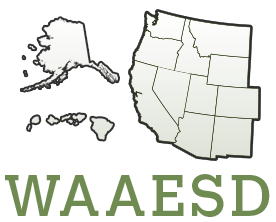
W1171: Germ Cell and Embryo Development and Manipulation for the Improvement of Livestock
(Multistate Research Project)
Status: Inactive/Terminating
W1171: Germ Cell and Embryo Development and Manipulation for the Improvement of Livestock
Duration: 10/01/2004 to 09/30/2009
Administrative Advisor(s):
NIFA Reps:
Non-Technical Summary
Statement of Issues and Justification
STATEMENT OF THE ISSUE:
The knowledge base for understanding the fundamental underlying biological mechanisms and principles of techniques used to enhance germ cell and embryo development and to produce genetically enhanced livestock is limited. Furthermore, the vast majority of these methodologies are extremely inefficient. The efficiencies of these technologies will have to be substantially improved if we are to benefit from the advantages of germ cell and embryo manipulation that can lead to increased food and fiber production. These advantages include the production of more desirable products, new products, value-added products, and increased efficiency of the utilization of natural resources.
JUSTIFICATION:
The development of transgenic animals used for food and fiber production has significant potential for consumers, animal producers, their communities, and our environment. Potential examples of such transgenic animals are those producing a milk containing human proteins to make a more desirable human baby formula, those producing a leaner, more desirable meat, or those more efficient in growth, reproduction, wool production, or milk production, including those with increased disease resistance (Wall, 2002; Wheeler et al., 2003). Increased efficiencies in production of animal products can be of economic benefit to both consumers and producers and have obvious advantages to the environment in terms of reduced use of natural resources.
Current procedures for the production of experimental transgenic animals can involve the use of in vitro oocyte maturation, in vitro fertilization, in vitro culture, cell culture and nuclear transfer either before or after gene transfer (Hasler et al., 1995, Wall, 2002). This is more practical than recovery of in vivo fertilized embryos, but is still extremely time and labor-consuming. Currently, more than 10 hours of labor are required to produce a single bovine embryo via nuclear transfer for transfer back to a recipient female. When this is coupled with a 1-5% pregnancy rate, an estimated 1000 hours are required to produce a single transgenic offspring. Before transgenic animals can contribute significantly to production systems, their production will have to be far more efficient. The inefficiencies occur during oocyte maturation, during nuclear transfer, during embryo culture and during establishment of pregnancy. Short and long-term storage of the transgenic embryos is necessary for efficient production of transgenic animals, but this technology needs improvement as well (Hurtt et al., 1999).
The details of meiotic maturation of oocytes (particularly the details of nuclear maturation) are beginning to be understood. Much of this work has been done with mouse oocytes; yet differences are known to exist between murine oocytes and bovine, porcine, or ovine oocytes (Cran and Moor, 1989). This is just one of the areas where the proposed multi-state research will contribute to animal production.
Although there have been recent advances in nuclear transfer technology in livestock and laboratory species (Wilmut et al., 1997; Schnieke et al., 1997; Wakayama et al., 1998; Eyestone and Campbell, 1999; Kuhholzer and Prather, 2000; Wheeler and Walters, 2001; Escriba et al., 2002; Niemann et al., 2003; Wang and Zhou, 2003; Mullins et al., 2004), much still needs to be learned regarding the biology and application of these methods to production of transgenic animals. This technology is very inefficient at present (Wilmut et al., 1997; Campbell, 1999; Niemann and Hues, 2000; Niemann and Rath, 2001; Paterson et al., 2003) and needs improvement before it can be widely used for livestock systems. Research to increase the practicality of making transgenic animals is directly in line with FAIR95 Goal 2 "Meet consumer needs in domestic and international markets for competitive and high-quality products from animals. Objective l. Increase efficiencies of production livestock. Objective 2. Enhance the quality of products from animals" (Anonymous, 1993). The economic significance of transgenic animals to U.S. animal agriculture in the future cannot be estimated with any confidence. However, the livestock and dairy industries generated over 68 billion dollars of on-farm receipts in 1992 (Anonymous, 1994). Even small effects on efficiency would repay research costs several times over.
A multi-state research approach is an extremely advantageous means to approach these problems efficiently. Alternative approaches can be tested in multiple laboratories, and the effective procedures further tested in the remaining laboratories. Oocyte and embryo procedures appear particularly laboratory dependent; for example, the optimal exposure time for vitrification of mouse oocytes and mouse blastocysts varied significantly among laboratories (Wood et al., 1993; Valdez et al., 1993; Zhu et al., 1993; Shaw et al., 1992). Improvements in nuclear transfer methods and the development of embryonic/ somatic cell lines to serve as nuclei donors are other areas that would benefit from this multiple laboratory approach.
The use of gene transfer alone or in combination with nuclear transfer is very useful for obtaining a variety of information. Some examples are insight into the cell cycle, nuclear and cytoplasmic programming or re-programming, genomic imprinting, gene expression, epigenetics and developmental processes to name just a few. This information can be used in studies to examine basic biological questions, biomedical questions, genetic questions and evolutionary questions as well as applications for agriculture.
This proposal will evaluate two areas critically important to the future success of animal biotechnology: 1) the understanding of the developmental biology and underlying biological mechanisms of fertilization and embryonic development and 2) the refinement of methods for production of genetically modified animals to improve livestock production efficiency.
Related, Current and Previous Work
RELATED REGIONAL PROJECTS:
Related regional research projects are NC-210 Mapping the Pig Genome, NCR-057 Reproductive Physiology, NEC-80 Biotechnology-Animal Development, and NC1006 Methods to Increase Reproductive Efficiency in Cattle. However, the work is complementary, rather than duplicative. It is assumed that any new multistate projects that may be duplicative of the proposed multistate project will not be approved.
The remaining description of current and previous work will be organized by objectives and references are typically to current reviews.
1). Understanding the developmental biology and underlying biological
mechanisms of fertilization and embryonic development.
Oocyte Maturation:
Use of in vitro matured oocytes in combination with in vitro fertilization for the production of livestock embryos in the laboratory is rapidly increasing (Yang et al., 1998; Telfer et al., 2000; Nagai, 2001; Coy and Romar, 2002; Tosti et al., 2002; Miyano, 2003; Zeuner et al., 2003). These techniques have not only tremendous research value in studying the basic biological events that occur during oocyte maturation, fertilization and early embryonic development, but also provide an inexpensive and readily procurable source of preimplantation livestock embryos. Successful maturation, fertilization and subsequent embryonic development depend heavily on initial oocyte quality. Procedures that could accurately determine an oocytes viability and its likelihood to fertilize and develop as an embryo following in vitro maturation would dramatically improve the efficiency of in vitro embryo production. Alternatively, reliable methods for assessment of viability of in vivo- or in vitro-derived embryos would permit screening of embryos prior to manipulation or transfer procedures. Linking these indicators of embryo viability and successful development with the expression and function of specific genes could also afford valuable insights into mechanisms contributing to embryonic mortality.
An integral aspect of in vitro embryo production is successful oocyte maturation. Oocyte maturation is dependent on two separate events: nuclear maturation and cytoplasmic maturation (Fulka et al., 1998). Nuclear maturation refers to the progression of the oocyte from the dictyate stage of meiosis I to metaphase II and extrusion of the first polar body. The process of cytoplasmic maturation appears to be the critical factor that determines the success of producing viable embryos. Determining and quantifying the indicators of cytoplasmic maturation in the oocyte would enable more efficient selection of oocytes for fertilization and embryo development in vitro.
Involvement of cyclin and p34cdc2 kinase in nuclear maturation has been convincingly demonstrated in a number of species (Cran and Moor, 1989, Polanski et al., 1998). Other details of nuclear maturation are yet to be understood and exhibit some species variation.
The biology of cytoplasmic maturation is even less well understood (Krisher and Bavister, 1998; Bevers and Izadyar, 2002; Lindsey et al., 2002; Krischek and Meinecke, 2002; Armstrong et al., 2003). The anatomy of the oocyte changes, including the development of microtubule organizing centers and the migration of intracellular organelles (Mattson and Albertini, 1990). The ability of the oocyte plasma membrane to fuse with sperm increases with increasing maturity, at least in the hamster (Zuccotti et al., 1991).
There appears to be a lack of synchrony between nuclear and cytoplasmic maturation in vitro. Porcine oocytes may achieve nuclear maturation to metaphase II after as few as 24 hours of culture, but do not possess developmental competence until over 40 hours of maturation (Grupen et al., 1997). It is possible to prevent spontaneous nuclear maturation by the addition of dibutyryl cAMP (Nekola and Smith, 1975) or roscovitine ((Franz et al., 2003) to the maturation medium, thus allowing more time for cytoplasmic maturation while avoiding nuclear aging. Developmental competence might be improved further by delaying nuclear maturation for a longer period of time because oocyte maturation in vivo requires several days as compared with the 42 to 44 hour maturation period typically reported for porcine oocytes.
Studies have indicated that morphological characteristics such as appearance of cumulus cells, oocyte size, and the time of polar body extrusion are related to the ability of oocytes to fertilize and develop into viable embryos (Younis al., 1989; Dominko and First, 1992). There are distinct morphological differences between in vivo and in vitro matured porcine oocytes (Wang et al., 1998). The slow or incomplete cortical granule release following sperm penetration (Wang et al., 1997) and uneven distribution of cortical granules within the oocyte (Wang et al., 1998) might be related to the high incidence of polyspermy reported for porcine oocytes matured and fertilized in vitro. Overall, the limited developmental competence of porcine oocytes matured in vitro appears to be related to failure of adequate cytoplasmic rather than nuclear maturation (Nagashima et al., 1996).
Equine oocytes require a longer in vitro maturation period than bovine oocytes (Shabpareh et al., 1993). Preovulatory follicular fluid before the LH surge inhibits maturation, while by 20 hours after the LH surge, follicular fluid stimulates oocyte maturation, even in the presence of inhibitory factors (Romero and Seidel, 1994). Prochazka et al. (2000) found that medium supplemented with either EGF or FSH could equally support nuclear maturation and cumulus expansion of porcine oocytes isolated from follicles 6 to 7 mm in diameter.
Fertilization and Sperm Capacitation:
Fertilization and initial cleavage occur in the mammalian oviduct. Although in vitro production and development of farm animal embryos have become routine, their development in vitro remains far below in vivo embryos. The secretion of several factor(s) from the mammalian oviduct may contribute to the superior development of in vivo produced embryos. A high molecular weight oviductal glycoprotein (OGP) is synthesized and secreted by the secretory cells of the oviduct. The presence of OGP is highest during the periovulatory period and declines thereafter, suggesting its potential role during in vivo fertilization and possibly during the initial cleavage stages (Malette et al., 1995; Verhage et al., 1998).
As part of the cytoplasmic maturation of oocytes, the protein composition of the oocyte plasma membrane changes including an increased ability to bind and/or fuse with sperm (Zuccotti et al, 1994; Cohen et al., 1996). Oocyte receptors for the sperm plasma membrane are either described/ hypothesized as the complementary receptors for putative ligands or in one case as integrin heterodimers. Wheeler et al. (1996) have verified that bovine oocytes increase their ability to bind sperm plasma membrane during the 22 hours of in vitro maturation and that this increase is dependent upon protein synthesis. Recently, a putative oocyte plasma membrane protein (POMP) receptor for sperm was identified (Berger et al., 1998; 1999; Yen and Berger, 1999).
Sperm gain the ability to bind and fuse with the oocyte plasma membrane in the equatorial segment during capacitation. Modification of one or more of the ligands or exposure of one or more of the ligands for the oocyte plasma membrane would appear to be an essential component of capacitation. A number of potential sperm ligands has been proposed to interact with the oocyte in different species; species specificity (at least among mammalian orders) would be expected. Ash et al. (1995) identified four predominant binders of the oocyte plasma membrane as potential porcine sperm ligands for the porcine oocyte plasma membrane.
Assisted Fertilization:
It has been shown that capacitation status of bovine sperm for intracytoplasmic sperm injection (ICSI) is irrelevant, and that there is a benefit of activation of oocytes with the calcium ionophore A23187 (Chen and Seidel, 1997). Further, a beneficial effect by activating equine oocytes with ICSI has been shown (Kato et al., 1997). A novel method of increasing fertilization rates in vivo via a sperm-oocyte adhesive peptide (Amann et al., 1999) may also be promising in vitro.
Sex Determination:
The ability to pre-select the sex of offspring would have tremendous economic benefit to beef and dairy producers alike. One approach has been to attempt to physically separate X- from Y-chromosome bearing spermatozoa using modified flow cytometry (Johnson et al., 1994). However, this procedure does not have widespread application at the present time, due to the cost of the equipment and limitations on sorting rate (Seidel and Garner, 2002).
It may be possible to significantly alter the sex ratio by screening and then selecting, ejaculates with a naturally altered ratio of X- to Y-bearing spermatozoa. Chandler et al. (1998) reported that within sires the ratio of X- to Y-bearing spermatozoa from different ejaculates of semen can be skewed significantly toward one sex, and this skewed ratio appears to translate into altered sex ratio of offspring. The ratio of X- to Y-chromosome bearing spermatozoa of ejaculates within bulls was found to be significantly skewed (~84%) in about 20% of the ejaculates evaluated. However, there is considerable controversy surrounding these findings. Studies are needed to confirm that the ratio of X- to Y bearing spermatozoa is skewed in some ejaculates of semen.
Embryo Development:
Although the methodology for maintaining mammalian livestock embryos in culture has existed for many years, the ability of the present systems to support normal development is limited. This is especially true of early embryos that often exhibit developmental blocks in vitro (Krisher and Bavister, 1998). These blocks are thought to be the result of inadequate culture systems since similar blocks are not found in vivo. In addition, numerous studies have shown that there is a decline in embryo viability corresponding to the length of time they are held in culture (Davis, 1985). More recently, co-culture systems have been developed to at least partially overcome the deficiencies associated with earlier systems (Trounson et al., 1994). The benefits of co-culture systems in promoting in vitro development are well established, but the mechanisms by which these benefits are achieved have yet to be elucidated. Evidence is accumulating that growth factors have an autocrine and paracrine role in embryo development (Gandolfi, 1994; Heyner et al., 1994, Glover and Seidel, 2003). Tremendous effort will be required to define these effects, but secretion of growth factors by the co-cultured cells may be at least one of the mechanisms for the beneficial effect of co-culture (Winger et al., 1997).
Experiments have confirmed the detrimental effects of high oxygen and beneficial effects of certain antioxidants on in vitro development of bovine embryos (Caamaqo et al., 1996, 1998; Olson and Seidel, 2000). Optimal ways have been determined to condition media with buffalo rat liver cells for culturing bovine embryos (Funston et al., 1997). Studying gene expression in elongating bovine embryos has shown the IGF system to have important regulatory roles (Keller et al., 1998). One of our stations (Colorado) has identified several genes with increased expression as embryos elongate (Glover and Seidel, 2003).
Extracellular matrix (ECM)-degrading proteinases are believed to play pivotal roles in early embryo development and embryo-uterine interactions (Cross et al., 1994). ECM-degrading proteinases include the plasminogen activator (PA)-plasmin system and the family of matrix metalloproteinases (MMP) (Vassalli and Pepper, 1994). The laboratory of one of our contributing stations (Oregon) has demonstrated that endodermal cells express PA during migration in vitro in sheep and cattle. During the periods of endodermal cell migration and trophoblastic expansion and elongation, ovine embryos express PA, MMP-9 and three MMP inhibitors (TIMPs-1, -2 and -3).
Blastocyst production by in vitro methods has plateaued at around 40% despite various attempts to improve culture conditions. The 40% development rate falls short of the 85 to 95% that occurs in vivo. Various attempts have been made by using co-culture systems, semi-defined mediua, or chemically defined media in a variety of culture conditions; however, in vitro production of blastocysts still hovers at or below 40% (Thibodeaux et al., 1992; Choi et al., 1998; Krisher and Bavister, 1998). Furthermore, in vitro-produced blastocysts are usually developmentally retarded with fewer inner cell mass cells compared with embryos developed in vivo. The apparent lack of biologic culture conditions necessitates further innovation in tissue culture methodology and the pursuance of further research in this area.
Epigenetic refers to changes in gene expression that occur without a change in DNA sequence. Two key factors are associated with the epigenetic control of gene expression 1) DNA methylation and 2) histone modifications which include methylation, phosphorylation and acetylation (Bird and Wolffe, 1999; Jenuwein and Allis, 2001). Shortly after fertilization, mammalian embryos undergo genome-wide epigenetic reprogramming by demethylation during preimplantation development followed later by de novo remethylation (Monk et al., 1987; Dean et al., 2001). This epigenetic reprogramming is essential for normal development to proceed. Aberrant reprogramming has been clearly linked to failed embryonic development. (Dean et al., 2001; Howlett and Reik, 1991; Shi and Haaf, 2002). Moreover, there is growing body of evidence which, suggests that inadequate epigenetic reprogramming during early mammalian development may result in large offspring syndrome (LOS) and other developmental abnormalities (Young et al., 1998; Hill et al., 2000a; Sinclair et al., 2000). Clearly, understanding the factors and mechanisms which control epigenetic reprogramming during early mammalian development are of critical importance
2). Refinement of methods for production of genetically modified animals to
improve livestock production efficiency.
Nuclear Transfer:
Nuclear transfer, which involves the transfer of each nucleus from a multicellular embryo into an enucleated metaphase II oocyte, has been developed in several species (Willadsen, 1986; Prather et al., 1987; Stice and Robl, 1988.). Nuclear transfer has become an active field of research over the last decade, culminating in reports of live offspring from differentiated cells of sheep, cattle, and mice (Schnieke et al., 1997; Wells et al., 1997; Cibelli et al., 1998; Kato et al., 1998; Wakayama et al., 1998). Dolly was not a fluke. Dozens of laboratories are producing calves and lambs whose genetic parents are somatic cells, often derived from adults (Cibelli et al., 2002). This technology has three broad applications: 1) applied animal breeding to copy outstanding animals (e.g., proven bulls), 2) a tool for basic research, (e.g., mechanisms of differentiation), and 3) a biotechnological tool (e.g., vastly simpler approaches to making transgenic farm animals).
Although offspring have been produced in livestock using nuclear transplantation, the efficiency rate has been low (Wilmut et al., 1997). In each of the species reported to date, the efficiency is ? 6% (1-6% in sheep, 1-4% in cattle, and 1% in pigs). The factors affecting the efficiency of nuclear transplantation are: enucleation of the recipient oocyte, fusion of the transplanted nucleus to the enucleated oocyte cytosol, activation of the oocyte, and "re-programming" of the transferred nucleus. Activation may be the factor responsible for the greatest loss in efficiency (Collas and Robl, 1990). However, the general consensus is that nuclear transfer efficiency is much too low to be economically viable except in very limited applications. The low efficiency also makes it an expensive research tool. Any increase in efficiency will greatly enhance the value of this technology and will contribute to our understanding of changes that must occur in chromosomes to allow embryonic gene expression patterns. It is also critical to begin to understand how potentially subtle modifications in the nuclear structure impact the ability of a cell to contribute to production of offspring and yet another to fail.
An important aspect of this field, in the past and the future, concerns understanding the abnormalities of offspring produced by nuclear transplantation (Seidel et al., 1997; Garry et al., 1998). Such calves and lambs have high rates of fetal and neonatal death. At birth, they are hypoxic, hypoglycemic, hypothermic, and readily die (Garry et al., 1998). It has been hypothesized that these animals have a genetically or epigenetically abnormal placenta, since the calves themselves appear normal genetically (Garry et al., 1996).
Transgenic Methodologies:
The ability to produce novel proteins in the mammary gland of domestic animals will have an important impact on agriculture. With the ability to use genetically enhanced somatic cells as nuclear donors, this objective appears to be attainable at rates which will allow use of domestic animals. Several years ago one of our contributing stations (Colorado) combined transgenics with in vitro-produced bovine embryos (Thomas et al., 1993), and produced the first transgenic calf demonstrated to, in fact, express a transgene (Bowen et al., 1994). Additional previous work has included the production and study of transgenic mice. Targeted milk properties include processing functionality (Maga et al., 1995; Gutierrez-Adan et al. 1996), anti-microbial properties (Maga et al., 1997), and the application of antisense constructs to obtain efficient down-regulation of protein secretion into the milk (Sokol et al., 1998).
Cryopreservation:
Cryopreservation of in vivo produced bovine embryos is quite successful and limited success has been achieved with other species such as late-stage porcine embryos (Nagashima et al., 1994; Dobrinsky, 1997). Initially, in vitro matured and fertilized embryos appeared more susceptible to damage during freezing and thawing (Leibo and Loskutoff, 1993, Arav et al., 1996). Alterations in in vitro maturation and culture can affect the susceptibility of the in vitro-produced oocytes to cryo-damage. The large equine embryo (routinely collected non-surgically) is particularly challenging to cryopreserve. Recently, a new concept was developed, step-down equilibration, to cryopreserve such embryos successfully (Young et al., 1997). Recently, equine and bovine oocytes have successfully been vitrified (Hurtt et al., 1999), and matured oocytes survived vitrification better than immature ones.
Stem Cell Biology:
Transgenic animals have great potential for livestock production, but the potential is still unrealized. The low efficiency in their production is a primary reason (Ebert and Schindler, 1993; Halter et al., 1994). These inefficiencies occur at all stages. An aspect of great importance in this regard is the isolation and manipulation of undifferentiated, pluripotent embryonic cells. There are two types of pluripotent embryonic cells, embryonic stem (ES) cells and embryonic germ (EG) cells. ES cells are isolated in culture from blastocyst-stage embryos, and EG cells are isolated from cultured primordial germ cell (PGC).
ES cells first were isolated from mouse embryos (Evans and Kaufman, 1981; Martin, 1981) and since have been used as a model for mammalian embryogenesis and more recently for genetic manipulation. Due to their ability to integrate foreign DNA and thereafter differentiate into any and all tissues of a normal individual, ES cells are effective vehicles for genetic engineering and for creating laboratory animal models of human diseases. Despite considerable effort, progress toward isolation of ES cells from agricultural species has been slow. Some of the more promising results have been achieved with pigs (Wheeler, 1994). To date, production of embryonic stem cell lines has been much more difficult in livestock species than in mice (Sims and First, 1994; Rund et al., 1996; Wheeler and Choi, 1997a; Iwasaki et al., 2000; Talbot et al., 2002; Denning and Priddle, 2003; Bonde et al. 2003; Wheeler and Malusky, 2003).
In the early 1990s, several laboratories reported isolation of ES-like cells from cultures of primordial germ cells (PGCs)(Matsui et al., 1992; Resnick et al., 1992). These cells were called EG cells to distinguish them from blastocyst-derived ES cells, but EG and ES cells appear to share many characteristics, including the ability to differentiate into numerous cell types. One of our contributing stations (California) successfully isolated EG cells from porcine PGC (Shim et al., 1997, Shim and Anderson, 1998), the first example in a species other than the mouse. Recent successes with isolation of ES and EG cells from agricultural species have been exciting, but generally these ES and EG cells have a low efficiency of incorporation into normally developing embryos. Moreover, in no instance have livestock ES or EG cells been shown to develop into gametes, a prerequisite to their use in genetic engineering. Several laboratories involved in the W-171 project have a history of productive research with ES and EG cells. By combining the resources, ingenuity and collaborative efforts from these laboratories, this Regional Research Project could yield undifferentiated cell lines of practical value to animal agriculture, a phenomenal contribution to animal agriculture.
Objectives
-
Understand the biology and underlying mechanisms of gamete development, fertilization and embryogenesis.
-
Refine methods for production of genetically modified animals to improve livestock production efficiency.
-
Methods
Objective 1: Understand the biology and underlying mechanisms of gamete development, fertilization and embryogenesis. The overall aim of the research to be conducted under this objective is to gain a more precise understanding of the biological requirements for successful oocyte maturation, fertilization, and subsequent embryonic development. The production of live offspring is dependent upon all of these events occurring in a well-orchestrated fashion. Arkansas, Iowa and Illinois will collaborate on investigations on in vitro production of porcine embryos. Porcine oocyte-cumulus complexes (OCC) will be recovered from the ovaries of abattoir gilts and matured for varying lengths of time in medium supplemented with various combinations of serum, FSH, estradiol, insulin and dibutyryl cAMP. A portion of OCC will be removed for evaluation of germinal vesicle breakdown, while remaining OCC will be matured for an additional 12 to 24 hours. Oocytes will be evaluated for nuclear maturation and electrically activated to assess parthenogenic development. The best treatment/time combination will be used for IVF to evaluate oocyte developmental competence and rate of polyspermy. Indiana will investigate the role of carbohydrate and fat metabolism during oocyte maturation in the control of nuclear maturation, as well as the acquisition of developmental competence. California will examine the presence of a putative oocyte plasma membrane protein (POMP) receptor before and after partial in vitro maturation with and without cycloheximide to inhibit protein synthesis. Oocytes will be examined using confocal microscopy, and the intensity of fluorescence will be measured and analyzed. In vivo heat stress will be used to reduce porcine oocyte quality to further evaluate POMP as a marker for oocyte quality. In a related study, porcine ovaries will be fixed, embedded in paraffin, and subjected to immunohistochemistry to examine the presence of POMP. Colorado will use in vivo maturation of oocytes in large follicles and in vitro maturation of oocytes from small follicles. Oocytes in the process of in vivo maturation in pre-ovulatory follicles will be recovered from cattle and horses by transvaginal ultrasound-guided aspiration at various times after giving an LH or hCG injection. At the onset of maturation, and at about 30 and 90% percent through normal maturation times for the respective species, mRNA will be quantified in oocytes within each species. There will be 3 approaches: microarrays, suppressive subtractive hybridization and candidate genes. The microarrays to be used will depend on what is available at the time for each species (or across species). mRNA for approximately 100 candidate genes (e.g., cell cycle regulatory molecules, genes implicated in regulating oocyte maturation) will be quantified by Real Time (RT) PCR. Indiana will also investigate genes involved in developmental competence using bovine microarrays by comparing in vivo versus in vitro matured ovine oocytes. Connecticut and Louisiana will use bovine oocytes from pre-pubertal ovaries for studies on in vitro maturation. Oocytes will be matured in vitro using standard procedures, except that chemically defined media will be used for most studies (Olson and Seidel, 2000). The dose-response of gonadotropins, of decreasing sodium ion , and of sulfhydryl-donating agents such as glutathione, cysteamine, and beta-mercaptoethanol; addition of amino acids present in oviductal and follicular fluid; benefits of the growth factors EGF and IGF-1 will be the main topics studied. Louisiana and Colorado will collaborate on methods for in vitro fertilization of equine oocytes via intracytoplasmic sperm injection. Joint efforts are planned to adapt ICSI technology for in-field use in the cow (Colorado, Oklahoma, Louisiana), small ruminants (Louisiana, Iowa) and swine (Illinois). Colorado will use the new piezo-injection technology for ICSI in cattle and horses, as well as injection of the sperm activating protein that causes oscillations of free intracellular Ca++ in oocytes (Fissore et al., 1998). Colorado will study methods of attaining physiological bovine oocyte activation including injecting IP3 and other agents affecting this pathway, coinciding with studies conducted by Utah where mechanisms associated with activation of bovine oocytes will be evaluated. The potential role of integrins and disintegrins in the interactions between the sperm and oocyte; any association between integrin associated intracellular calcium transients and either IP3 or ryanodine receptors and their possible link with specific integrin subunits found on the surface of bovine oocytes will be the focus of these investigations. Collaborative studies will be conducted on in vitro sperm capacitation because capacitation is needed for optimal in vitro production of embryos. California will determine, using flow cytometry, if bovine sperm with varying abilities to interact with oocytes vary in the binding of the DRKD antibody to the sperm surface, either initially or after normal in vitro capacitation. Initial comparisons will be made among samples within a single laboratory, but subsequent studies will focus on semen samples obtained from collaborating stations (Colorado, Louisiana, Utah). Fresh, frozen and epididymal sperm, and techniques for capacitation, will be studied by several stations (California, Colorado, Iowa, Illinois, Louisiana) for use in IVF procedures in various farm animal species. California and Illinois will investigate porcine sperm, California and Colorado will study bovine sperm, and Colorado, Iowa, and Louisiana will examine equine sperm. Prior research (Louisiana) has identified differences among species, which need further characterization. A major collaborative effort will focus on fertilization and subsequent development of in vitro matured bovine oocytes. All oocytes will originate from a single source (Wisconsin) and will be shipped to participating stations (Colorado, Connecticut, Iowa, Louisiana, Oklahoma, and Utah) for subsequent investigations. To the best of our knowledge, this will be the first time that oocytes from a common source will be utilized in such a manner. This uniformity in pre-fertilization conditions will enable a more clear-cut interpretation of experimental results obtained across stations. (Likewise, this approach affords the opportunity to investigate various methods of oocyte maturation on subsequent embryonic development using standardized protocols for fertilization and culture.) Preimplantation embryo development in vivo depends on the interaction between the embryo and the oviductal/uterine environment. Colorado, Louisiana, and Utah will investigate the role of various glycoproteins on embryonic development. Colorado plans to study the secreted oviduct glycoprotein that binds to oocytes (Verhage et al., 1998) and a seminal plasma peptide, Fert+, that recently was shown to improve fertility of subfertile bulls with artificial insemination (Amann et al., 1999). Utah will study changes in protein profiles (i.e., type, amount and distribution) of OVEP and UEC co-cultures. They will use electrophoresis to investigate kinetic changes of protein patterns, and immunocytochemistry and FISH techniques will be used to detect changes of specific proteins (receptors). Colorado and Iowa will investigate the role sulfhydryl-donating agents such as glutathione, cysteamine, and beta-mercaptoethanol in embryonic development using a chemically defined medium (Caamano et al., 1998; Olson and Seidel, 2000), and Colorado will continue their studies with various antioxidants such as vitamin E and fructose (Chung et al., 1996). Colorado will examine differences between in vitro produced and in vivo derived embryos in the metabolism of glucose occurring through glycolysis, the Krebs cycle, or the pentose phosphate pathway (PPP). Radiolabeled glucose will be followed through the metabolic pathways for both in vivo- and in vitro-produced embryos at both days 5 and 7 of gestation. Endpoints will include lipid content of embryos (Abe et al., 2002), freezability of embryos (Stojovic et al., 2002), and pregnancy rates including normality of calves. Subsequent studies will examine manipulation of metabolism with various pharmacological agents to make in vitro-produced embryos more normal. Indiana will continue to evaluate the normality of porcine embryos produced in vitro using metabolism of glucose, pyruvate and glutamine as measures of viability. Oregon plans to evaluate expression of components of the PA-plasmin system and the MMP during the periods of endodermal cell migration from the inner cell mass, formation of the bilayered trophoblast, and trophoblastic expansion and elongation in sheep and cattle embryos. They intend to determine the complete phenotypic expression pattern and the cellular localizations of the ECM-degrading proteinases and their associated inhibitors during these periods of development. Texas will attempt to identify and characterize the factors and mechanisms controlling epigenetic reprogramming during bovine oocyte maturation and pre-implantation development, as well as attempt to silence the expression of DNMT genes during bovine pre-implantation development and its subsequent effect on epigenetic reprogramming and embryo/fetal development. Novel approaches to in vitro culture will be investigated by Arkansas, California, Colorado, Illinois, and Louisiana. Colorado will attempt to determine the reasons for enhanced development during submarine culture (Vajta et al., 1997). Illinois has devised a new micro-fluidic embryo culture device, and this will be used at six other stations (Arkansas, California, Colorado, Connecticut, Indiana and Iowa) for studies on in vitro maturation and fertilization of porcine oocytes, as well as embryonic development. The stations will initiate experiments evaluating sequential addition of sperm aliquots at fertilization as a proposed means to reduce polyspermy (California, Illinois and Iowa) in a M199-based, Tris-based (Linfor and Berger, 1999; California), or mTBM-based fertilization medium (Illinois). Arkansas will take the lead in evaluating seeding the microfluidic chamber with epithelial cells and altering medium composition frequently during development/fertilization/ maturation. Oregon, Utah, Illinois, Maryland, Nevada and Colorado will investigate gene expression in developing pre-implantation embryos. Colorado will characterize the genomic organization and functions of genes that are expressed in greater amounts in day-17 than in day-15 bovine embryos, and gene expression between in vitro and in vivo embryos will be compared using differential display (Glover and Seidel, 2003). Illinois will characterize the gene and protein expression of porcine embryos cultured under various conditions using RT-PCR, real-time PCR and capillary electrophoresis. Oregon will collect embryos from superovulated donor cows and ewes, and RT-PCR will be used for determining the phenotypic expression pattern of the genes of interest. Localization of the cells expressing the specific genes will be conducted using in situ hybridization. For experiments involving analysis of secreted proteins by cultured embryos, isolated inner cell masses or trophoblast, conditioned medium will be recovered and analyzed by immunoblotting and casein and gelatin zymography. Utah will also use RT-PCR to trace sequential gene expression during embryo development. Arkansas, Colorado, and Iowa will be involved with studies on offspring sex ratios. Arkansas will investigate, using X- and Y-specific DNA probes in conjunction with FISH (Hassanane et al., 1998), if a naturally occurring skew in the ratio of X- to Y- chromosome bearing spermatozoa occurs among ejaculates, and, if so, will study factors that potentially influence the altered ratio. Seminal plasma will be evaluated for components that might be related to altered sex ratio. Ejaculates with a skewed sex ratio will be used for IVF to confirm that altered sex ratio of semen translates into altered gender ratio of embryos that will be sexed using commercially available probes. Iowa will continue studies on the use of a vaginal conductivity probe to pinpoint an insemination time that may lead to an altered calf sex ratio. In an effort to produce excellent quality oocytes for IVF, collaborative efforts are planned to evaluate protocols to maximize follicular growth and oocyte collection by transvaginal ultrasound-guided oocyte recovery (Louisiana, Illinois, Iowa, Connecticut). These studies will focus primarily on prepuberal, pregnant, and postpartum females. One station (Louisiana) has offered to train personnel from other stations to enhance collaborative efforts among the contributing stations. A joint effort is planned to evaluate an electronic heat detection system (Heat Watch) in different regions (Louisiana, Illinois, Iowa, Utah, Connecticut) using dairy and beef females. The initial focus of this research will be to evaluate differences in the onset and duration of behavioral estrus in similar genotypes during different climates/seasons of the year. Ultimately, these data will be used to predict the best time for transvaginal oocyte collection that will optimize the number of oocytes collected from each donor female. Objective 2: Refine methods for production of genetically modified animals to improve livestock production efficiency. The overall aim of the research to be conducted under this objective is to enhance the success of each step that is required for successful production of genetically enhanced embryos and offspring. Continual advances in gene transfer techniques and nuclear transfer methodology have led to a crucial need for more research that will lead to increased incorporation of these methods into livestock production systems. Colorado, Louisiana, Connecticut, Illinois and Utah will conduct studies on nuclear transfer. Utah will investigate methods for increasing activation rates of bovine nuclear transfer embryos, as well as study the developmental potential of hybrid (sheep-cow) nuclear transfer embryos to produce viable offspring. Utah will evaluate the ability of natural RGD-containing peptides (disintegrins/ADAMS) to induce, a) intracellular calcium transients, b) subsequent parthenogenetic development and, c) to determine if their presence in fertilization medium can disrupt fertilization by the sperm. Further, Utah will carry out experiments to better understand the specificity of the RGD - receptor interaction and the potential impact of variations in adjacent amino acids. Finally, Utah will try to identify the disintegrin/ADAM present on the surface of the sperm that mediates the cell surface intracellular calcium response. Utah, Louisiana, Illinois, Connecticut and Colorado will investigate the use of somatic cells for nuclear transfer. Louisiana and Illinois will develop methodology to establish caprine, ovine, equine, and bovine somatic cell lines that are useful for nuclear transfer, while Colorado will optimize methods of transplanting nuclei from fetal fibroblasts and cumulus cells into activated enucleated equine and bovine oocytes. Connecticut will test the nuclear transfer efficiency of different donor cell types for nuclear transfer and explore the possibility of transfecting cultured cells for transgenesis. Utah will investigate the use of somatic cell lines (embryonic, fetal and adult) from domestic animal species as vectors for the production of transgenic animals using nuclear transfer. California, Louisiana and Colorado will study the normalcy of embryonic and fetal development in vitro and in vivo because it has been well documented that a portion of the nuclear transfer pregnancies spontaneously abort and a portion of offspring have abnormalities. Colorado will continue to search for genetic or epigenetic changes in cloned embryos vs. control embryos. They will examine the placenta by using real-time RT PCR to compare differences in mRNA content of various genes in the cotyledons of cloned vs. IVF vs. control day-45 pregnancies (collected by a novel, non-surgical procedure and day 15 pregnancies). Differential expression of candidate genes will be confirmed by Northern blots; genes will be cloned, sequenced, and identified and/or characterized. California, Colorado, Connecticut, Illinois, Iowa, Louisiana, Maryland, and Utah will investigate techniques for the efficient production of transgenic livestock. California and Illinois will test in vivo differentiation of porcine ES and EG cells after being combined with embryos of various developmental stages. In addition, Illinois will test in vivo differentiation of porcine ES cells in the SCID mouse tumor system. Porcine cell lines that have been screened for markers of undifferentiated cells and that have been shown using in vitro differentiation tests to be pluripotent will be tested for in vivo differentiation. California will share cell lines among participating laboratories in order to test them under varying conditions. Iowa will investigate methods for more efficient isolation of porcine primordial germ cells. Maryland will explore the isolation, characterization and in vitro differentiation of goat embryonic-derived stem cell lines. Colorado, Connecticut, Utah, Illinois and Louisiana will conduct studies with transfected cell lines. Colorado will conduct experiments with cattle and sheep and will use green fluorescent protein as a reporter gene to study placental expression driven by the regulatory regions of molecules such as placental lactogens, and interferon tau. Illinois will conduct transfection experiments with the bovine alpha-lactalbumin gene and promoter to drive mammary expression of IGF-I and beta 1,4 galactosyltransferase. Similarly, Utah will transfect somatic cell lines with genes designed for expression of foreign proteins in the mammary gland and for enhancement of the resistance of animals to disease of economic importance to agriculture. Louisiana and Maryland will conduct transfection studies using embryo-derived stem cell lines from goats and cattle. Maryland and Illinois will explore the feasibility of inducing the expression of pluripotency determining factors to increase the efficiency of isolation and selection of embryonic stems cells in domestic species. California and Illinois will work towards a better understanding of the cell regulatory mechanisms underlying exogenous DNA integration, leading to the manipulation of factors responsible for the low efficiency of gene targeting. Californias specific goal is to test the hypothesis that selected recombinases can be manipulated to intermediate DNA pairing and strand exchange, directing cells preferentially to use the HR pathway over non-homologous end joining. Colorado, Iowa, Louisiana, and Maryland will investigate methods for cryopreservation of oocytes and embryos. Colorado, Louisiana, Iowa, and Maryland will investigate the potential use of vitrification for the freezing of mammalian oocytes and embryos. Louisiana will compare conventional and vitrification methodologies for the cryopreservation of oocytes from the mouse, goat and cow, while Colorado and Maryland will study vitrified oocytes (cattle, goats, and horses) using cryo-loops by evaluating fixed and stained specimens (Hurtt et al., 1999) and cleavage and blastocyst formation after IVF. Louisiana and Iowa will evaluate various non-conventional apparatuses (e.g., electron microscope grids) for their ability to enhance the cooling rate during the cryopreservation process. Several stations will work to develop efficacious methods of vitrifying oocytes. At various times during in vitro maturation, oocytes will be vitrified using the cryo-loop method (Checura and Seidel, 2003). Various agents that cause solutions to vitrify including macromolecules such as Ficoll, albumin, and various mixtures of intracellular cryoprotectants such as ethylene glycol, glycerol, and formamide will be studied. Colorado and Iowa will investigate cryopreservation of equine and porcine blastocyst stage embryos. Colorado will expand step-down equilibration studies with large equine blastocysts (Young et al., 1997), compare concentrations of glycerol and ethylene glycol, investigate whether the step-down procedure before freezing is beneficial. Iowa will examine the biophysical properties of porcine blastocysts in an attempt to develop a suitable method for cryopreservation of intact, non-manipulated porcine blastocysts. Gene expression in oocytes and embryos will also be examined by several stations (Colorado, Illinois, Maryland, Indiana and Nevada). Maryland will examine the onset of expression of pluripotency determining factors in embryos from several species, including goats, bovine and mice. Comparison of expression between in vivo and in vitro produced embryos will be made to determine whether differences of expression of these factors affect overall embryonic development and viability. Genes involved in oocyte quality will be examined in ovine and porcine oocytes using serial analysis of gene expression (SAGE), as well as microarrays. Populations of oocytes with high and low developmental potential will be compared, such as in vitro and in vivo matured oocytes, oocytes from pre-pubertal gilts versus sows, and oocytes from small and large follicles. Genes identified will be verified using real time quantitative PCR. In this way, genes critical for cytoplasmic maturation and developmental potential can be identified and further characterized.Measurement of Progress and Results
Outputs
- peer-reviewed journal articles/book chapters (n=307 during current project period 1999-2003)
- non-reviewed technical publications (n=162 during current project period 1999-2003)
- abstracts (n=283 during current project period 1999-2003)
- miscellaneous publications (n=64 during current project period 1999-2003)
- development of a commercially viable microfluidic embryo culture device
- Output 6: development of enhanced methods for nuclear transfer Output 7: refinement of techniques for cryopreservation of oocytes and embryos Output 8: enhanced system for in vitro production of embryos
Outcomes or Projected Impacts
- improved methods for nucleartransfer will impact the seedstock livestock industry as well as biomedical research companies
- microfluidic embryo culture devices will impact commerical embryo transfer firms as well as human infertility clinics
- enhanced in vitro embryo production systems will impact purebred livestock producers as well as human in vitro fertilization practices
- improved methods for oocyte and embryo cryopreservation will enable development of oocyte and embryo banks, increase utilization of frozen embryos in commercial embryo transfer firms, as well as aid efforts to preserve endangered species
- greater knowledge of livestock stem cell biology will provide alternative means to create genetically enhanced livestock