In addition, studies to elucidate the inheritance of resistance and molecular markers linked to these genes continue. Similar studies of the phenotypic and genetic diversity of the rust pathogen using differential cultivars and molecular markers continue. These studies provide insights on the genetic diversity and the co-evolution of the rust pathogen with the common bean.
White mold. Moderate to high levels of white mold resistance were found in >20 P. coccineus (Gilmore et al., 2002) and common bean accessions (Grafton et al., 2002; Steadman et al., 2001). Approximately 550 breeding lines derived from crosses of ICA Pijao with P. coccineus, P. costaricensis, and P. polyanthus were screened for white mold resistance. Approximately 75 of the most promising interspecific breeding lines have been selected for further screening. Susceptible Othello and UI 320 were crossed and backcrossed with resistant P. coccineus PI 433246 and PI 439534 for development of inbred-backcross lines. A single dominant gene controlled inheritance of resistance in PI 433246 and PI 439534 (Schwartz et al., 2004). Moderate levels of resistance were accumulated in snap beans B7354, Cornell 601, and NY6020-4. Also, pinto Chase , AN 37, and CO 75944 with low to moderate levels of resistance were developed. Molecular markers linked to resistance QTL were identified in 'ICA Bunsi' (Ender, 2003; Kolkman and Kelly, 2003; Miklas et al., 2004), G 122 (Miklas et al., 2001a), PC-50 (Park et al., 2001), and NY 6020-4 (Miklas et al., 2003).
Nutritional Value. Major genes and QTL affecting canning quality (overall appearance and degree of splitting) were identified and mapped (Posa-Macalincag et al., 2002). These traits were highly correlated (0.91 to 0.97) and heritable (0.83-0.85). Black beans stored at 4.50C, 50-60% relative humidity, and 125 mm mercury for two years had physico-chemical characteristics comparable to freshly harvested beans (Berrios et al., 1999).
The relative ability of bean extracts to inhibit lipid peroxidation was red>brown>black >white (Beninger and Hosfield, 2003). Antioxidant activity was most strongly related to quantity of condensed tannins (proanthocyanidins). Anthocyanins in black beans and quercetin glycosides (yellow color present in a variety of beans) also contributed antioxidant activity. Beans without condensed tannins had low antioxidant activity.
Objectives
-
To broaden the genetic base of bean cultivars of major market classes,
-
To improve yield potential and resistance to major abiotic and biotic stresses affecting production,
-
To identify health promoting constituents and properties, and
-
To develop new bean-based products to increase consumption in the U.S. and globally.
Methods
Our overall strategy is to convert useful tropical and subtropical dry bean germplasm to adapt to the U.S. mainland growing environments. Subsequently, introgress and pyramid favorable alleles and QTL for enhanced yield potential, nutritional value, and resistance to multiple abiotic and biotic stresses using a multi-disciplinary and multi-institutional team approach that will sustain regional and national production, consumption, and export. To accomplish these objectives, our research activities are divided into various sub-projects (see Appendix Table 1) in which researchers from two or more participating states and institutions conduct research on each major problem as a team. To identify and set priorities for conversion of exotic germplasm, all W-150 participating researchers are periodically consulted and production problems and deficiencies in available germplasm are considered. The overriding theme of broadening the genetic base of dry and snap bean cultivars by introgression and pyramiding of favorable alleles and QTL from across market classes, races, gene pools, and wild populations within the primary gene pool and related Phaseolus species is embedded and emphasized within each project. Often a three-tiered breeding approach elegantly described by Kelly et al. (1998) and also discussed in considerable details in the W-150 project for FY 2000-2005 is used wherever appropriate. For example for white mold, introgression of resistance alleles and QTL from P. coccineus and other species in the secondary gene pool would be conducted at the base of the "breeding pyramid". Accumulation or pyramiding of resistance alleles and QTL from across the primary and secondary gene pools would be at the middle, and transfer into breeding lines and cultivars of each market class at the apex limited to elite x elite crossing and selection. Although not described separately in this project, our general strategy for yield improvement is to use high yielding breeding lines and cultivars as the base parents for crosses in each project. The concept of evolutionary origin, gene pool, and races (Gepts et al., 1986; Singh et al., 1991), and growth habit, maturity, seed characteristics, and combining ability along with traits of primary interest are considered for parental selection, crossing, and population development in each project. Breeding methods and selection techniques employed may vary from project to project depending upon the type of population and genetic distance between parents used, inheritance of the traits under improvement, screening or evaluation methods employed, and resources available. For diseases caused by variable pathogens, plant samples and/or pathogen isolates are collected and sent to the lead researcher (e.g., Fusarium to Schwartz, geminiviruses to Brown and Gilbertson, Xcp/Xcpf and Psp to Gilbertson, Sclerotinia to Steadman, and Uromyces to Pastor-Corrales) for characterization using the conventional and modern evolving PCR-based fingerprinting and other techniques. Similarly, the W-150 researchers strive to develop and improve upon the laboratory, greenhouse, and field techniques for efficient and economical screening of germplasm, segregating populations, families, breeding lines, and cultivars. Promising germplasm and improved breeding lines and cultivars from all projects are systematically tested yearly in the regional and national project specific and non-specific nurseries for adaptation, yield, nutritional value and cooking qualities, and reaction to major abiotic and biotic stresses. As stated earlier, these regional or national nurseries are CDBN, BRN, WMN, MRPN, and WRBT. Subsequently, the most promising breeding lines are tested in the state trials and in farmers' fields for identification, release, and commercialization of new cultivars. Because of inter-disciplinary, inter-institutional, multi-state collaboration improved cultivars and germplasm are increasingly joint releases. Thus, germplasm, research data, resources, and expertise are shared among all participants, and results discussed and work plans made during the annual W-150 meetings. Research procedures specific to a few projects will be briefly described below. Exotic Germplasm Conversion. Two approaches, namely "Pure Conversion" (PC) and "Trait Introgression Conversion" (TIC) will be used. In PC intended for complex traits, the ppd (and bush growth habit) allele from the elite adapted genotypes will be introgressed into the exotic tropical and sub-tropical recurrent genotypes, and selection for the target traits (see Appendix Table 2) reserved for later generations at multiple sites. In TIC intended for qualitative traits, the elite adapted genotype will be used as the recurrent parent with the target trait selection conducted in each generation. Additionally, alleles for growth habit and photoperiod insensitivity will be investigated using genetic and association mapping to initiate fine mapping, assessing allelic diversity at these loci, and develop tightly-linked markers. Abiotic Stresses. Heat resistant breeding lines will be developed from segregating populations using greenhouse and field studies. The greenhouse temperatures will be set at 32°C day and 28°C night 10 d prior to the earliest anthesis at Cornell University. Field screenings will be conducted during the high-temperature season at the Juana Diaz substation, University of Puerto Rico. Data will be recorded for yield, yield components, flower and young pod abscission, and seed and pod quality. Biotic Stresses. Common bacterial blight. Common blight samples will be obtained from all over the U.S. and the bacteria as well as the host cultivars characterized. For bacteria, morphological characteristics, brown pigment production, PCR- detection with the common bacterial blight primers, rep-PCR fingerprinting, and pathogenicity on selected Andean and Middle American beans will be used. Between 100 and 200 RIL will be developed from each of Othello/VAX 1, Othello/VAX 3, and Red Hawk/VAX 3 crosses. RIL will be evaluated for common bacterial blight in greenhouse using the multiple-needle inoculation method and/or in the field. Data will be used to find and tag new resistance QTL. Fusarium root rot and wilt. Breeding lines of different market classes from resistant x susceptible crosses will be screened in the greenhouse and field for Fusarium root rot and Fusarium wilt pathogen isolates from the Central High Plains and Michigan. Also, PCR-based marker techniques will be used. Promising breeding lines will be tested in adaptation nurseries and yield trials across the U.S. Geminiviruses. Efforts will be focused in three major areas: 1) biotic and molecular characterization of emerging bean-infecting begomoviruses, 2) application of P. vulgaris genomics, in relation to begomoviral pathogenesis and herbivory of phloem-feeding homopterans (Homoptera/Hemiptera), and 3) identification of molecular markers for BGYMV resistance genes. For characterization studies of geminiviruses causing curly top in the Western U.S., plants with suspected symptoms will be tested by PCR with a general curly top primer pair and a general DNA probe that detects bean-infecting geminiviruses. Positive samples will be further tested with curly top virus-specific primer pairs to identify the specific virus involved. Positive samples not detected with the specific primers will be further examined by DNA sequencing of PCR-amplified fragments. In terms of the application of genomics, efforts will be made to clone the dominant resistance gene for BDMV using a degenerate primer-heterologous transformation approach. For development of BGYMV resistance markers, the F2 plants and F2:3 families from Morales /BAT 93 and PR9771-3-1/BAT 93 populations will be evaluated for reaction to BGYMV. DNA will be extracted from the F2 plants, and plants found to be homozygous for resistance and susceptibility will be used to screen for potential RAPD markers for the resistance genes. Linkage between the candidate markers and the resistance genes will be determined based on the degree of recombination in the F2. A RAPD marker found to be tightly linked to either the bgm-2 or the Bgp-1 resistance genes would be converted to a SCAR. The two populations will also be used to map the bgm-1, bgm-2 and Bgp-1 resistance genes. Halo blight. Halo blight samples will be collected from various states and Psp races determined by inoculation of the host differentials. Races will be assessed for phaseolotoxin production by a bacterial growth inhibition assay and for the presence of the phaseolotoxin gene with gene-based PCR primers. Subsequently, they will be characterized by repetitive element PCR to assess whether Psp races have distinct DNA fingerprints when tested by the rep-PCR method. Approximately 100 RIL will be developed from each of Canadian Wonder (CW)/A43, CW/UI-3, CW/PI 150414, CW/CAL 143, and other crosses. These RIL will be evaluated for halo blight reactions in the greenhouse and under field conditions. Also, DNA from each RIL and parent will be extracted to find molecular markers linked with resistance alleles and QTL. Rust. New sources of Andean and Middle American beans with resistance to the broadest spectrum of rust pathogens will be identified. They will be crossed with susceptible genotypes to study the genetics of resistance, and to identify and tag resistance alleles. A new set of host differentials that includes six Andean and six Middle American beans, as well as molecular tools, including the elongation factor 1±, IGS, ITS, and others, will be used to determine pathogen diversity and its co-evolution with the common bean. White mold. Mycelial compatibility grouping, virulence, ribosomal DNA polymorphism, and microsatellites will be used to determine pathogen variation in the U.S. Potential resistant germplasm and breeding lines will be subjected to a multi-state greenhouse and field testing for identification of dependable white mold resistance. New resistance sources will be used to further improve existing white mold resistance. Previously available interspecific breeding lines will be evaluated for white mold in greenhouse and field, and new sets of breeding lines will be developed from pinto bean x P. coccineus crosses. Nutritional Value Dry beans of different market classes will be screened for in vitro antioxidant activity, total phenolic content, phenolic content by classes, major individual phenolic compounds, resistant starch, indigestible protein, total fiber, and Lunasin homologues (anticancer peptides). Antioxidant capacity of processed beans will be assessed since antioxidant activity is expected to be partially lost during heat treatment. Total antioxidant capacity will be measured using the ABTS assay, the Oxygen Radical Absorbance Capacity (ORAC) assay, and the FOX3 assay. Total phenolics will be estimated colorimetrically and the amount of phenolics in each class (e. g., benzoic acid derivatives, cinnamic acid derivatives, flavonoids) will be determined by spectral characteristics of phenolics separated by HPLC. Major phenolic compounds will be identified by mass spectrometry, NMR and IR. Beans from a diverse genetic background will be screened for Lunasin and its homologues. Lunasin positive extracts will be tested for cancer preventive properties. Since relatively little is known about the bioavailability of most plant antioxidants, in-vivo antioxidant activity will be assessed for different market classes possessing the greatest in-vitro antioxidant activity. A rat feeding study will assess glycemic effect [glycation of hemoglobin (Hb A1c)] and inflammatory response (C-reactive protein) in blood before and after induction of diabetes. It is hypothesized that beans possessing the greatest in-vivo antioxidant activity, the lowest glycemic index, and the greatest anti-inflammatory response will have cancer inhibitory activity compared to a control diet and to beans with low antioxidant and anti-inflammatory capacity. This hypothesis will be tested in a chemically induced mammary cancer experiment with rats. The potential for navy beans to mitigate the colon cancer enhancing properties of obesity, hyperglycemia, and hyperinsulinemia will be tested in a chemically induced colon cancer experiment in mice genetically predisposed to these conditions. All genetically improved beans will be canned to ensure that canning quality is maintained or improved. Extrusion will be used to prepare prototype snack items made from beans. The snack items will be promoted as healthy snacks with intent to move continued product development and marketing to the commercial sector. Gluten-free flour will be produced for people suffering from celiac disease , for vegetarians, and for health-conscious individuals desiring alternate food choices that will provide protein and fiber. Reduced or oligosaccharide free flour will be produced by enzymatic hydrolysis of the tri- and tetraoligosaccharides to produce products for individuals concerned with flatulence. The variability in indigestible starch and indigestible protein will be determined in the nursery maintained at East Lansing (MI) to estimate inheritance of these traits.Measurement of Progress and Results
Outputs
- Tropical and sub-tropical germplasm, possessing traits useful to the U.S. bean production, will be converted to temperate adaptation and released to the public. This germplasm will provide researchers novel genes to broaden the genetic base of the U.S. cultivars (e.g., new resistance genes to abiotic and biotic stresses). In addition, markers tightly linked to favorable alleles and QTL involved in domestication will be developed.
- Heat-resistant germplasm will be developed and used to breed new cultivars to increase and stabilize yields in high-temperature environments in the mainland U.S. and Puerto Rico.
- A detailed understanding of the extent and nature of genetic diversity of the pathogens causing economically important diseases such as curly top, bean golden mosaic, common and halo bacterial blights, Fusarium root rot and wilt, rust, and white mold in the U.S. will be obtained. Similarly, new alternative methods of detection, inoculation, and/or screening environments for geminiviruses and white mold will be developed. Together, this will help determine the most appropriate inoculation method and the number and type of strains/isolates that need to be used for resistance breeding. Also, the potential utility of PCR detection method (e.g., phaseolotoxin primers for the Psp strains) and of genetic fingerprinting (e.g., rep-PCR as a rapid method for identifying Psp races) for detecting and monitoring pathogen diversity in bacterial, fungal and viral pathogens of interest in the U.S. will be established. Host-pathogen differentials will be revised or developed for common and halo bacterial blights and rust. Improved knowledge regarding the significance and practical application of co-evolution of common bacterial blight and rust pathogens and common bean gene pools will be obtained. Finally, it is likely that new common bean R gene candidates will be identified by the degenerate primer-heterologous transformation approach. Because there is evidence that a diversity of such genes can be upregulated upon infection by a single pathogen, it is possible that a diversity of functional R genes could be cloned.
- The genetic base of resistance will be broadened; and new germplasm, improved breeding lines and cultivars of major market classes will be developed. The improved breeding lines and cultivars will possess high levels of resistance to curly top, bean golden mosaic, common and halo bacterial blights, Fusarium root rot and wilt, rust, and white mold; either singly or in various combinations, providing multiple-disease resistant cultivars. Concurrently, molecular markers for new resistance alleles and QTL for most of these diseases will be generated from this project.
- New information from laboratory and clinical studies will document the health benefits of eating dry beans and will be disseminated to consumers. Market classes of beans have comparatively higher bioactivity to reduce the incidence of cancer and diabetes will be identified and this should provide some insight into whether we can breed for greater nutritional value. Components of dry beans that impart health benefits will be identified. Value-added, bean-based snacks and convenience foods will be looked as a way to increase overall bean consumption.
Outcomes or Projected Impacts
- Improved high yielding bean cultivars resistant to multiple abiotic and biotic stresses (especially multiple-diseases) will contribute to dominate the regional and national production. Area planted to new cultivars may increase by more than 10% leading to substantial yield increases in the participating states.
- Commercial value of new cultivars may exceed $250 million annually.
- Adoption of multiple-disease resistant cultivars may reduce fungicide use by 25% or more resulting in savings to the producers and reduced use of pesticides, which will benefit the environment.
- Comparative health benefits (including specific components that impart health benefits) of eating different market classes of dry beans will be known to consumers.
- Availability of new value-added, bean-based snacks and convenience foods are expected to increase overall bean consumption in the U.S.
Milestones
(2006): To convert useful tropical germplasm adapted to the U.S. bean growing environments by 2010 the elite adapted x tropical crosses need to be made by 2006.(2007): To develop heat-resistant breeding lines and cultivars adapted to U.S. conditions by 2010, all genetic studies including the identification and tagging of heat-resistant alleles and QTL should be completed by 2007.
(2008): To determine genetic diversity in pathogens causing curly top, halo and common bacterial blights, rust, and white mold in the U.S. by 2010, most of the sampling of infected plants or seeds and isolations need to be completed by 2008. This will be followed by development or refinement of the host-pathogen differentials, genetic fingerprinting, and the PCR-based detection methods for monitoring and studying the significance of pathogen diversity for germplasm enhancement and development of cultivars.
(2009): To develop and release new cultivars and breeding lines resistant to curly top, bean golden mosaic, common and halo bacterial blights, Fusarium root rot and wilt, rust, and/or white mold by 2010, identification of new sources of resistance, inheritance of resistance, including identification and tagging of resistance alleles and QTL, needs to be completed by 2009. Also by 2009, most of crossing or population developments, and early generation selections should be accomplished; and state, regional and national testing of new breeding lines initiated.
(2009): To enhance consumer acceptance of beans and promote new bean products by 2010, the laboratory, preclinical, and clinical trials needed for the identification of health benefits associated with beans and the bean constituents responsible for these benefits need to be finished, and necessary food processing technologies made available by 2009.
Projected Participation
View Appendix E: ParticipationOutreach Plan
Research results from each sub-project will be promptly published in refereed and non-refereed journals, extension bulletins, flyers, etc., and posted on the web sites of individual institutions or programs.
Breeding lines and cultivars will be extensively tested statewide, regionally (e.g., the Midwest Regional Performance Nursery and Western Regional Bean Trials), and nationally (e.g., the Cooperative Dry Bean Nursery), including on-farm strip-plantings of the most promising or outstanding genotypes in different cropping systems.
Breeder, Foundation, Registered, and Certified seed of the new cultivars will be produced and distributed to bean growers and the seed industry.
Field days will be held each year at or near crop maturity. In addition, the most important findings will be shared with all interested parties through workshops, news media, and electronic mail.
The released cultivars and germplasm lines will be registered with the Crop Science Society of America, Bean Improvement Cooperative, or American Society of Horticultural Sciences, and seed deposited with the National Center for Genetic Resources Preservation.
Organization/Governance
Present officers of the W-150 Regional Project are: Chair, Shree P. Singh, University of Idaho, Kimberly, ID. Vice-Chair, Phillip Griffiths, Cornell University, Geneva, NY. Secretary, Timothy Porch, USDA-ARS, Tropical Agriculture Research Service, Mayaguez, PR.
The directors of the various participating state institutions designate the
W-150 participating researchers, who in-turn elect the Members or officers of the Technical Committee. The project is considered a Western Regional Research Project, but has always had substantial participation by states in other bean producing regions of the U.S. and USDA-ARS researchers. The Technical Committee officers are a Chairperson, Vice-Chairperson, and Secretary. Unless he/she declines to serve, the Vice-Chairperson will succeed the Chairperson. The Secretary is elected annually and the previous Secretary will succeed the Vice-Chairperson, unless he/she declines to serve. An election will be held if any officer declines to serve in his/her office. The officers will be elected from the officially designated representatives. The Western Association of Agricultural Experiment Station Directors select the Administrative Advisor who has no voting rights.
The Technical Committee will meet annually, unless otherwise planned, at a place and on a date designated by a majority vote of the committee. Minutes will be recorded and an annual progress report will be prepared by the Technical Committee and submitted through proper channels.
Literature Cited
Acevedo Román, M., A. Molina Castañeda, J.C. Angel Sánchez, C.G. Muñoz, and J.S. Beaver. 2004. Inheritance of normal pod development in bean golden yellow mosaic resistant common bean. J. Am. Soc. Hort. Sci. 129:549-552.
Andersen, J.W., L. Story, B. Sieling, W-J.L. Chen, M.S. Petro, and J. Story. 1984. Hypocholesterolemic effects of oat-bran or bean intake for hypercholesterolemic men. Am. J. Clinical Nutri. 40:1146-1155.
Anderson, J.W. 1987. Dietary fiber, lipids and atherosclerosis. Am. J. Cardiol. 60: G17-G22.
Anderson, J.W., N.J. Gustafson, D.B. Spencer, J. Tietyen, and C.A. Bryant. 1990. Serum-lipid response of hypercholesterolemic men to single and divided doses of canned beans. Am. J. Clinical Nutri. 51: 1013-1019.
Anderson, J.W., B.M. Smith, and C.S. Washnock. 1999. Cardiovascular and renal benefits of dry bean and soybean intake. Am. J. Clinical Nutri. 70: 464S-474S.
Ariyarathne, H.M., D.P. Coyne, G. Jung., P.W. Skroch, A.K. Vidaver, J.R. Steadman, P.N. Miklas, and M.J. Bassett. 1999. Molecular mapping of disease resistance genes for halo blight, common bacterial blight, and bean common mosaic virus in a segregating population of common bean. J. Am. Soc. Hort. Sci. 124:654-62.
Bazzano, L.A., J. He, L. Ogden, G. Loria, C. Vupputuri, S. Myers, L. and P. K. Whelton. 2001. Legume consumption and risk of coronary heart disease in US men and women. Archives of Internal Medicine 161: 2573-2578.
Beaver, J.S, J.C. Rosas, J. Myers, J. Acosta, J.D. Kelly, S. Nchimbi-Msolla, R. Misangu, J. Bokosi, S. Temple, E. Arnaud-Santana, and D.P. Coyne. 2003. Contributions of the bean/cowpea CRSP to cultivar and germplasm development in common bean. Field Crops Res. 82:87-102.
Beaver J.S., and J.R. Steadman. 1999. Adelantos en el mejoramiento de frijol Andino Caribeno. Agron. Mesoam. 10:77-82.
Beaver, J.S., M. Zapata, and P.N. Miklas. 1999. Registration of PR9443-4 dry bean germplasm resistant to bean golden mosaic, common bacterial blight, and rust. Crop Sci. 39:1262.
Beninger, C.W., and G.L. Hosfield. 2003. Antioxidant activity of extracts, condensed tannin fractions, and pure flavonoids from Phaseolus vulgaris L. seed coat color genotypes. J. Agric. Fd. Cem. 51:7879-7883.
Berrios, J.D., B.G. Swanson, and W.A. Cheong. 1999. Physico chemical characterization of stored black beans (Phaseolus vulgaris L.). Food Res. Int. 32:669-676.
Bingwen, L., W. Zhaofeng, L. Wanzhen, and Z. Rongjue. 1981. Effects of bean meal on serum cholesterol and triglycerides. Chinese Medical J. 94: 455-458.
Blair, M.W., M.J. Bassett, A.M. Abouzid, E. Hiebert, J.E. Polston, R.T. McMillan, W. Graves, and M. Lamberts. 1995. Occurrence of bean golden mosaic virus in Florida. Plant Dis. 79:529-533.
Broughton, W.J., G. Hernandez, M. Blair, S. Beebe, P. Gepts, and J. Vanderleyden. 2003. Beans (Phaseolus spp.)- model food legumes. Plant and Soil 252:55-128.
Brown, J.K., K.M. Ostrow, A.M. Idris, and D.C. Stenger. 1999. Biotic, molecular and phylogenetic characterization of bean calico mosaic virus, a distinct /geminivirus/ species with affiliation in the squash leaf curl virus cluster. Phytopathology 89: 273-280.
Burke, D.W. 1982a. Registration of red Mexican beans Rufus, NW-59, and NW-63. Crop Sci. 22:685-686.
Burke, D.W. 1982b. Registration of pinto beans NW-410 and NW-590. Crop Sci. 22:684-685.
Burke, D.W. 1982c. Registration of pink beans Viva, Roza, and Gloria. Crop Sci. 22:684.
Central America. Annu. Rpt. Bean Improv. Coop. 45:166-167.
Correa, P. 1981. Epidemiological correlations between diet and cancer frequency. Cancer Res. 41: 3685-3689.
Corrêa, R.X., M.R. Costa, P.I. Good-God, V.A. Ragagnin, F.G. Faleiro, M.A. Moreira, and E.G. de Barros. 2000. Sequence characterized amplified regions linked to rust resistance genes in the common bean. Crop Sci. 40:804-807.
Coyne, D.P., D.S. Nuland, D.T. Lindgren, J.R. Steadman, D.W. Smith, J. Gonzales, J. Schild, J. Reiser, L. Sutton, C. Carlson, J.R. Stavely, and P.N. Miklas. 2000. 'Weihing' great northern disease resistant dry bean. HortScience 35:310-312.
Cramer, R.A., P.F. Byrne, M.A. Brick, L. Panella, E. Wickliffe, and H.F. Schwartz. 2003. Characterization of Fusarium oxysporum isolates from common bean and sugar beet using pathogenicity assays and random-amplified polymorphic DNA markers. J. Phytopathology 151:352-306.
Cross, H., M.A. Brick, H.F. Schwartz, L.W. Panella, and P.F. Byrne. 2000. Inheritance of resistance to Fusarium wilt in two common bean races. Crop Sci. 40:954-958.
Ender, M. 2003. QTL analysis of genetic resistance to white mold (Sclerotinia sclerotiorum) in common bean (Phaseolus vulgaris). Ph.D. diss., Michigan State University, East Lansing MI. 166pp.
Fall, A. L., P.F. Byrne, G. Jung, D.P. Coyne, M.A. Brick, and H.F. Schwartz. 2001. Detection and mapping of a major locus for Fusarium wilt resistance in common bean. Crop Sci. 41:1494-1498.
Foster-Powell, K., and J.B. Miller. 1995. International tables of glycemic index. Am. J.Clinical Nutri. 62: S871-S890.
Fourie, D., P.N. Miklas, and H.M. Ariyarathne. 2004. Genes conditioning halo blight resistance to races 1, 7, and 9 occur in a tight cluster. Annu. Rpt. Bean Improve. Coop. 47:103-104.
Freytag G.F., M.J. Bassett, and M. Zapata. 1982. Registration of XR-235-1-1 bean germplasm. Crop Sci. 22:1268-69.
Freytag, G.F., J.D. Kelly, M.W. Adams, J. Lopez Rosa, J. Beaver, and R. Echavez Badel. 1985. Registration of two navy bean breeding lines, L226-10 and L227-1. Crop Sci. 25:714.
Fung, T.T., W.C. Willett, M.J. Stampfer, J.E. Manson, and F.B. Hu. 2001. Dietary patterns and the risk of coronary heart disease in women. Arch. Int. Medic. 161:1857-1862.
Garrido-Ramirez, E.R., M.R. Sudarshana, and Gilbertson, R.L. 2000. Bean golden mosaic virus from Chiapas, Mexico: characterization, pseudorecombination with other bean-infecting geminiviruses and germplasm screening. Phytopathology 90:1224-1232.
Gepts, P., and D.G. Debouck. 1991. Origin, domestication, and evolution of the common bean, Phaseolus vulgaris, p. 7-53, In O. Voysest and A. Van Schoonhoven (eds.) Common beans: Research for crop improvement. C.A.B. Intl., Wallingford, UK and CIAT, Cali, Colombia.
Gepts, P., T.C. Osborn, K. Rashka, and F.A. Bliss. 1986. Phaseolin protein variability in wild forms and landraces of the common bean (Phaseolus vulgaris): evidence for multiple centers of domestication. Econ. Bot. 40:451-468.
Gilmore, B., J.R. Myers, and D. Kean. 2002. Completion of testing of Phaseolus coccineus plant introductions (PIs) for white mold, Sclerotinia sclerotiorum, resistance. Annu. Rpt. Bean Improv. Coop. 45:64-65.
Grafton, K.F., J.B. Rasmussen, J.R. Steadman, D.C. Hauf, and C. Donohue. 2002. Potential new sources of resistance to white mold in the Phaseolus core collections. Annu. Rpt. Bean Improv. Coop. 45: 58-59.
Gross, Y., and J. Kigel. 1994. Differential sensitivity to high temperature of stages in the reproductive development of common bean (Phaseolus vulgaris L.). Field Crops Res. 36:201-212.
Gu, W., J. Zhu, D. Wallace, S. Singh, and N. Weeden. 1998. Analysis of genes controlling photoperiod sensitivity in common bean using DNA markers. Euphytica 102:125-132.
Haley, S.D., L.K. Afanador, P.N. Miklas, J.R. Stavely, and J.D. Kelly. 1994. Heterogeneous inbred populations are useful as sources of near-isogenic lines for RAPD marker localization. Theor. Appl. Genet. 88:337-342.
Haley, S.D., P.N. Miklas, J.R. Stavely, J. Byrum, and J.D. Kelly. 1993. Identification of RAPD markers linked to a major rust resistance gene block in common bean. Theor. Appl. Genet. 86:505-512.
Hang, A.N., M.J. Silbernagel, P.N. Miklas and G.L. Hosfield. 2000. Registration of LeBaron small red dry bean. Crop Sci. 40:853-854.
Hangen, L.A., and M.R. Bennink. 2003. Consumption of black beans and navy beans (Phaseolus vulgaris) reduced axozymethane-induced colon cancer in rats. Nutri. Cancer 44:60-65.
Harland, B.F., and E.R. Morris. 1995. Phytate - a good or a bad food component. Nutri. Res. 15:733-754.
Hosfield, G.L., G.V. Varner, M.A. Uebersax, and J.D. Kelly. 2004. Registration of Merlot small red bean. Crop Sci. 44:351-352.
Hu, F.B., E.B. Rimm, M.J. Stampfer, A. Ascherio, D. Spiegelman, and W.C. Willett. 2000. Prospective study of major dietary patterns and risk of coronary heart disease in men. Am. J. Clinical Nutri. 72: 912-921.
Hughes, J.S., C. Ganthavorn, and S. Wilson-Sanders. 1997. Dry beans inhibit azoxymethane-induced colon carcinogenesis in F344 rats. J. Nutri. 127: 2328-2333.
Idris, A.M., E. Hiebert, J. Bird, and J.K. Brown. 2003. Two newly-described begomoviruses of Macroptillium lathyroides and common bean. Phytopathology 93:774-783.
Johnson, E., P.N. Miklas, J.R. Stavely, and J.C. Martinez-Cruzado. 1995. Coupling-and repulsion-phase RAPDs for marker assisted selection of PI 181996 rust resistance in common bean. Theor. Appl. Genet. 90:659-664.
Jung, G., S. Beebe, J. Nienhuis, S. Park, D.P. Coyne, J. Marita, F. Pedraza, and J. Tohme. 1998a. Development of SCAR markers linked to common bacterial blight resistance genes (QTL) in common bean. Annu. Rpt. Bean Improv. Coop. 41: 97-98.
Jung, G., D.P. Coyne, J.M. Bokosi, J.R. Steadman, J. Nienhuis. 1998b. Mapping genes for specific and adult plant resistance to rust and abaxial leaf pubescence and their genetic relationship using random amplified polymorphic DNA (RAPD) markers in common bean. J. Am. Soc. Hort. Sci. 123: 859-863.
Jung, G., D.P. Coyne, P. Skroch, J. Nienhuis, E. Arnaud-Santana, J. Bokosi, H. Ariyarathne, J. Steadman, J. Beaver, and S. Kaeppler. 1996. Molecular markers associated with plant architecture and resistance to common blight, web blight, and rust in common beans. J. Am. Soc. Hort. Sci. 121:794-803.
Jung, G., P. Skroch, D.P. Coyne, J. Nienhuis, H. Ariyarathne, S. Kaeppler, and M. Bassett. 1997. Molecular-marker-based genetic analysis of tepary-bean-derived common bacterial blight resistance in different developmental stages of common bean. J. Am. Soc. Hort. Sci. 122: 329-337.
Kalavacharla, V., J.R. Stavely, J.R. Myers, and P.E. McClean. 2000. Crg, a gene required for Ur-3-mediated rust resistance in common bean, maps to a resistance gene analog cluster. Mol. Pl. Microb. Int. 13:1237-1242.
Kelly, J.D., M.W. Adams, A.W. Saettler, G.L. Hosfield, G.V. Varner, J.S. Beaver, and M.A. Uebersax. 1989. Registration of Mayflower navy bean. Crop Sci. 29:1571.
Kelly, J.D., M.W. Adams, A.W. Saettler, G.L. Hosfield, G.V. Varner, M.A. Uebersax, and J. Taylor. 1990. Registration of 'Sierra' pinto bean. Crop Sci. 30:745-746.
Kelly, J.D., P. Gepts, P.N. Miklas, and D.P. Coyne. 2003. Tagging and mapping genes and QTL and molecular marker-assisted selection for traits of economic importance in bean and cowpea. Field Crops Res. 82:135-154.
Kelly, J.D., G.L. Hosfield, G.V. Varner, M.A. Uebersax, P.N. Miklas, and J. Taylor. 1992. Registration of 'Alpine' great northern bean. Crop. Sci. 32:1509-1510.
Kelly, J.D., G.L. Hosfield, G.V. Varner, M.A. Uebersax, and J. Taylor. 1999. Registration of 'Matterhorn' great northern bean. Crop Sci. 39:589-590. Kelly, J. D., J.M. Kolkman, and K. Schneider. 1998. Breeding for yield in dry bean (Phaseolus vulgaris L.). Euphytica 102:343-356.
Kelly, J.D., and P.N. Miklas. 2004. Germplasm enhancement in the United States: the tropical connection. Annu. Rpt. Bean Improv. Coop. 47:35-36.
Kelly, J.D., and V.A. Vallejo. 2004. A comprehensive review of the major genes conditioning resistance to anthracnose in common bean. HortScience 39:1196-1207.
Kennedy, A.R. 1994. Prevention of carcinogenesis by protease inhibitors. Cancer Res. 54:S1999-S2005.
Koenig, R., S.P. Singh, and P. Gepts. 1990. Novel phaseolin types in wild and cultivated common bean (Phaseolus vulgaris, Fabaceae). Econ. Bot. 44: 50-60.
Koinange, E.M.K., S.P. Singh, and P. Gepts. 1996. Genetic control of the domestication syndrome in common-bean. Crop Sci. 36:1037-1045.
Kolkman, J.M., and J.D. Kelly. 2003. QTL conferring resistance and avoidance to white mold (Sclerotinia sclerotiorum) in common bean (Phaseolus vulgaris). Crop Sci. 43:539-548.
Konsens, I., M. Ofir, and J. Kigel. 1991. The effect of temperature on the production and abscission of flowers and pods in snap bean (Phaseolus vulgaris L.). Ann. Bot. 67:391-399.
Koratkar, R., and A.V. Rao. 1997. Effect of soyabean saponins on azoxymethane-induced preneoplastic lesions in the colon of mice. Nutri. Cancer Int. J. 27: 206-209.
Larson, R.C., and P.N. Miklas. 2004. Generation and molecular mapping of a sequence characterized amplified region marker linked to the Bct gene for resistance to Beet curly top virus in common bean. Phytopathology 94:320-325.
McClean, P.E., J.R. Myers, and J.J. Hammond. 1993. Coefficient of parentage and cluster analysis of North American dry bean cultivars. Crop Sci. 33:190-97.
Meine, C.M.S., R. Naidoo, and M.M. Liebenberg. 2004. Conversion of the RAPD marker for Ur-4 to a co-dominant SCAR marker SA141079/800. Annu. Rpt. Bean Improv. Coop. 47:261-262.
Melotto, M., and J.D. Kelly. 1998. SCAR markers linked to major disease resistance genes in common bean. Annu. Rpt. Bean Improv. Coop. 41:64-65.
Miklas, P.N. 2000. Use of Phaseolus germplasm in breeding pinto, great northern, pink, and red bean for the Pacific Northwest and intermountain region. p. 13-29. In S.P. Singh (ed.) Bean research, production and utilization. Proc. of the Idaho bean workshop. Univ. of Idaho, Moscow, ID.
Miklas, P.N., R. Delorme, and R. Riley. 2003. Identification of QTL conditioning resistance to white mold in a snap bean population. J. Am. Soc. Hort. Sci. 128:564-270.
Miklas, P.N., D.C. Hauf, R.A. Henson, and K.F. Grafton. 2004. Inheritance of ICA Bunsi-derived resistance to white mold in a navy x pinto bean cross. Crop Sci. 44:1584-1588.
Miklas, P.N., W.C. Johnson, R. Delorme, R.H. Riley, and P. Gepts. 2001a. QTL conditioning physiological resistance and avoidance to white mold in dry bean. Crop Sci. 41:309-315.
Miklas, P.N., J.R. Smith, K.F. Grafton, D.P. Coyne, and M.A. Brick. 2001b. Release of pinto and great northern bean germplasm lines USPT-CBB-1, USPT-CBB-2, USPT-CBB-3, and USGN-CBB-4 with resistance to common bacterial blight, rust, and mosaic. Annu. Rpt. Bean Improv. Coop. 44:183-185.
Miklas, P.N., J.R. Smith, A.N. Hang, K.F. Grafton, and J.D. Kelly. 2001c. Release of navy and black bean germplasm lines USNA-CBB-1, USNA-CBB-2, USNA-CBB-3, USNA-CBB-4, and USBK-CBB-5 with resistance to common bacterial blight. Annu. Rpt. Bean Improv. Coop. 44:181-182.
Miklas, P.N., J.R. Smith, R. Riley, K.F. Grafton, S.P Singh, G. Jung, and D.P. Coyne. 2000a. Marker-assisted breeding for pyramided resistance to common bacterial blight in common bean. Annu. Rpt. Bean Improv. Coop. 43:39-40.
Miklas, P.N., J.R. Stavely, and J.D. Kelly. 1993. Identification and potential use of a molecular marker for rust resistance in common bean. Theor. Appl. Genet. 85:745-749.
Miklas, P.N., V. Stone, M.J. Daley, J.B. Stavely, J.R. Steadman, M.J. Bassett, R. Delorme, and J.S. Beaver. 2000b. Bacterial, fungal, and viral disease resistance loci mapped in a recombinant inbred common bean population (Dorado/XAN176). J. Am. Soc. Hort. Sci. 125:476-481.
Miklas, P.N., M. Zapata, J.S. Beaver, and K.F. Grafton 1994. Registration of four dry bean germplasm resistant to common bacterial blight: ICB-3, ICB-6, ICB-8, and ICB-10. Crop Sci. 39:594.
Mkandawire, A.B.C., R.B. Mabagala, P. Guzman, P. Gepts, and R.L. Gilbertson. 2004. Genetic diversity and pathogenic variation of common blight bacteria (Xanthomonas campestris pv. phaseoli and X. campestris pv. phaseoli var. fuscans) suggests coevolution with the common bean. Phytopathology 94:593-603.
Monterroso, V.A., and H.C. Wien. 1990. Flower and pod abscission due to heat stress in beans. J. Am. Soc. Hort. Sci. 115:631-634.
Nemchinova, Y.P., and J.R. Stavely. 1998. Development of SCAR primers for the Ur-3 rust resistance gene in common bean. Phytopathology 88:S67.
Osorno, J.M., J.S. Beaver, F. Ferwerda, and P.N. Miklas. 2003. Two genes from Phaseolus coccineus L. confer resistance to bean golden yellow mosaic virus. Annu. Rpt. Bean Improv. Coop. 46:147-148.
Park, S.O., D.P. Coyne, A. Dursun, and G. Jung. 1998. Identifying randomly amplified polymorphic DNA (RAPD) markers linked to major genes for common bacterial blight resistance in tepary bean. J. Am. Soc. Hort. Sci. 123:278 282.
Park, S.O., D.P. Coyne, and J.R. Steadman. 2004a. Development of a SCAR marker linked to the Ur-7 gene in common bean. Annu. Rpt. Bean Improv. Coop. 47:269-270.
Park, S.O., D.P. Coyne, J.R. Steadman, K.M. Crosby, and M.A. Brick. 2004b. RAPD and SCAR markers linked to the Ur-6 Andean gene controlling specific rust resistance in common bean. Crop Sci. 44:1799-1807.
Park, S.O., D.P. Coyne, J.R. Steadman, and P.W. Skroch. 2001. Mapping of QTL for resistance to white mold diseases in common bean. Crop Sci. 41:1253-1262.
Park, S.O., D.P. Coyne, J.R. Steadman, and P.W. Skroch. 2003. Mapping of the Ur-7 gene for specific resistance to rust in common bean. Crop Sci. 43:1470-1476.
Pastor-Corrales, M.A. 2003. Sources, genes for resistance and pedigrees of 52 rust and mosaic resistant dry bean germplasm lines released by USDA Beltsville bean project in collaboration with the Michigan, Nebraska, North Dakota Agricultural Experiment Stations. Annu. Rpt. Bean Improv. Coop. 46:235-241.
Pastor-Corrales, M.A., and J.R. Stavely. 2001. New bean germplasm lines with multiple genes for rust and mosaic resistance. Phytopathology 91: S70.
Pedraza, F., G. Gallego, S. Beebe, and J. Tohme. 1997. Marcadores SCAR y RAPD para la resistencia a la bacteriosis comun (CBB). p. 130-134. In S.P. Singh and O. Voysest (eds.) Taller de mejoramiento de frijol para el siglo XXI: Bases para una estrategia para America Latina. CIAT, Cali, Colombia.
Porch, T.G. 2001. Genetics and applications of heat tolerance in common bean. PhD Diss. Cornell Univ. N.Y.
Posa Macalincag, M.C.T., G.L. Hosfield, K.F. Grafton, M.A. Uebersax, and J.D. Kelly. 2002. Quantitative trait loci (QTL) analysis of canning quality traits in kidney bean (Phaseolus vulgaris L.). J. Am. Soc. Hort. Sci. 127:608-615.
Queiroz, V.T., C.S. Sousa, T.L.P.O. Souza, M.R. Costa, D.A. Sanglad, V.A. Ragagnin, E.G. Barros, and M.A. Moreira. 2004. SCAR marker linked to the common bean rust resistance gene Ur-11. Annu. Rpt. Bean Improv. Coop. 47:271-272.
Rainey, K.M., and P.D. Griffiths. 2004. Identification of heat tolerant Phaseolus acutifolius A. Gray plant introductions following exposure to high temperatures in a controlled environment. Gen. Res. Crop. Evol.(In press).
Rainey, K.M., and P.D. Griffiths. 2005. Differential responses of common bean genotypes to high temperatures. J. Am. Soc. Hort. Sci. 130:(In press).
Schneider, K., M. Brothers, and J.D. Kelly. 1997. Marker-assisted selection to improve drought resistance in common bean. Crop Sci. 37: 51-60.
Schneider, K.A., K.F. Grafton, and J.D. Kelly. 2001. Genetic and QTL analysis of resistance to Fusarium root rot in bean. Crop Sci. 41:535-542.
Schoonhoven, A. van, C. Cardona, and J. Valor. 1983. Resistance to the bean weevil and the Mexican bean weevil (Coleoptera:Bruchidae) in noncultivated common bean accessions. J. Econ. Entomol. 76:1255-1259.
Schwartz, H.F., K. Otto, H. Terán, and S.P. Singh. 2004. Inheritance of white mold resistance in the interspecific crosses of pinto cultivars Othello and UI 320 and Phaseolus coccineus L. accessions PI 433246 and PI 439534. Annu. Rpt. Bean Improv. Coop. 47:279-280.
Seo, Y.-S., P. Gepts, and R.L. Gilbertson. 2004. Genetics of resistance to the geminivirus, Bean dwarf mosaic virus, and the role of the hypersensitive response in common bean. Theor. Appl. Genet. 108:786-793.
Shonnard, G.C., and P. Gepts. 1994. Genetics of heat tolerance during reproductive development in common bean. Crop Sci. 34: 1168-1175.
Shutler, S.M., G.M. Bircher, J.A. Tredger, L.M. Morgan, A.F. Walker, and A.G. Low. 1989. The effect of daily baked bean (Phaseolus vulgaris) consumption on the plasma-lipid levels of young, normo-cholesterolemic men. British J. Nutrition 61: 257-265.
Silbernagel M.J., and R.M. Hannan. 1992. Use of plant introductions to develop U.S. bean cultivars. p 1-8. In H. Shands and L.E. Weisner (eds.) Use of plant introductions in cultivar development. Part 2. CSSA Spec. Publ. 20. CSSA, Madison, WI.
Simpson, H.C.R., S. Lousley, M. Geekie, R.W. Simpson, R.D. Carter, T.D.R. Hockaday, and J.I. Mann. 1981. A high-carbohydrate leguminous fiber diet improves all aspects of diabetic control. Lancet 1: 1-4.
Singh, S.P. 1995. Selection for water stress tolerance in interracial populations of common bean. Crop Sci. 35:118-124.
Singh, S.P. 2001. Broadening the genetic base of common bean cultivars: a review. Crop Sci. 41:1659-1675.
Singh, S.P., P. Gepts, and D.G. Debouck. 1991. Races of common bean (Phaseolus vulgaris, Fabaceae). Econ. Bot. 45:379-396.
Singh, S.P., and C.G. Muñoz. 1999. Resistance to common bacterial blight among Phaseolus species and common bean improvement. Crop Sci. 39:80-89.
Singh, S.P., C.G. Muñoz, and H. Terán. 2001. Registration of common bacterial blight resistant dry bean germplasm VAX 1, VAX 3, and VAX 4. Crop Sci. 41:275-276.
Smith, J.R., S.J. Park, P.N. Miklas, and C.H. Canaday. 2003. Release of inter-racial dry bean germplasm line TARS-PT03-1 with yield potential and resistance to soil pathogenic fungi and common bacterial blight. USDA-ARS Germplasm Release Notice, 2 p.
Sonnante, G., T. Stockton, R.O. Nodari, V.L. Becerra Velásquez, and P. Gepts. 1994. Evolution of genetic diversity during the domestication of common-bean (Phaseolus vulgaris L.). Theor. Appl. Genet. 89:629-635.
Soto, M., and R.L. Gilbertson. 2002. Distribution and rate of movement of the curtovirus /Beet mild curly top virus /(Family/ Geminiviridae/) in the beet leafhopper. Phytopathology 93:478-484.
Stavely, J.R. 1984. Genetics of resistance to Uromyces phaseoli in a Phaseolus vulgaris line resistant to most races of the pathogen. Phytopathology 74:339-344.
Stavely, J.R., and K.F.Grafton 1985. Genetics of resistance to 8 races of Uromyces appendiculatus in Phaseolus vulgaris cultivar Mexico 235. Phytopathology 75:1310 (Abstr.).
Stavely, J.R., K.F.Grafton, and J.D. Kelly. 1998. Release of BelDakMi-RMR-14 erect, short vine, rust, and mosaic resistant pinto bean germplasm line. USDA-ARS germplasm release notice, Beltsville, MD.
Stavely, J.R., K.F.Grafton, and J.D. Kelly. 1999. Release of BelDakMi-RMR-15, -16, -17, and -18 erect, short vine, rust and mosaic resistant pinto bean germplasm lines. USDA-ARS germplasm release notice, Beltsville, MD.
Stavely, J.R., J.D. Kelly, and K.F. Grafton 1992. Release of four erect, short-vine pinto bean germplasm lines, BelDakMi-rust resistant-1, -2, -3, and -4.0 U.S.D.A., North Dakota and Michigan Agric. Exper. Sta. Germplasm Release Notice. 3p.
Stavely, J.R., J.D. Kelly, and K.F. Grafton. 1994. BelMiDak-rust-resistant navy dry bean germplasm lines. HortScience 29:709-911.
Stavely, J.R., and M.J. Silbernagel. 1993. Simultaneous screening for reactions to rust and bean common mosaic virus. Annu. Rpt. Bean Improv. Coop. 36:164-165.
Steadman, J.R., K. Eskridge, J. Costs, K. Grafton, J. Kelly, K. Kriecek, J. Kolkman, J. Myers, and P. Miklas. 2001. Evaluation of sources of resistance to Sclerotinia sclerotiorium in common bean with five methods at multiple locations. Annu. Rpt. Bean Improv. Coop. 44:89-90.
Steadman, J.R., K.M. Eskridge, K. Powers, C. Kurowski, R. Mainz, J. Kelly, P. Griffiths, K. Grafton, J. Myers, P. Miklas, and K. Kmiecik. 2003.
Identification of partial resistance to Sclerotinia sclerotiorum in field and greenhouse tests at multiple locations. Annu. Rpt. Bean Improv. Coop. 46:225-226.
Steadman, J.R., J.M. Kolkman, and K.M. Eskridge. 2002. Screening for and identifying sources of resistance to Sclerotinia sclerotiorum in common bean. Annu. Rpt. Bean Improv. Coop. 45:48-49.
Steadman, J.R., L.K. Otto-Hanson, K. Powers, C. Kurowski, R. Mainz, J. Kelly, P. Griffiths, K. Grafton, J. Myers, P. Miklas, and H. Schwartz. 2004. Identification of partial resistance to Sclerotinia sclerotiorum in common bean at multiple locations. Annu. Rpt. Bean Improv. Coop. 47:281-282.
Taylor, J.D., D.M. Teverson, and J.H.C. Davis. 1996b. Sources of resistance to Pseudomonas syringae pv. phaseolicola races in Phaseolus vulgaris. Plant Pathology 45: 479-485.
Taylor, J.D., D.M. Teverson, M.A. Allen, and M.A. Pastor-Corrales. 1996a. Identification and origin of races of Pseudomonas syringae pv. phaseolicola from Africa and other bean growing areas. Plant Pathology 45:469-478.
Teverson, D.M. 1991. Genetics of pathogenicity and resistance in the halo-blight disease of beans in Africa. Ph.D. Dissertation. Univ of Birmingham, UK. 205p.
Thomas, C.V., R.M. Manshardt, and J.G. Waines. 1983. Teparies as a source of useful traits for improving common beans (Phaseolus acutifolius hybridization with Phaseolus vulgaris). Desert Plants 5:43.48.
Tietyen-Clark, J. 1986. The benefits of bean. p. 1. In J.W. Andersen (ed.) Diabetes and Nutrition News. HCF Diabetes Foundation, Lexington, U.S.A.
Velasquez V., R., and H.F. Schwartz. 2000. Resistance of two bean lines to wilt by Fusarium oxysporum f. sp. phaseoli under different soil temperatures. Agro-Ciencia 16:81-86. [in Spanish]
Velez, J., M.J. Bassett, J.S. Beaver, and A. Molina. 1998. Inheritance of resistance to bean golden mosaic virus in common bean. J. Am. Soc. Hort. Sci. 123:628-631.
White, J.W., and S.P. Singh. 1991. Breeding for adaptation to drought. p. 501-560. In A. van Schoonhoven and O. Voysest (eds.) Common beans: Research for crop improvement. C.A.B. Int., Wallingford, U.K. and CIAT, Cali, Colombia.
Yu, K., S.J. Park, and V. Poysa. 2000. Marker-assisted selection of common beans for resistance to common bacterial blight: efficacy and economics. Plant Breed. 119:411-415.
Zapata, M., and J.S. Beaver. 2002. Common bacterial blight studies in the Caribbean and Central America. Annu. Rpt. Bean Improv. Coop. 45:166-167.