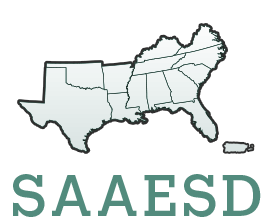
S1028: Ecological and genetic diversity of soilborne pathogens and indigenous microflora
(Multistate Research Project)
Status: Inactive/Terminating
S1028: Ecological and genetic diversity of soilborne pathogens and indigenous microflora
Duration: 10/01/2006 to 09/30/2012
Administrative Advisor(s):
NIFA Reps:
Non-Technical Summary
Statement of Issues and Justification
This project would apply to SAAESD Priority Areas under Goals 1A (integrated and sustainable agricultural production systems), 4B (natural resource and ecosystem management), and 4F (IPM systems, including biologically-based tactics). Soilborne pathogens are a diverse group of pathogens that reduce plant emergence and infect roots and crowns. The result is reduced plant productivity, increased costs to the grower and potential ecological damage to the adjacent natural environment. Below is a summary of current problems growers face due to soilborne pathogens in four specific plant production systems direct-seeded cotton and soybean, ornamental bedding plants destined for use in the landscape, and vegetable transplants for field production.Cotton seedling diseases are a major factor affecting cotton production worldwide (DeVay, 2001; Hillocks, 1992; Melero-Vara and Jimenaz-Diaz, 1990; Howell, 2001; Howell, 2002). Loss estimates for seedling diseases accounted for 27% of the total estimated losses in lint production from 1991-2000 (DeVay, 2001). The primary pathogens of the cotton seedling disease complex are Rhizoctonia solani, Thielaviopsis basicola, Pythium spp., and Fusarium spp. In addition to stand losses due to damping-off disease, both pre and post-emergence, seedling diseases may delay early season crop growth and result in additional management problems. In severe disease situations replanting may be required. Cotton seed in the U.S. is universally treated with fungicides prior to sale, indicating the severity of seedling disease problems and the efficacy of these fungicides. The producer must decide whether or not to use additional fungicides either on the seed before planting (planter-box, hopper-box, or custom seed treatments) or in the planting furrow (in-furrow treatments). These practices should give greater protection to emerging plants and have been reported to be effective in controlling seedling disease (Chambers, 1995; Colyer et al., 1991; Colyer and Vernon, 2005; Minton and Garber, 1983, Minton et al., 1982). Costs for hopper-box fungicides or custom seed treatments may range from $4.15 to $11.12 per hectare ($1.68 to $4.50 per acre), while in-furrow fungicides may cost the grower from $10.75 to $54.36 per hectare ($4.35 and $22.00 per acre). Replanting costs per hectare are approximately $61.78 ($25.00 per acre) excluding technology fees which are approximately $88.92 per hectare ($35.00 per acre) (Bryant et al., 2003).
The soybean cyst nematode, Heterodera glycines Ichinohe, caused yield losses between 4.2 and 3.6 million metric tons in the U.S. between 1999 and 2002 (Wrather et al., 2003). Management strategies for H. glycines acknowledge the clear relationship of increasing H. glycines population densities with decreasing soybean yields (Niblack et al., 1992), and have the common goal of maintaining population densities of the nematode below economic damage threshold levels. These strategies include crop rotation, use of resistant cultivars and chemical control. Sources of host plant resistance against the nematode have been identified and incorporated into soybean germplasm and commercial soybean cultivars. Development of marker-assisted selection methods for H. glycines resistance (Vierling et al., 2000) enables more efficient introgression of soybean cyst nematode resistance genes into commercial cultivars. However, the diversity of soybean cyst nematode populations (Niblack et al., 2002) puts longevity and sustainability of using host plant resistance as the sole management strategy at risk because populations of H. glycines that are virulent on resistant soybean cultivars exist.
Vegetable transplant and bedding plant production are important components of U.S. agriculture in the southeastern states. Bedding plant production at $2.5 billion in 2004 accounted for over 50% of the entire value of floriculture crops in the U.S. (Jarardo, 2005). Vegetable (broccoli, cabbage, pepper, tomato, and others) and strawberry transplants were valued at $173 million in 2003 (USDA, NASS, 2004). Four southern states (FL, TX, GA, and NC) produced 16% of all these crops grown in the U.S. Fresh market tomatoes are an extremely important vegetable commodity. In several states (FL, GA, SC, TN and VA), they are the number one fresh market vegetable crop in terms of dollar value. Nine southern states account for 61% of the U.S. fresh market tomato crop (USDA, NASS, 2005). While of lower value, broccoli is often an important rotational crop for some growers. In Kentucky, for example, it is an important fall crop.
While bedding plants are transplanted into the landscape by people other than the grower, many vegetable producers grow their own transplants. For example, over 4,300 tomato transplants and over 11,000 broccoli transplants are needed per acre. In either situation, the soilborne pathogens that are problematic for cotton seedlingsR. solani and Pythium spp.are also problematic for bedding plants and vegetables in both the transplant and field production phases (Conover, 1949; Jones et al., 1991; Keinath and Farnham, 2001).
Production of many vegetable transplants and bedding plants take place in greenhouses where temperature and moisture conditions can be controlled. Although sanitation is practiced, many greenhouse facilities inadvertently provide conditions favorable for survival of damping-off pathogens such as Pythium spp. and R. solani. In the vegetable transplant and bedding plant commodities there is no tolerance for diseased plants. Thus, even one diseased plant in a flat of otherwise healthy transplants may result in the loss of the entire flat. In addition to damping-off disease, R. solani causes crown and root rot diseases on older transplants that magnifies the amount of loss to the producer due to the length of time plants were utilizing greenhouse resources prior to the loss. Although fungicides are effective in preventing Rhizoctonia crown and root rots, non-target problems can arise in their use since greenhouse operators capture and re-use irrigation water.
Bedding plants and vegetable transplants continue to be challenged by the same soilborne pathogens after planting into the landscape or field. Fungicide use post-plant can be effective but is limited in both situations. Once bedding plants are sold at retail and planted in the landscape around homes and businesses by the consumer, there are few control options other than general cultural practices like mulching and raised beds to limit root disease problems. With vegetables, concerns about worker exposure and fungicide resistance require that other methods of disease control be made available.
Biological controls could offer an alternative, if they were safe, effective and economical. Biological control using antagonistic microbes, alone or as supplements, to minimize the use of chemical pesticides has received increasing attention for cotton in recent years (Aqil and Batson, 1999; Hagedorn et al., 1993; Howell, 2002; Howell et al., 2000). Biological controls have been registered and used on cotton as an alternative and supplement to fungicides. Regional testing of seed treatment biological agents indicates that these agents do not perform as consistently as fungicides and suggest different strategies need to be examined. In-furrow treatments are commonly used on cotton, and planters are equipped for liquid or granular application in the planting furrow and may offer a method to increase the likelihood of establishing a biological agent and controlling seedling diseases.
The advantage of bedding plants and vegetable transplants treated with biocontrol agents during greenhouse production is that those plants may carry the biocontrol agent along on the root system when planted in the landscape or field. In the case of a biocontrol agent that is rhizosphere competent, this could extend the disease protection period through the life of the crop in the landscape or field. Isolates of binucleate Rhizoctonia sp. (BNR) have proven effective in preliminary experiments for control of both Pythium and Rhizoctonia damping-off in bedding plants (Burns and Benson, 2000; Honeycutt and Benson, 2001) and may also be effective in preliminary experiments for control of damping-off of vegetable transplants.
Plant growth-promoting rhizobacteria (PGPR) are naturally occurring root-colonizing bacteria which can induce increased plant growth (Cleyet-Marcel et al., 2001; Kloepper, 1994; Glick, 1995), often with concomitant reductions in plant diseases. The beneficial effects of PGPR for disease control have been reported for many crops and pathogens (Raupach et al., 1996; Raupach and Kloepper, 1998; Reddy et al., 1999; Reddy et al., 2000). The best strains and mixtures of PGPR activate the plants natural defense mechanisms in a phenomenon termed induced systemic resistance (ISR) (Raupach and Kloepper, 2000; Jetiyanon and Kloepper, 2002). Gustafson LLC commercialized a PGPR preparation under the name BioYieldä. The product is incorporated into the potting mix used to grow transplants and is a combination of two spore-forming PGPR Bacillus strains. Treated transplants show increased shoot and root growth leading to more rapid development than nontreated transplants. An ISR response is frequently observed.
Beauveria bassiana is a ubiquitous fungus with entomopathogenic properties and an extensive host range of pest insects. With few exceptions, research on B. bassiana as a control for plant pathogens has been limited to laboratory studies on growth and cell lysis. The potential of B. bassiana to control important seedling pathogens, such as R. solani and Pythium myriotylum has recently been demonstrated (Bishop, 1999; Ownley et al., 2000; Ownley et al., 2004; Seth et al., 2001; Seth, 2001; Marshall et al., unpublished data).
Introduction of commercial biological agents to field soils, whether for disease management or enhancing plant productivity, has not been consistently successful across all crops or even within a defined geographic region on a single crop. For example, our previous cooperative research suggested that biological-based seed treatments did not increase stand of snap bean in the southeastern U.S, although they were beneficial on other crops. A likely cause of the failures is our lack of understanding of the genetic diversity of soilborne pathogen populations and the indigenous soil microflora associated with plant roots. While state-to-state differences will exist, there are common underlying themes. Genera and species of deleterious and beneficial soil microflora often belong to the same culturable groups, even when isolated from different soil types, but genetic and physiological relationships apparently determine the specific role these organisms play in sustainable crop production. The same is also apparent for the organisms that cannot be cultured, but that now can be examined at the molecular level. Plant pathogens may appear morphologically similar, but they may be genetically different (or similar) within and among field locations.
Considerable diversity of R. solani exists in the southeastern U. S., with two of the three most recent anastomosis groups (AG-11 and AG-13) being identified from plants and soil in this region. The question is, Where do these fungi originate? Research has demonstrated that AG-3 associated with potatoes may have migrated in one direction (northeastern U.S. to N.C.) due to movement of seed potatoes (Ceresini et al., 2002a/b; Ceresini et al., 2003). How do other R. solani AG groups move? Do natural areas harbor R. solani and serve as new genetic material for this pathogen in field and greenhouse situations? While ornamental bedding plants, and to a lesser extent vegetable transplants, can be moved great distances within the region (and so move pathogens with them), direct seeded crop plants, such as cotton and soybean, do not move.
The soilborne pathogens Rhizoctonia and Pythium and the diseases they cause are common throughout the southeastern U.S. for virtually all plants, whether direct seeded into a field or initially grown in a greenhouse. Yet, we know little of their genetic background or origin. Because fungicides are not consistently efficacious, economical, ecologically desirable or commercially desirable to use (organic plant production), biological control and/or PGPR agents should be considered a component of an ecologically-based approach to manage these pathogens. Successful completion of the research in this proposed project will help to reduce our reliance on chemical pesticides and increase the sustainability of U.S. agriculture, while providing insight into the pathogen itself.
This group of scientists is not capable of conducting the proposed research project on an individual scientist basis. It requires a multi-state, team approach. Each scientist brings their own expertise and their own individual states perspective to the problems outlined. Within each state is a diversity of crop-growing regions that often bear no similarity to each other, but which are similar to a region in another state. We expand our knowledge of the problems by conducting similar experiments in diverse locations and then closely examining our results at our annual meetings in preparation for publication and outreach to the agricultural community.
Related, Current and Previous Work
The proposed project is a natural progression from the research that has been conducted over the last 35 years related to management of rhizosphere microorganisms, both pathogenic and beneficial. The first southern regional project on this topic was S-26 which initiated research on rhizosphere ecology. Research continued with S-90 (management of rhizosphere microorganisms), S-241 (management of rhizosphere dynamics to control soilborne pathogens), S-269 and S-302 (both on biological control of soilborne pathogens).
Regional evaluation of biological seed treatments was initiated with S-241, continued with S-269 and S-302, and will be continued with the new project. These studies have advanced our knowledge of their use in controlling seedling diseases. Annual results have been published in Biological and Cultural Tests for Control of Plant Diseases (see Batson et al., 2001a/b and 2003a/b/c for S-302 results). Summaries of a snap bean study and biocontrol agent survival on seed (snap bean and cotton) have been published (Keinath et al., 2000; Elliott et al., 2001), and a summary of a cotton study is forthcoming.
For S-302, biological seed treatments evaluated regionally included the bacterial genera Bacillus, Paenibacillus, and Stenotrophomonas, and the fungal genera Trichoderma and Beauveria. In addition to examining the effects of seed treatments on plant populations (and so disease control), seed populations of the biological control agents were assayed, both at treatment and at planting, to determine purity and viability. Seedling disease pathogens naturally present at test site locations included Rhizoctonia, Pythium and Fusarium. In previous projects, numerous biological seed treatments (both commercial and experimental) were evaluated. For S-302, the focus was placed on a limited number of seed treatments so cultivar and chemical seed treatment interactions with the biological seed treatments could be more closely evaluated.
The performance of these treatments varied across years and location. However, several treatments with Bacillus subtilis used alone were effective against seedling disease pathogens of cotton in at least one location in 3 of 4 years, resulting in significantly improved stand counts relative to the non-treated control. In the snap bean tests, significantly improved stand counts due to biological agents used alone (Bacillus subtilis and Trichoderma harzianum) were observed in only one year at one location for each agent. Except for one location in one year of the snap bean test, the addition of biological control agents to chemical seed treatment standards did not improve efficacy relative to chemical standards alone. Thus, while commercially available biological agents may not be useful for conventional agriculture as applied currently, they may be useful for organic agriculture and transitional agriculture. Significant interactions between cultivar and seed treatment were observed, although on a limited basis.
Other research conducted under S-302 further evaluated biological control agents included in the regional trials on crops other than cotton and snap bean. No additional benefit was observed when Bacillus spp. supplemented fungicide seed treatments of soybean. However, Bacillus and Paenibacillus spp. did increase yield for one tomato cultivar, even though the bacteria had no effect on disease incidence or severity. Germination and emergence of some ornamental bedding plants species (geranium, vinca and impatiens) were negatively impacted by Beauveria and Bacillus/Paenibacillus, either when used alone or together. The proposed project would expand beyond cotton and snap bean to include ornamental and other vegetable species in the regional biological control treatment trials.
Non-commercial biological control agents were also examined under S-302. Binucleate Rhizoctonia (BNR) incorporated into either soil or potting mix were efficacious in suppressing seedling diseases caused by R. solani on cotton and poinsettia. In both situations, the studies were in conjunction with the effect of cultural practices, such as cover crops or compost amendments, on BNR. These cultural practices do appear to have a positive effect on disease control, perhaps by providing a food base for the BNR. These results and earlier results (Cartwright and Benson, 1996; Cubeta and Echandi, 1991; Ross et al., 1998; Villajuan-Abgona et al., 1996) warrant further examination of BNR for biocontrol in the proposed project, and further examination of effect of cultural practices on soilborne pathogens and plant growth.
A new topic of study under S-302 was the genetic diversity of R. solani and the origin of that diversity (Ceresini et al., 2002a/b, 2003). The genetic diversity of 162 isolates of R. solani anastomosis group 3 (AG-3) was examined using somatic compatibility and DNA based (AFLP and PCR-RFLP) genetic markers. Results suggest that populations of R. solani AG-3 from soil in North Carolina and potato seed tubers from northern production areas in the U.S. (Maine and Wisconsin) and Canada are genetically diverse. The soil population of R. solani AG-3 from NC had a structure that included both clonality and recombination. Five of the most predominant clones found in North Carolina soil were also identified on potato tuber samples (seed population) originating from northern potato seed production areas. The relative contribution of one-way migration (unidirectional) of R. solani AG-3 on infested potato seed tubers originating from production areas in Canada, Maine and Wisconsin (source population) to the genetic diversity and structure of populations of R. solani AG-3 in North Carolina soil (recipient population) was identified as an important component for long distance dispersal of the pathogen. Unidirectional migration of R. solani AG-3 also provides an efficient mechanism to introduce new alleles and double stranded RNA (dsRNA) elements into recipient soil populations in North Carolina.
Considerable diversity of R. solani exists in the southeastern U. S., with two of the three most recent anastomosis groups (AG-11 and AG-13) being identified from plants and soil in this region (Carling et al., 1994; Carling et al., 2002; Tomaso-Peterson and Trevathan, 2004). The question is, Where do these isolates originate?. The importance of R. solani in this region also is indicated from the diversity of the crops in the region affected by this pathogen. Another reason for selecting R. solani is because a majority of the projected participants already are working on R. solani in NC, TN, SC, GA, FL, MS, and TX. Several cooperators are active participants in the International Rhizoctonia Working Group and have access to current techniques used for molecular genetic analysis and identification of anastomosis groups.
In the use of cultural practices, crop rotation has long been proposed as an effective strategy to manage H. glycines (Ross, 1962). In some cases, two to three years of non-host crops would be necessary to reduce population densities of H. glycines below threshold levels (Wrather et al., 1992). Such rotation intervals are difficult to achieve under current intensive production systems in the midwestern U.S., where rotating between corn and soybean is the norm. Recent shifts to reduced tillage practices may be beneficial in that population densities tend to be lower in no-till systems compared to conventional tillage systems (Workneh et al., 1999). In these studies, it was suggested that increased soil moisture under no-till facilitates and promotes the activity of nematode antagonists in soil. Although differences in soil chemical, physical and biological parameters among conventional and no-tillage systems have been documented (Aon et al., 2001), limited attention has been devoted to investigating disease suppressive soils in such systems. Most agricultural soils are expected to have some antagonistic potential towards plant-parasitic nematodes, and methods for detection of such soils have recently been summarized (Westphal, 2005). The underlying reason for reduced nematode numbers under reduced tillage systems has not been determined experimentally.
There is currently one active multistate project (W1147) that is similar to the proposed project in that its focus is on soilborne pathogens and biological controls of those pathogens. However, the diseases and crops of interest in W1147 are focused on root diseases of mature crops, including avocado, citrus, wheat and turfgrass, and not on seedling diseases of cotton, soybean, vegetables and bedding plants. Furthermore, the environmental conditions of the arid west are vastly different from the humid southeast. Thus, even research with the same organisms on the same crop would be complimentary and not duplicative research.
A developmental committee exists in the North Central region (NCDC204). Again, while their focus is also on biological control of soilborne pathogens, the diseases and crops of interest are different, and the goals are expanded to include foliar pathogens. This group has an additional objective to examine the molecular and cellular basis of traits affecting biological control agents, whereas we are interested in genetic traits of the pathogen.
Objectives
-
Examine commercial and non-commercial biocontrol agents for use as seed treatments, in-furrow treatments or as potting mix amendments.
-
Examine the effect of cultural practices on soilborne pathogens and plant growth.
-
Examine the genetic diversity of Rhizoctonia solani between natural ecosystems and agricultural ecosystems.
Methods
Objective 1: Examine commercial and non-commercial biocontrol agents for use as seed treatments, in-furrow treatments or potting mix amendments. While plant pathologists are permitted to ship biocontrol agents from state to state, we are not permitted to ship live pathogens without APHIS permits. Therefore, the biocontrol agents used by all participants in the experiments will be of the same formulation from one common source, with that source being dependent on the agent. However, inoculum of the target pathogen, Rhizoctonia solani, will be produced by each participant, if needed to induce disease. Data comparison will be made among participants within each cropping system and among cropping systems. Participants for each cropping system are listed in the "pathogen inoculum" subheading below. Biocontrol agents Binucleate Rhizoctonia (BNR) fungi from North Carolina and Arkansas as well as other potential locations will be formulated from a wheat bran biomass into a pesta formulation (Connick et al., 1991). The granular nature of the pesta formulation will allow for either surface application to growing flats, incorporation into potting mix, or in-furrow applications. Application rate is normally 0.5% on a volume to volume basis. A second formulation method for BNR will be made using infested millet seed. After a 2-week incubation period, colonized seed is air-dried and stored prior to use. Other candidate biocontrol agents (e.g., Beauveria bassiana, Trichoderma spp.) will be evaluated as they become available from industry, regional project cooperators, and other university and USDA scientists. These will be applied directly to seed or growing flats or incorporated into potting mix. Pathogen inoculum Bedding plants - AR, FL, KY, NC, SC Rhizoctonia solani will be grown for 10 to 14 days on sterile long grain rice. Generally, 18 ml deionized water is combined with 25 g rice grains in a 250-ml Erlenmeyer flask with aluminum foil cover. The rice medium is sterilized by autoclave for 40 min. The next day, a stiff bent wire is used to break apart the solid mass, and the rice is autoclaved again for 40 min. When cool, 3-5 agar disks of test fungus are added to the rice culture. Each day, the inoculated growth media will be agitated to keep the mycelium from binding rice grains together. Cotton - AR, LA, TN Rhizoctonia solani inoculum will be prepared by transferring agar plugs from PDA cultures into millet seed medium, 827 g millet seed plus 992 ml distilled water, in plastic containers. Millet seeds are autoclaved twice for 90 min, 24 h apart, before inoculation. Containers are incubated for two weeks at room temperature. After incubation, colonized grains are air dried and stored prior to use. Vegetable transplants - AR, KY, SC, TN Rhizoctonia solani will be grown in a corn meal-sand mix (3 g cornmeal + 100 g fine quartz sand + 7 ml deionized water). This mix is autoclaved for 30 min on 2 successive days prior to inoculating with the pathogen by transfer of agar plugs from PDA cultures. Greenhouse evaluation with bedding plants Impatiens plugs (Super Elfin White) will be purchased from a major plug supplier. Plugs will be transplanted to 6-cell packs with a peat moss based medium, such as Fafard no. 4P potting mix, and fertilized with 200 ppm-N (Peters liquid, 20-20-20) weekly. Impatiens in cell packs will be grown on a capillary mat pad with overhead watering until the canopies of individual plants begin to overlap with one another. The formulated biocontrol agent will be sprinkled over the surface of the cell pack and lightly watered to settle the formulation and remove any clinging to the plant foliage. The biocontrol agent formulation will be given 1 day to become established in the potting mix/plant rhizosphere. Inoculum of R. solani will consist of a single colonized rice grain that is placed on the potting mix surface about 1 cm from the stem of one central plant in the 6-cell pack. In untreated controls, the pathogen will grow from the rice grain to infect the nearby stem of the central plant causing a soil line lesion that eventually girdles the stem killing the seedling (crown rot). Subsequently, the infected seedling will serve as a food base for the pathogen to grow across the potting mix surface to infect other seedlings in the cell pack. Under favorable conditions, all unprotected plants can be killed within 2 weeks. The experiment will have four replications of each biocontrol agent treatment plus a standard fungicide drench such as thiophanate methyl or fludioxinil, the inoculated but untreated control and a non-inoculated control. Treatments will be arranged in a randomized complete block design on the greenhouse bench. Cell packs will be spaced far enough apart to avoid interplot interference. Cell packs will be observed weekly and the number of seedlings in each cell pack with crown rot noted. This will give an estimate of disease progress. Since plants with crown rot die, the percentage of the six plants that die in each cell pack after 4 weeks will be the basis for assessing disease severity. Appropriate ANOVA statistics with mean separations will be used to analyze the results. Landscape evaluation with bedding plants Impatiens plugs will be transplanted to cell packs, and then grown on a capillary mat pad with overhead watering as above. The formulated biocontrol agent will be sprinkled over the surface of the cell pack immediately following transplanting and lightly watered to settle the formulation and remove any clinging to the plant foliage. Plants will be grown for an additional six weeks to simulate commercial production in the greenhouse. The effects of treatments on seedling survival, leaf size and plant height will be recorded prior to transplanting in the landscape. One week prior to transplanting to landscape beds, one half of the treated plants will be given a second surface application of biocontrol agent to compare the ability of the putative biocontrol agents to establish long term in the root zone. The market size impatiens would then be planted in a landscape bed (field) where R. solani was known to exist and observed over the course of the growing season for development of Rhizoctonia crown and root rot. Plants would be rated for disease as: 1=healthy, 2=infected with crown rot lesions, or 3=plant dead, due to crown and root rot. Untreated control plants planted in a randomized complete block design with the biocontrol agent treated plants would serve as a measure for comparing disease control. Plant growth [(height + width)/2] would be measured at monthly intervals during the growing season so beneficial or detrimental effects of biocontrol agents on growth could be measured. Greenhouse and field evaluation of vegetable transplants The efficacy of a Tennessee isolate of B. bassiana (11-98), commercial B. bassiana, and other biological control agents will be evaluated on tomato transplants for control of pre- and post-emergence damping-off caused by R. solani. Untreated controls and fungicide standards will be included in tests. The potting mix will be inoculated with the pathogen in the cornmeal-sand mixture. Control agents will be mixed with the potting mix or applied as seed treatments. The effects of treatments on disease incidence and transplant growth will be recorded. Broccoli will be used as a test crop for field evaluation of the efficacy of biological controls and also to determine the treatment effects on plant growth and yield. Depending on the nature of the biological agent, treatments will either be applied to seed or mixed with the peat-based potting mixes used to grow the broccoli transplants. The effects of treatments on seedling survival, and leaf size and/or stem diameter of representative plants will be recorded prior to transplanting. Materials to be evaluated will be replicated a minimum of four times in standardized field tests. Each replicate will contain a minimum of 20 plants. The effects of treatments on seedling disease incidence, plant height, head diameter, and yield will be recorded at regular intervals. In addition to the biological agents, each test will include an untreated control and one or more chemical standards. Evaluations will be conducted in both greenhouses and in fields naturally infected with R. solani and other soilborne pathogens and will be conducted during periods when soil temperatures favor pathogen activity. Irrigation will be used as needed to create disease-conducive conditions. Soil temperatures will be monitored, rainfall and irrigation events will be recorded, and the fertility and soil texture of test sites analyzed. As appropriate, interaction between biocontrol agents and cultural practices (e.g., herbicide use, fertilizers, planting date and cultivar) on the targeted soilborne diseases will be evaluated. Data collected in both the transplant production phase and the field evaluation phase will be analyzed to determine significant treatment effects on disease control efficacy, plant growth, and yield. The correlation of treatment efficacy with other test parameters (e.g., soil temperature, rainfall events, soil texture, etc.) will be determined to identify the conditions needed for optimal efficacy of biological control agents. Field evaluation with cotton seed Seed will be commercially treated with fungicides. The formulated biocontrol agents will be applied in the furrow as granular or liquid applications depending on the product, with no biological seed treatment and a fungicide in-furrow treatment as controls. Plot size will be two or four rows by a minimum length of 13 m. The design will be a randomized complete block with a minimum of four replications. The tests will rely on natural inoculum. A separate test or an additional treatment of inoculum of R. solani as millet seed in the planting furrow may be used at some sites. Stand establishment will be assessed as stand counts at 3 and 6 weeks and stand uniformity (Skip index) at 6 weeks. Plots will be harvested for seed cotton yield. Disease will be assessed by rating seedlings arbitrarily dug from plots 3 weeks after planting. Biocontrol agent and pathogen assays - all participating locations Persistence of the introduced biocontrol agents will be monitored as follows. For BNR biocontrol fungi either alkaline water agar with antibiotic or a Ko and Hora medium (Ko and Hora, 1971) will be used. For other biocontrol agents, appropriate media would be used. Root segments will be assayed, and the number of colonies growing out of root segments determined after 24 and 48 hours. Timing of assay is dependent on cropping system - e.g., 3 weeks for cotton seedlings; up to 42 days for bedding plants. Samples from untreated plants would serve as a control. Persistence of the introduced biocontrol agents, specifically the BNR fungi, in the potting mix used for bedding plant production will be monitored. Samples of potting mix and roots would be collected at 1, 7, 14, 21, and 42 days after application of biocontrol formulation. Potting mix samples could be assayed for BNR fungi with the multipelleter sampler (Henis et al., 1978) on Ko and Hora medium. The presence and identity of plant pathogens affecting bedding plants, vegetable transplants and cotton seedlings will be verified by plating represntative diseased plants on selective media. Objective 2: Examine the effect of cultural practices on soilborne pathogens and plant growth. Crop rotation is a common cultural method of managing soilborne pathogens, and is based on the knowledge that the population of many plant pathogens is reduced when the pathogen's host is not present. Recently, other theories of exploiting plants to manage soilborne pathogens have begun to emerge. This includes the growing of specific plant species (and often specific cultivars) in a field setting, often followed by the incorporation of this plant material into the soil prior to planting with the desired crop species. Because this is an emerging technology, the proposed experiments for this objective are exploratory, with four participating states (AR, IN, SC, TN) examining different uses of this technology technology that may then be exploited by all of the participating states during the progression of this particular project. Thus, while the research under this objective is initially perhaps not as coordinated as that described under Objectives 1 and 3, this objective is serving the group's overall goal of examining influences on the ecological diversity of soilborne pathogens and indigenous microflora. Commonality of experiments will provide the group with data to develop a more coordinated set of experiments. Two plant families are being examined for their beneficial effects (Brassicaceae and Fabaceae), both of which produce gases that suppress plant pathogens when the plants are degraded after incorporation into the soil. Brassicaceae species either have resistant traits against plant-parasitic nematodes or have high biofumigation potential (Kirkegaard and Sarwar, 1998). The latter is a process that occurs when glucosinolate rich plant material is incorporated into soil and releases isothiocyanates during natural decomposition. These chemicals reduce populations of soilborne plant pathogens (Sarwar et al., 1998). This project will primarily focus on the plant species Brassica juncea (Indian mustard). Such brassicas will be evaluated for crop production potential in Indiana, South Carolina and Tennessee. The second plant family is Fabaceae, with Vicia the genus of interest. Hairy vetch (Vicia villosa) was previously examined in AR during S-269 for control of cotton seedling diseases. Candole and Rothrock (1997a) determined that ammonia was produced when hairy vetch was incorporated into the soil, which accounted for the disease suppression effect observed (Candole and Rothrock, 1997b; Rothrock et al., 1995). The hairy vetch was grown as a winter cover crop prior to incorporation into the soil. Recently, Zhou and Everts (2004) have used this technology to suppress Fusarium wilt of watermelon in Maryland. Suppression towards pathogens important in Indiana, namely root knot nematodes (Meloidogyne spp.) in watermelon, will be evaluated using Brassica juncea, Raphanus sativus or related Brassicaceae as the suppressive plant species. This technology aims to take advantage of the combined action of host plant resistance of the cover crops towards root knot nematodes and biofumigation effects of Brassica tissue. These tests will be conducted as field studies for the plant species that have shown some beneficial potential for pest suppression, and in greenhouse studies for those that need to be characterized for host status and suppression of plant-parasitic nematodes and fungal diseases. In field trials, it will be determined how to implement this technology in existing production systems. Indiana growing conditions allow for short growing periods for cover crops. In the fall, fields are occupied until shortly before cessation of growth and the precrop period before the spring planting of watermelon in May is limited. Planting date strategies and choice of possible overwitnering Brasscaceae will be investigated. AR will evaluate the suppressive effect of B. juncea (specifically, cv. Fumus) on vegetables and cotton. Two cotton fields will be used. Field one has a severe reniform nematode infestation. Field 2 has a severe root-knot nematode problem and Fusarium wilt. Both fields have the seedling disease pathogens; R. solani, Pythium spp., and Thielaviopsis basicola. Large strips (6 or 8 rows wide) will be overseeded across the field with the cover crop prior to cotton defoliation. The treatments in the fields will be no treatment, B. juncea, and Telone. Pathogen populatios will be monitored. Disease and cotton development will be measured throughout the season. Yields will be taken for cover crop biomass and cotton lint. Small plot tests will be initiated on vegetables using B. juncea Fumus. Similar data will be collected in these tests. In TN, Brassica juncea 'Florida Broadleaf' mustard and other brassicas crops will be grown, mulched into soil, and evaluated in field trials for suppression of naturally-occurring fungal diseases of wheat; and for control of take-all using furrow incorporated Gaeumannomyces inoculum produced on oat grains. In laboratory assays, 'Florida Broadleaf' mustard mulch suppressed growth of Gaeumannomyces and Fusarium isolates from diseased wheat roots. In growth chamber studies, mustard mulch incorporated into soil with inoculum of Gaeumannomyces graminis var. tritici from infected wheat roots, reduced severity of take-all in a subsequent wheat planting (Breeden, 2005; Breeden et al., unpublished). Field experiments will be replicated and treatment plots will be 5 rows x 50 m length. Treatment effects will be assessed by measurements of disease incidence and severity, and plant growth (height and number of heads) of selected portions of each treatment plot. Yield of each plot will also be determined. SC will expand upon the research of AR (with cotton) and the more recent MD research with watermelon In South Carolina, hairy vetch (Vicia villosa), previously used in Maryland to suppress Fusarium wilt of watermelon (Zhou and Everts, 2004), will be to compared V. villosa (hairy vetch) with 'Cahaba White', a root-knot-resistant hybrid common vetch cultivar (V. sativa x V. cordata), and rye as the negative control for suppression of Fusarium wilt of watermelon. The experiment will be conducted as a 3 x 2 factorial design with five replicates. The main plot treatments will be cover crops, and subplot treatments will be seedless watermelon cultivars 'Sugar Heart' (susceptible to race 1 and 2 of Fusarium oxysporum f. sp. niveum [FON]) and 'Seedless Sangria' (moderately resistant to race 1 FON). Hairy vetch and rye will be seeded November 1 at 45 and 30 lb/A, respectively. In March, cover crops will be flattened, sprayed with herbicide, and disked to incorporate to a depth of 6 inches before raised beds covered with black polyethylene mulch are formed. Three-week-old watermelon seedlings will be transplanted into the plots. The diploid cultivar 'Royal Majesty', highly resistant to race 1 of FON, will be planted to serve as a pollenizer. Incidence of Fusarium wilt will be monitored biweekly throughout the growing season. Total and marketable yields, expressed as weight and number of fruit, will be measured from three harvests. The pathogen will be isolated from infected vines and the race present will be identified in greenhouse tests using differential cultivars (Egel et al., 2005). Objective 3: Examine the genetic diversity of Rhizoctonia solani between natural ecosystems and agricultural ecosystems.Rhizoctonia species will be obtained from different agricultural cropping systems (rice, cotton, soybean and vegetables). At least two sites (states) will be used for each cropping system. A closely situated natural ecosystem (i.e., an ecosystem that represents what was present prior to agriculture, is being reverted back to a natural ecosystem, or is situated close to agriculture) will be sampled for each agricultural site. Cropping system--------Native site----------Participating State
Rice--------------------Everglades-------------FL
Rice/soybean------------Prairie----------------AR
Cotton------------------White River or Delta---AR
Cotton------------------Oak-pine forest--------NC
Corn/soybean------------Forested wetland-------NC
Corn/soybean------------Restored strip mine----IN
Vegetable (legume)------Forested wetland-------SC
Vegetable (solanaceous--Oak-hickory forest-----TN
Participants in each state listed above will obtain isolates of Rhizoctonia from agricultural crop or native species of plants and soil, using the similar isolation and storage techniques described in Objective 1. The sites will be selected based on their history of cultivation (or lack of cultivation), their proximity to agricultural production areas, and whether field sites have or are transitioning into or out of agricultural production. Physical and chemical properties of the soils will be determined by a single analytical laboratory. Isolates will be obtained from plants by washing 2-cm long pieces of tissue in running tap water for 30 minutes, followed by blotting on a sterile paper towel and plating on water agar amended with 50 µg/ml streptomycin sulfate and tetracycline. Isolates will be obtained from soil by baiting (beet seed and sterile toothpicks) and soil pelleting techniques. The baits will be washed in running tap water and will be placed directly on two different semi-selective media (Flowers, 1976; Ko and Hora, 1971). A soil pelleting apparatus (Henis et al., 1978) will be used to place soil directly on these two semi-selective media. All plates will be incubated at two different temperatures (15C and 25C). Hypha of each isolate will be stained with 0.1% phenosafranin and in 3% KOH to determine their nuclear condition and will be stored in 50% glycerol/potato dextrose broth at -80 C for future determination of anastomosis group (AG). Isolates also will be stored on Whatman FTA cards and as boiled hyphae for saving DNA for future molecular analysis (Gitaitis et al., 2005). At least 60 isolates will be stored from plants and from soil for each site. At least 20 isolates of the AG causing disease on that crop will be stored per site. Anastomosis group (AG) affinity will be determined by sequence analysis of ribosomal RNA and beta-tubulin genes (Gonzalex et al., 2001, 2006), and will be confirmed with specific AG tester isolates using the technique of Kronland and Stanghellini (1988) in accordance with the reaction types described by Carling (MacNish et al., 1993; Carling, 1996). Since the actual molecular analysis does not require living cultures, the FTA cards and boiled hyphae can be shipped to other labs for analysis (NC and FL and perhaps others), but the same protocol will be used at all labs. Morphological confirmation of the molecular identification does require the use of living cultures (i.e., AG tester strains). These studies will therefore be carried out in participating institutions that are able to acquire the appropriate permits, but the protocol remains the same for all labs. All data will be collected in a central location, most likely by developing a password protected web site at one of the participating institutions.
Measurement of Progress and Results
Outputs
- Data obtained from the biocontrol evaluation experiments will be analyzed and published in Biological and Cultural Tests for Control of Plant Diseases. Collected Rhizoctonia isolates will be stored and characterized morphologically and molecularly, with data published in a refereed journal. Data obtained from the experiments evaluation cultural practices on soilborne pathogens and plant growth will be summarized and published in a refereed journal.
Outcomes or Projected Impacts
- Reduction in fungicide use will likely be achieved by use of biological control agents and cultural practices to control soilborne plant pathogens. Increased plant growth, yield or plant longevity may be achieved by the introduction of plant-growth promoting rhizosphere microorganisms (seed or soil treatments) or incorporation of brassica species into the soil. Population diversity of Rhizoctonia (species and AG) in native versus agricultural ecosystems and plants versus soil in an individual ecosystem will be documented, as will the influence of baiting or soil plating and media and temperature on recovery of Rhizoctonia species.
Milestones
(0):le results from each year's evaluation of biological control agents will be used to determine design of the following year's greenhouse and field experiments, each biocontrol agent will be evaluated on the same crop for at least two years. The Rhizoctonia isolates will be collected, stored and identified morphologically within the first three years in order to proceed with molecular characterization of selected isolates. The biofumigation experiments will proceed for a minimum of two years before determining whether to proceed with the same brassica species for future experiments.Projected Participation
View Appendix E: ParticipationOutreach Plan
Results of the project will be made available to the scientific community, Extension Specialists and County Agents via refereed publications, technical reports (e.g., Biological and Cultural Control Tests), internal university reports, and research and extension publications. A webpage will be maintained at North Carolina State University. Graduate, undergraduate and high school students would be involved at all stages of the project.
Organization/Governance
The executive committee is composed of a Chair and Secretary, with a new Secretary elected each year at the annual meeting. The Secretary automatically rotates into the Chair position the following year. The Secretary is responsible for writing and submitting minutes of the annual meeting to the Chair. The Chair is responsible for writing and submitting the annual report to the appropriate group. Subcommittees will be formed specifically for the writing of grants to support the project and to write journal articles for publication.
Literature Cited
Aqil, T., and Batson, W. E. 1999. Evaluation of a radical assay for determining the biological activity of rhizobacteria to selected pathogens of the cotton seedling disease complex. Pakistan Journal of Phytopathology 11:30-40.
Altman, J. and Campbell, C.L. 1977. Effect of herbicides on plant diseases. Annual Review of Phytopatholgy 15:361-385.
Aon, M.A., Sarena, D.E., Burgos, J.L., and S. Cortassa 2001. (Micro)biological, chemical and physical properties of soils subjected to conventional or no-tillage management: an assessment of their quality status. Soil and Tillage Research 60:173-186.
Batson, W. E. Jr., Caceres, J., Benson, M., Brannen, P. M., Cubeta, M. A., Conway, K., Elliott, M. L., Fajardo, J., Huber, D. M., Keinath, A. P., Newman, M., Ownley, B., Rothrock, C. S., Schneider, R. W., Molsenbocker, C. E., Sumner, D. R., and Thaxton, P. 1999. Efficacy of biological agents as seed treatments for cotton stand establishment. Pages 103-104 in: Proc. Beltwide Cotton Conf. Vol. 1. Jan 3-7 1999, Orlando, Florida, National Cotton Council of America, Memphis, TN.
Batson, Jr., W.E., Caceres, J., Elliott, M.L., Huber, D.M., Hickman, M.V., McLean, K.S., Ownley, B., Newman, M., Padgett, G. Boyd, Rushing, K.W., Kenny, D.S., Rothrock, C.S., Seebold, K., and Thaxton, P. 2003a. Biological seed treatment evaluations for control of the cotton seedling disease complex, 2001. Biological and Cultural Tests for Control of Plant Diseases. Report 18:F002.
Batson, Jr., W.E., Caceres, J., Benson, M., Cubeta, M.A., Elliott, M.L., Isakeit, T., McLean, K.S., Padgett, G.B., Rothrock, C.S., and Seebold, K. 2003b. Evaluation of selected biological seed treatments for cotton seedling disease control, 2002. Biological and Cultural Tests for Control of Plant Disease. Report 18:F003.
Batson, Jr., W.E., Caceres, J., Benson, M., Cubeta, M.A., Elliott, M.L., Huber, D.M., Hickman, V., Keinath, A.P., Dubose, V., McLean, K.S., Ownley, B., Canaday, C., Rothrock, C.S., and Seebold, K. 2003c. Evaluation of biological seed treatments for control of the seedling disease of snap bean, 2001. Biological and Cultural Tests for Control of Plant Disease. Report 18:V029.
Batson, Jr., W.E., Caceres, J., Benson, M., Cubeta, M.A., Elliott, M.L., Huber, D.M., Hickman, M.V., McLean, K.S., Ownley, B., Newman, M., Rothrock, C.S., Rushing, K.W., Kenny, D.S., and Thaxton. P. 2001a. Biological seed treatment evaluations for control of the seedling disease complex of cotton, 2000. Biological and Cultural Tests for Control of Plant Diseases. Report 16:F12.
Batson, Jr., W.E., Caceres, J., Benson, M., Cubeta, M.A., Elliott, M.L., Huber, D.M., Hickman, M.V., Keinath, A.P., Dubose, V., McLean, K.S., Ownley, B., Canaday, C., Rushing, K.W., and Kenny, D.S. 2001b. Evaluation of biological seed treatments for control of the seedling disease complex of snap bean, 2000. Biological and Cultural Tests for Control of Plant Diseases. Report 16:V81.
Bradley, C.A., Hartman, G.L., Wax, L.M., Pedersen,W.L. 2002. Influence of herbicides on Rhizoctonia root and hypocotyl rot of soybean. Crop Protection 21(8):679-687.
Breeden, T. S. 2005. Brassica mulches and meal control fungal wheat pathogens in vitro and take-all disease in soil. M. S. Thesis, The University of Tennessee, Knoxville.
Bryant, Kelly J., Stiles, H.S., Hogan Jr., R., and Windham, T.E.. 2003. Estimating 2004 Costs of Production: Cotton. Univ. of Arkansas, Division of Agriculture, Coop. Ext. Serv., Little Rock, AR. AG-768-12-03.
Burns, J. R., and Benson, D. M. 2000. Biocontrol of damping-off of Catharanthus roseus caused by Pythium ultimum with Trichoderma virens and binucleate Rhizoctonia fungi. Plant Disease 84: 644-648.
Canaday, C. H. 2006a. Effects of cultivar, a SAR inducer, and PGPR on foliar and fruit diseases and yields of staked-tomato, 2005. Biological and Cultural Tests for Control of Plant Diseases. 21:V010
Canaday, C. H. 2006b. Evaluation of seed treatments and an in-furrow spray for control of snap bean seedling diseases, 2005. Fungicide and Nematicide Tests 61:ST001
Canaday, C. H., Helsel, D. G., and Wyllie, T. D. 1986. Effects of herbicide-induced stress on root colonization of soybeans by Macrophomina phaseolina. Plant Disease 70:863-866.
Canaday, C. H., Sams, C. E., Wyatt, J. E., and Schmitthenner, A.F. 1999. Increases in the incidence of seedling diseases associated with chloride salts. Phytopathology 89:S93.
Candole, B.L., and Rothrock, C.S. 1997a. Characterization of the suppressiveness of hairy veth-amended soils to Thielaviopsis bassicola. Phytopathology 87:197-202.
Candole, B.L., and Rothrock, C.S. 1997b. Using marked strains to assess the effect of hairy vetch amendment on the inoculum densities of Thielaviopsis basicola, Pythium ultimum and Rhizoctonia solani. Soil Biology and Biochemistry 30:443-448.
Cardoso, J.E., and Echandi, E. (1987b) Biological control of Rhizoctonia root rot of snap bean with binucleate Rhizoctonia-like fungi. Plant Disease 71:67-170.
Carling, D.E. 1996. Genetics of Rhizoctonia species. Pages 37-47 in: Rhizoctonia Species Taxonomy, Molecular Biology, Ecology, Pathology and Disease Control. Sneh, B., Jabaji-Hare, S., Neate, S., and Dijst, G., eds. Kluwer Academic, Dordrecht.
Carling, D.E., Rothrock, C.S., MacNish, G. C., Sweetingham, M.W., Brainard, K.A., and Winters, S. W. 1994. Characterization of anastomosis group 11 (AG-11) of Rhizoctonia solani. Phytopathology 84:1387-1393.
Carling, D.E., Baird, R.E., Gitaitis, R.D., Brainard, K.A., and Kuninaga, S. 2002. Characterization of AG-13, a newly reported anastomosis group of Rhizoctonia solani. Phytopathology 92:893-899.
Cartwright, D. K., and Benson, D. M. 1996. Efficacy of binucleate Rhizoctonia fungi to control Rhizoctonia damping-off of impatiens. Phytopathology 86:S104-105 (Suppl.)
Ceresini, P.C., Shew, H.D., Vigalys, R., Rosewich-Gale, U.L., and Cubeta, M.A. 2003. Detecting migration in populations of Rhizoctonia solani AG-3 from potato in North Carolina using multilocus genotype probabilities. Phytopathology 93:610-615.
Ceresini, P.C., Shew, H.D. Vilgalys, R., and Cubeta, M.A. 2002a. Genetic diversity of Rhizoctonia solani AG-3 from potato and tobacco in North Carolina. Mycologia 94:437-449.
Ceresini, P.C., Shew, H.D. Vilgalys, R., Rosewich, U.L., and Cubeta, M.A. 2002b. Genetic structure of populations of Rhizoctonia solani AG-3 on potato in eastern North Carolina Mycologia 94:450-460.
Chambers, A. Y. 1995. Comparative effects of no-till and conventional tillage on severity of three major cotton diseases in Tennessee. In: M. R. McCelland et al. (ed.) Conservation-tillage systems for cotton: A review of research and demonstrations results from across the Cotton Belt. Arkansas Agric. Exp. Stn. Bull.
Cleyet-Marcel, J.C., M. Larcher, H. Bertrand, S. Rapior, and X. Pinochet. 2001. Plant growth enhancement by rhizobacteria. Pages 185-197 in: Nitrogen Assimilation by Plants: Physiological, Biochemical, and Molecular Aspects. J.F. Morot-Gaudry, ed. Science Publishers, Inc., Plymouth, U.K.
Colyer, P. D., Micinski, S., and Nguyen, K. T. 1991. Effects of planting date on the efficiency of an in-furrow pesticide and the development of cotton seedling disease. Plant Disease 75:739-742.
Colyer, P. D. and Vernon, P. R. 2005. Impact of stale seedbed production on seedling diseases in cotton. Plant Disease 89:744-748.
Connick, W. J., Boyette, C.D., and McAlpine, J. R. 1991. Formulation of mycoherbicides using a pasta-like process. Biological Control 1:281-287.
Conover, R.A. 1949. Rhizoctonia canker of tomato. Phytopathology 39:950-951.
Cubeta, M.A., and Echandi, E. 1991 Biological control of Rhizoctonia and Pythium damping off of cucumber: An integrated approach. Biological Control 1:227-236.
DeVay, J. E. 2001. Seedling Diseases. Page 13-14 in: Compendium of Cotton Diseases, 2nd ed. T. L. Kirkpatrick, and C. S. Rothrock, eds. APS Press, St. Paul, MN.
Egel, D. S., Harikrishnan, R., and Martyn, R. 2005. First report of Fusarium oxysporum f. sp. niveum race 2 as causal agent of Fusarium wilt of watermelon in Indiana. Plant Disease 89:108.
Elliott, M.L., Des Jardin, E.A., Batson Jr., W.E., Caceres, J., Brannen, P.M., Howell, C.R., Benson, D.M., Conway, K.E., Rothrock, C.S., Schneider, R.W., Ownley, B.H., Canaday, C.H., Keinath, A.P., Huber, D.M., Sumner, D.R., Motsenbocker, C.E., Thaxton, P.M., Cubeta, M.A., Adams, P.D., Backman, P.A., Fajardo, J., Newman, M.A., and Pereira, R.M. 2001. Viability and stability of biological control agents on cotton and snap bean seeds. Pest Management Science 57:695-706.
Flowers, R.A. 1976. A selective medium for isolation of Rhizoctonia solani from soil and plant tissue. (Abstr.) Proceeding of the American Phytopathological Society 3:219.
Gitaitis, R., Martinex, N., Seebold, K., Stevenson, K., Sanders, H., and Mullis, S. 2005. PCR amplification of nucleic acids of bacteria, fungi and viruses recovered and stored on FTA cards. Phytopathology 95:S35.
Glick, B.R. 1995. The enhancement of plant growth by free-living bacteria. Canadian Journal of Microbiology 41:109-117.
Gonzalez, D., Vilgalys, R., and Cubeta, M.A. 2006. Phylogenetic utility of indels within sequences of ribosomal and beta-tubulin genes in fungi belonging to the Rhizoctonia species complex. Mol. Phylogen. Evol. 40:459-470.
Gonzalez, D, Carling, D., Kuninaga, S., Vilgalys, R., and Cubeta, M.A. 2001. Ribosomal DNA systematics of Ceratobasidium and Thanatephorus with Rhizoctonia anamorphs Mycologia 93:1138-1150.
Hagedorn, C., Gould, W. D., and Bardinelli, T. R. 1993. Field evaluations of bacterial inoculants to control seedling disease on cotton. Plant Disease 77:278-282.
Harikrishnan, R., and Yang, X.B. 2002. Effects of herbicides on root rot and damping-off caused by Rhizoctonia solani in glyphosate-tolerant soybean. Plant Disease 86:1369-1373.
Henis, Y. Ghafar, A., Baker, R., and Gillespie, S. L. 1978. A new pellet soil sampler and its use for the study of population dynamics of Rhizoctonia solani in soil. Phytopathology 68:371-376.
Hillocks, R. J. 1992. Cotton Diseases. CAB International, Oxon.
Honeycutt, E. W., and. Benson, D. M. 2001. Formulation of binucleate Rhizoctonia spp. and biocontrol of Rhizoctonia solani on impatiens. Plant Disease 85:1241-1248.
Howell, C. R. 1982. Effect of Gliocladium virens on Pythium ultimum, Rhizoctonia solani, and damping-off of cotton seedlings. Phytopathology 72:496-498.
Howell, C. R. 1991. Biological control of Pythium damping-off of cotton with seed-coating preparations of Gliocladium virens. Phytopathology 81:738-741.
Howell, C. R. 2001. Diseases caused by Pythium species. Pages 14-15 in: Compendium of Cotton Diseases, 2nd ed. T.L. Kirkpatrick, and C.S. Rothrock, eds. APS Press, St. Paul, MN.
Howell, C. R. 2002. Cotton seedling preemergence damping-off incited by Rhizopus oryzae and Pythium spp. and its biological control with Trichoderma spp. Phytopathology 92:177-180.
Howell, C. R., and Stipanovic, R. D. 1995. Mechanisms in the biocontrol of Rhizoctonia solani-induced cotton seedling disease by Gliocladium virens: Antibiosis. Phytopathology 85:469-472.
Howell, C. R., Hanson, L. E., Stipanovic, R. D., and Puckhaber, L, S. 2000. Induction of terpenoid synthesis in cotton roots and control of Rhizoctonia solani by seed treatment with Trichoderma virens. Phytopathology 90:248-252.
Jarardo, A. 2005. Floriculture and nursery crops situation and outlook yearbook. USDA, ERS FLO-2005 (http://www.ers.usda.gov/publications/flo/Jun05/FLO2005.pdf).
Jetiyanon, K., and J.W. Kloepper. 2002. Mixtures of plant growth-promoting rhizobacteria for induction of systemic resistance against multiple plant diseases. Biological Control 24:285-292.
Jones, J.B., Jones, J.P., Stall, R.E., and Zitter, T.A. eds. 1991. Compendium of Tomato Diseases. APS Press, St. Paul, MN.
Katan, J,. and Eshel, Y. 1973. Interactions between herbicides and plant pathogens. Residue Review 45:145-177.
Keinath, A.P., and Farnham, M.W. 2001. Effect of wirestem severity on survival and head production of transplanted broccoli and cabbage. Plant Disease 85:639-643.
Keinath, A.P., Batson Jr., W.E., Caceres, J., Elliott, M.L., Sumner, D.R., Brannen, P.M., Rothrock, C.S., Huber, D.M., Benson, D.M., Conway, K.E., Schneider, R.N., Motsenbocker, C.E., Cubeta, M.A., Ownley, B.H., Canaday, C.H., Adams, P.D., Backman, P.A., and Fajardo, J. 2000. Evaluation of biological and chemical seed treatments to improve stand of snap bean across the southern United States. Crop Protection 19:501-509.
Kirkegaard, J.A., and Sarwar, M. 1998. Biofumigation potential of brassicas: I. Variation in glucosinolate profiles of diverse field-grown brassicas. Plant and Soil 201:7189.
Kloepper, J. W. 1994. Plant Growth-Promoting Rhizobacteria. Pages 137-166 in: Azospirillum/Plant Associations. Y. Okon, eds. CRC, Boca Raton, FL.
Ko, W. and Hora, F. K. 1971. A selective medium for the quantitative determination of Rhizoctonia solani in soil. Phytopathology 61:707-710.
Kronland, W. C., and Stanghellini, M. E. 1988. Clean slide technique for the observation of anastomosis and nuclear condition of Rhizoctonia solani. Phytopathology 78:820-822.
MacNish, G. C., Carling, D. E., and Brainard, K. A. 1993. Characterization of Rhizoctonia solani AG-8 from bare patches by pectic isozyme (zymogram) and anastomosis techniques. Phytopathology 83:922-927.
Melero-Vara, J. M., and Jimenaz-Diaz, R. M. 1990. Etiology, incidence, and distribution of cotton seedling damping off in southern Spain. Plant Disease 74:597-600.
Minton, E. B., and Garber, R. H. 1983. Controlling the seedling disease complex of cotton. Plant Disease 67:115-118.
Minton, E. B., Papavizas G. C., and Lewis, J. A. 1982. Effect of fungicide seed treatments and seed quality on seedling disease and yield of cotton. Plant Disease 66:832-835.
Niblack, T.L., Baker, N.K., and Norton, D.C. 1992. Soybean yield losses due to Heterodera glycines in Iowa. Plant Disease 76:943-948.
Niblack T.L., Arelli, P.R., Noel, G.R., Opperman, C.H. Ore, J.H., Schmitt D.P., Shannon, J.G., and Tylka, G.L. 2002. A revised classification scheme for genetically diverse populations of Heterodera glycines. Journal of Nematology. 34(4):279-288.
Ownley, B.H., Bishop, D.G., and Pereira, R.M. 2000. Biocontrol of Rhizoctonia damping-off of tomato with Beauveria bassiana. Phytopathology 90:S58.
Ownley, B. H., Pereira, R. M., Klingeman, W. E., Quigley, N. B., and Leckie, B.M. 2004. Beauveria bassiana, a dual purpose biocontrol organism, with activity against insect pests and plant pathogens. Emerging Concepts in Plant Health Management, R. T. Lartey and A. J. Caesar, eds., Research Signpost, Kerula, India.
Raupach, G.S., and Kloepper, J.W.. 2000. Biocontrol of cucumber diseases in the field by plant growth-promoting rhizobacteria with and without methyl bromide fumigation. Plant Disease 84:1073-1075.
Raupach, G.S., and Kloepper, J.W.. 1998. Mixtures of plant growth-promoting rhizobacteria enhance biological control of multiple cucumber pathogens. Phytopathology 88:1158-1164.
Raupach, G.S., Liu, L., Murphy, J.F., Tuzun, S, and Kloepper, J.W. 1996. Induced systemic resistance in cucumber and tomato against cucumber mosaic virus using plant growth-promoting rhizobacteria (PGPR). Plant Disease 80:891-894.
Reddy, M.S., Rodriguez-Kabana, R., Kenney, D.S., Ryu, C.M., Zhang, S., Yan, Z., Martinez-Ochoa, N., and Kloepper, J.W. 1999. Growth promotion and induced systemic resistance mediated by a biological preparation. Phytopathology 89: S39.
Reddy, M.S., Ryu, C.M., Zhang, S., Yan, Z., Kenney, D.S., Rodriguez-Kabana, R., and Kloepper, J.W. 2000. Approaches for enhancing PGPR-mediated ISR on various vegetable transplant plugs. Auburn University Website, Available at: http://www.ag.auburn.edu/argentina/pdfmanuscripts/reddy.pdf
Rodriguez-Kabana, R. and Curl, E.A. 1980. Nontarget effects of pesticides on soilborne pathogens and disease. Annual Review of Phytopathology 18:311-332.
Rodriguez-Kabana, R., Curl, E.A., and Funderburk Jr., H.H. 1966. Effect of four herbicides on growth of Rhizoctonia solani. Phytopathology 56:1332-1333.
Ross, J.P. 1962. Crop rotation effects on the soybean cyst nematode population and soybean yields. Phytopathology 52:815-818.
Ross, R. E., Keinath A. P., and Cubeta, M. A. 1998. Biological control of wirestem on cabbage using binucleate Rhizoctonia spp. Crop Protection 17:99-104.
Rothrock, C.S., Kirkpatrick, T.L., Frans, R.E., and Scott, H.D. 1995. The influence of winter legume cover crops on soilborne plant pathogens and cotton seedling diseases. Plant Disease 79:167-171.
Sanogo, S., Yang, X.B., and Scherm, H. 2000. Effects of herbicides on Fusarium solani f. sp. glycines and development of sudden death syndrome in glyphosate-tolerant soybean. Phytopathology 90:54-66.
Sarwar M., Kirkegarrd, J.A., Wong, P.T.W., and Desmarchelier, J.M. 1998. Biofumigation potential of brassicas: III. In vitro toxicity of isothiocyanates to soil-borne fungal pathogens. Plant and Soil 201: 103112.
Seth, D. 2001. Effect of inoculum, cultivar, and the biological control fungus Beauveria bassiana on damping-off caused by Rhizoctonia solani on tomato. M.S. Thesis. The University of Tennessee, Knoxville.
Seth, D., B.H. Ownley, R. Pereira, and C.H. Canaday. 2001. Effect of application method and inoculum form of Beauveria bassiana on Rhizoctonia damping-off in tomato. Phytopathology 91:S81.
Tomaso-Peterson, M., and Trevathan, L. E. 2004. Rhizoctonia solani AG-13 isolated from corn in Mississippi. Plant Disease 88:908.
Vierling, R.A., Faghihi, J., Ferris, V.R. and Ferris, J.M. 2000. Methods for conferring broad-based soybean cyst nematode resistance to a soybean line. United States Patent 6,096,944.
Villajuan-Abgona, R., Kageyama, K. and Hyakumachi, M. (1996) Biocontrol of Rhizoctonia damping-off of cucumber by non-pathogenic binucleate Rhizoctonia. European Journal of Plant Pathology 102:227-235.
USDA, NASS. 2005. Agricultural Statistics 2005. Chapter IV: Statistics of Vegetables and Melons. (http://www.usda.gov/nass/pubs/agr05/05_ch4.PDF)
USDA, NASS. 2004. Nursery Crops 2003 Summary.
(http://usda.mannlib.cornell.edu/reports/nassr/other/nursery/nurser04.pdf)
Westphal, A. 2005. Detection and description of soils with specific nematode suppressiveness. Journal of Nematology 37:121-130.
Workneh, F., Tylka, G.L., Yang, X.B., Faghihi, J., and J.M. Ferris. 1999. Regional assessment of soybean brown stem rot, Phytophthora sojae and Heterodera glycines using area-frame sampling: prevalence and effects of tillage. Phytopathology 89:204-211.
Wrather, J.A., Anand, S.C., and Koenning, S.R. 1992. Management by cultural practices. Pages 125-132 in: Biology and Management of the Soybean Cyst Nematode. R.D. Riggs and J.A. Wrather, eds. APS Press, St. Paul, MN..
Wrather, J.A., Koenning, S.R., and Anderson, T. R. 2003. Effect of diseases on soybean yields in the United States and Ontario (1999-2002). Online. Plant Health Progress doi:10.1094/PHP-2003-0325-01-RV.
Zhou, X. G., and Everts, K. L. 2004. Suppression of Fusarium wilt of watermelon by soil amendment with hairy vetch. Plant Disease 88:1357-1365.