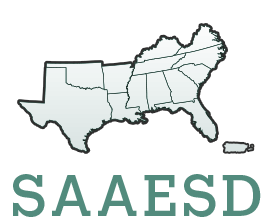
S1002: New Technologies for the Utilization of Textile Materials (S272)
(Multistate Research Project)
Status: Inactive/Terminating
S1002: New Technologies for the Utilization of Textile Materials (S272)
Duration: 10/01/2001 to 09/30/2006
Administrative Advisor(s):
NIFA Reps:
Non-Technical Summary
Statement of Issues and Justification
Project's Primary website is at http://msa.ars.usda.gov/la/srrc/csrees/main.htm (direct link can be found under LINKS)
The U.S. textile industry is a world leader in manufacturing textiles, employing more than 600,000 people in nearly every state and generating $69 billion annually (113). In order for the U.S. textile industry to maintain its leadership and competitive edge while preserving the environment, new technologies are needed to convert agricultural residues and by-products into textile products and to enhance the technical performance of conventional textiles for new consumer, medical and industrial applications. This new millennium project focuses on expanding the uses for agricultural commodities and by-products, investigating more environmentally friendly methods for textile processing utilizing biotechnologies, and developing novel, high performance textile products for specialty applications. The research proposed herein is not duplicated by any of the other 139 Regional Research Projects listed at http://www.msstate.edu/org/saaesd/cris/reproj.htm; furthermore, NC-170 focuses primarily on protective clothing. It also is broader in scope and compliments some of the related projects listed under the USDA-ARS program area of Quality and Utilization of Agricultural Products.
Justification: The textile industry today is concerned with five main interrelated issues: procurement of raw materials, converting them into finished products, meeting customer expectations, and human health/environmental safety, and making a profit. Many of these issues parallel the priorities developed by the ESCOP, e.g., the environment, sustainable production systems, economies of rural communities, and consumer interests. There also is a need to develop new materials and composites and new manufacturing technologies for making textiles that are more "environmentally friendly." Finding alternative raw materials, synthetic pathways, natural processes, and reaction conditions are central to EPAs mission and have been endorsed by former president Clinton since 1995.
Justification for Developing Value Added Products from Renewable/Recyclable Resources
The U.S. agriculture accounts for -
20% of the GNP through the production of agricultural raw materials and products. The agriculture industry also contributes $40 billion annually in exports with each billion resulting in -
30,000 jobs. However, oil imports continue to drain our economy and account for -
20% of our trade deficit. At the same time, the world's plant biomass is about 2,000 billion tons, and the renewable resources amount to about 100 billion tons/year (68). Given the abundance of biomass feedstocks, the value added to the U.S. economy, and potential reduction in the trade deficit, it seems logical to pursue the use of biomass for production of materials and chemicals. Furthermore, considering the four million tons of post-consumer textiles waste generated annually, the rising cost of disposal, reduction in available space, and increasing environmental concerns, research is critically needed on innovative recycling technologies for textile wastes.
Since the introduction of nylon in the1940s, synthetic fibers have had a significant impact on the quality of our lives. However, the demand for natural fibers continues to increase because of their many outstanding properties, including aesthetics, comfort, and biodegradability. Research efforts are refocusing on exploring alternative fiber crops, crop residues, and agricultural by-products, which are often underutilized. For example, kenaf fibers are environmentally friendly, easy to grow, and adaptable to many soil types. AES scientists in AR, GA, LSU-LA, and TN (3, 13, 14, 28, 80, 90-92) are investigating kenaf because of its economic potential for fiber producers as well as the cottage craft industries in AR and other states with significant craft industry-based economies. However, before kenaf can become a viable crop, new value-added products must be developed to prove its versatility in the textile, apparel, and craft industries. Work by scientists in AR, KS, and MS (18-20, 82-83) has resulted in the production and manufacturing of a variety of kenaf products for both apparel and home furnishing uses. Because of the worldwide interest in kenaf, hemp, jute, and other alternative crops, work is needed to further enhance its aesthetics and properties for a wider range of products through bleaching, dyeing, and finishing. These research efforts will facilitate the use of kenaf in various types of value added products for the craft industry.
Similarly, agricultural residues and by products from major U.S. agricultural commodities (sugarcane, soybeans, wheat, corn, etc.) could be used to produce a multitude of value-added non-food products, ranging from fibers, films, plastics, and composites to resins, finishing agents, and auxiliaries. Furthermore, many of the chemical compounds used for textile wet processing are currently obtained from petrochemicals and could also be derived from agri-chemicals.
Sugarcane is an important agricultural crop in LA and FL. FL producing is the largest U.S. producer of sugarcane with a crop value of $472 billion (53.2% of the total), followed by LA with a crop value of $287 billion (30.4%). Most of the crushed stalks (bagasse) currently produced are used as in-house fuels in mill processing and for other low value applications, such as mulch and ceiling tiles (75). Development of value-added products from the waste or low-value materials could allow mills to migrate to cleaner burning fuels and provide economic benefits to sugarcane producers. Researchers in LSU-LA have developed processes for converting bagasse into textile and geotextile products (19-20). The development of higher value-added products would create new uses and provide economic benefits for both LA and FL.
About 22% of the textile fibers produced are used for industrial applications, which includes low and high resin composites (113). However, the abundance of recyclable fiber and agricultural residues, coupled with the dwindling supply of some natural resources, have created the need to develop alternative structural composites. Researchers in KS, NE, and LSU-LA (30, 34, 39) have been exploring the use of agricultural residues and byproducts from wheat, soybeans, and bagasse for textile applications and low and high resin composites. Agri-plastics and composites appear to be the most promising use for many of these crop residues. However, many challenges exist in identifying market segments, determining performance requirements, selecting resins, and optimizing manufacturing properties. Attempts to manufacture agri-fiber composites have been impeded by finding suitable resins that parallel the quality of wood-based particleboards made with formaldehyde-based resins. Fundamental research is needed on evaluating the flow properties and manufacturing conditions to maximize the use of agri-plastics in toys and other consumer applications.
Work also is being done on making solvent- spun cellulosics from cheaper raw materials, such as agricultural wastes (e.g., bagasse and wheat straw) or post consumer cellulosic textiles (rayon and cotton and their blends). Obtaining fiber-forming cellulose solutions (lyocell) from reclaimed cellulosics or agricultural wastes has become important topic for researchers in LSU-LA, KS, and TN (15, 21, 22, 69). Likewise, researchers in TN in conjunction with Cargill first produced spun-bonded
and melt-blown nonwovens with good strength, cover, and hand properties from polylactide derived from the by-products of the fermentation of corn. Further work is needed on exploring other nonfood uses of agricultural residues.
Justification for Bio-Processing and Related New Technologies for Textile Applications
Developing environmentally safer methods for processing textiles can be done with biological systems, rather than conventional chemistries. Historically, enzymes have been used for the retting of bast fibers and desizing of grey goods. In the last decade, enzyme systems have been developed for bioscouring and antipilling cellulosics and for imparting anti-felting properties to wool (9). New frontiers in biotechnology for textile processing include cross-linking of polymer chains to impart easy-care properties, and enzyme surface modification to enhance absorption/dyeing and aesthetic properties. Another approach to reducing the use of energy, chemicals, and time in textile processing is dual or multi-purpose dyes and finishing agents. KS is developing a dual purpose, dye-insect control agent produced by linking together a reactive dye with insect growth regulators. This technology would help reduce the $1 billion annual loss due to insect attack.
Rapidly progressing, new technologies require innovative materials for a large variety of applications. Optimizing both the bulk and surface properties of various materials represents one of the promising approaches for meeting the technical and economical requirements for high-tech materials. Because of the cost related to developing new fibers, polymer chemists now focus on modifying existing fibers to impart the desired aesthetic or functional properties. Conventional fiber modification methods include various thermal, mechanical, and chemical treatments. Another important means of modifying the fiber to increase the uptake of dyes and finishes or to impart unique functionality is through plasma technology (102-104). The reactive species of plasma, resulting from ionization, fragmentation, and excitation processes, are high enough to dissociate a wide variety of chemical bonds, resulting in a significant number of simultaneous recombination mechanisms. Plasma syntheses opens up new possibilities for polymer chemistry, particularly in industrial applications where the specific advantages of producing pore-free, uniform thin films of superior physical, chemical, electrical and mechanical properties have been required.
The main advantages of plasma polymerization methods are: 1) applicability to almost all organic, organo-metallic and hetero-atomic organic compounds, 2) modification of surface properties without altering the bulk characteristics, 3) low quantities needed of monomeric compounds making it non energy intensive, and 4) wide applicability to most organic and inorganic structures. Exciting applications in polymer chemistry include 1) cold plasma discharge synthesis of new polymeric structures, 2) plasma induced polymerization processes, 3) surface grafting of polymers, and 4) surface modification of polymers. Characteristics that can be improved include wettability, flame resistance, adhesive bonding, printability, electromagnetic radiation reflection, surface hardness etc.
Justification for the Development of Textiles for Protective/Medical Applications
Specialty textiles contribute to human health, safety, and comfort by protecting people from exposure to heat, chemicals, pesticides, pathogens in blood and body fluids, and electromagnetic radiation. AES researchers continue to focus on developing textile systems for human health and safety. Two important requirements for protective medical apparel are barrier effectiveness and comfort for the wearer. Depending on the end-use, different characteristics are required to achieve the optimum barrier efficiency. The effectiveness of protective apparel worn by patients and health care workers continues to be a major concern because of the risks of infection when the protective apparel fails. The risks are especially great with the spread of HIV and other infectious diseases. OSHA estimates that more than 5.6 million health care/public safety workers are at potential risk of being exposed to HIV and hepatitis B. Centers for Disease Control [CDC], the Association of Operating Room Nurses [AORN], and the Occupational Safety and Health Administration [OSHA]) have published guidelines for health care workers to minimize exposure risks. OSHA mandates that health care workers wear personal protective equipment that meets specified performance levels (25).
Another major health concern is the increasing incidence of skin cancer caused by exposure to sunlight. Skin cancer is the most common form of cancer in the U.S., resulting in -
9,000 deaths annually. Both acute and cumulative exposure to solar radiation, especially UV-B radiation (280-315 nm), can result in sunburn, aging, immune-suppression, and skin cancer. The risk of developing skin cancer can be greatly reduced by minimizing exposure or by using protective apparel. The challenge for textile scientists is to develop summertime fabrics that are both comfortable and provide adequate protection. Work is needed to elucidate the relationship between the chemical structure of dyes and their UV-absorbing properties, and how the UV-absorption of fabrics is influenced by end-use conditions (light, laundering, and wear). Researchers in KS, NE, TN, and TX (24, 109, and 123) are interested in the influence of polymer additives (delustrants, UV absorbers, and fluorescent whitening agents) on the UVR transmission of fabrics. Work is needed to answer the question of whether the type of water (distilled, sea, or pool water) influences UV transmission. A predictive model is needed that interrelates the influence of fiber, yarn, and fabric characteristics on UPF of fabrics. Doctors (KU Medical Center) have requested information on the relationship between fabric and apparel characteristics that will provide adequate UV protection. Such information would be beneficial to doctors when counseling patients on preventive measures for reducing the risk of skin cancer.
Justification for Developing and Evaluating Textiles with Enhanced Resistance
(or Susceptibility) to Environmental Degradation
Heat, light, atmospheric contaminants, weathering, microorganisms, and insects are some of the major environmental agents that can attack and degrade textiles during wear, use, or storage. For many end-use applications, resistance to these environmental agents is desirable. However, one or more of these degrading influences may be targeted when designing textiles that are environmentally degradable. S-272 researchers have examined many aspects of environmental degradation related to enhancing the resistance or susceptibility, depending on the product end-use and requirements.
Related, Current and Previous Work
Our former Regional Project S-272, Development of Textile Materials for Environmental Compatibility and Human Health and Safety (terminates 9/30/2001) resulted in numerous publications cited herein and at our web site (http://msa.ars.usda.gov/la/srrc/csrees/main.htm). The proposed research focuses on developing value-added products from agricultural fibers, residues, and by-products, especially those having multi-state importance to the participating states. Greater emphasis will be placed on developing a) textile products from renewable and recyclable resources and b) textile processing methods utilizing enzymatic and plasma treatments. Work will continue on assessing the environmental compatibility of selected and newly developed materials and their suitability for specific end-uses, including consumer products and medical/protective textiles. Equipment, experimental methods, processing technologies, and expertise relative to the synthesis, extraction, and processing of agri-fibers, previously developed through S-272 project, will be utilized and expanded upon in this proposed multi-state-project as reflected in the current and previous work..
Development of Value Added Products from Renewable/Recyclable Resources
The diversity of resources for this objective result in a concentration of cooperative efforts under sub-objectives, embracing novel ideas for making products from renewable and recyclable materials.
New Lyocell and Composite Fibers and Fabrics Derived from Biomass. The earliest commercial method for manufacturing regenerated cellulosic fibers (rayon) from delignified wood chips is the viscose process, which is complex and environmentally-polluting. Today, solvent-spun cellulosic fibers (lyocell) are being produced from more environmentally benign solvents. A major limitation of these new lyocell fibers is fibrillation under wet abrasion conditions. The formation of lyocell fibers from N-methyl morpholine N-oxide monohydrate, NMMO.H2O, solutions in conditions allowing a tailored fibrillation is currently being investigated at KS, LA-LSU and TN (22). This is achieved by controlling rheological parameters in the liquid crystalline phase preceding fiber formation (77, 78) or by using an electrical discharge (cold plasma) radio frequency technique, developed by WI and LSU-LA. Work also has been done on spinning lyocell fibers from kenaf, steam exploded bagasse, and wheat straw (81, 92). Negulescu et al. (70) have proposed a scheme to separate the cellulosic component from PC blends from which lyocell fibers can be spun.
A new area of interest is biosynthetic polymers, such as polylactide polymers (PLA), produced by Dow-Cargill from corn-derived lactic acid. These polymers can be processed by melt fabrication methods (61). Morphological transformation of PLA fibers/fabrics during the application of disperse dyes have been recently presented by LSU-LA investigators (60).
Non-food Applications of Agricultural By-products and Residues for Textiles and Composites. Agriculture production generates residues in the form of cereal straw, corn stover, sugarcane bagasse, etc., while the production and consumption of soft goods generate billions of pounds of fiber and textile residues. Attention has been drawn to converting these agri-residues and consumer wastes to textiles, composites, paper pulp, and other consumer and industrial products. KS researchers have investigated the use of tocopherols to reduce photo-degradation in fibers and soy proteins as fillers in thin plastics films (39). More recently, Gatewood, Ramaswamy, et al. (35) have focused on adding value to agri-composites through bleaching, dyeing, and controlling particle size. An H2O2 bleaching system with an acetic acid rinse substantially improved the whiteness of the straw. Major limitations of straw board that need to be addressed are poor moisture resistance and adhesion with formaldehyde-based resins because of the waxy cuticle. Ramaswamy et al. (30) investigated the use of straw and kenaf as fillers in plastics or high resin composites. The agri-fibers and resins were ground into specified particle sizes, extruded, chopped into pellets, and hot pressed into thick plastic sheets, then exposed to heat, light and weathering. Work is needed on the processing and properties of other agri-fibers, by-products, and residues, or recyclable materials for composite applications.
Development of Degradable Cotton-core Nonwovens. Cotton, a renewable resource, has many properties that are most suitable for constructing absorbent nonwoven products. Preparation of nonwovens made of biomass PLA or polyesterimide at TN could make the cotton-core products completely biodegradable and further increase consumer appeal (47, 67). Cotton-core nonwovens will be elaborated in the following sections related to the use of biodegradable hygiene products.
Development of Kenaf Value-added Products for Textiles and Crafts. The Kenaf Demonstration Project was started in 1986 with cooperation from USDA and Kenaf International. Its primary goal was to make the kenaf industry a reality in the U.S. (New Multi-state Project Development Committee DC-95-06). Today, kenaf is a market-ready commodity used in making paper, cordage, fabric blends, etc. (80, 82, 83). Much work has been done on fiber preparation to facilitate the utilization of kenaf in value-added products. Research is needed to introduce kenaf to the craft industry for producing various types of value-added products - baskets, hats, etc. Hence, kenaf could have a formidable influence on craft industry in AR and other states.
Development of Industrial Textile Products from Sugarcane Fibers. Researchers at LSU-LA, FL, and TN (13, 14, 18-20) have focused on developing products from sugarcane for commercial use in geotextiles and other industrial applications. This integrated project, involving states producing almost 85% of the U.S. sugarcane, exemplifies the development of mill-to-market, bio-based, value-added products. Work has been done at LSU-LA on delignification, cellulose extraction, and fiber processing methods. Results indicated that atmospheric extraction can be used and steam explosion omitted with higher alkaline concentrations (91). A pilot scale reactor was constructed at LSU-LA to bring the process closer to commercial production conditions. Preliminary results have demonstrated that sugarcane rind, mechanically separated from the stalks, can be chemically and mechanically treated to extract the valuable cellulosic fibers.
Bagasse erosion control mats have been produced and tested against existing commercial products. These mats were comparable in performance to commercial wood, straw, and coconut products and had better water, light, and flame resistance than other commercial products (111, 112). The bagasse and coconut fiber mats also biodegraded at approximately the same rate. Significant advantages of the bagasse mats were 1) stitching was not needed because of fiber entanglement and 2) ability to conform to the contours of the soil, preventing washout. Preliminary work on bleaching and dyeing of sugarcane fiber have been carried out (90). The geotextile erosion control market is an area of expected growth. The development of other value-added products from bagasse will result in economic development at the local, national, and international levels.
Development of Bio-Processing and Related New Technologies for Textile Applications
The overall objective in this multi state research project is to utilize relatively new surface enhancing technologies to improve the processibility, functionality, and aesthetics of fabrics. Research activities in this objective address three important areas related to purifying and enhancing the characteristics and appearance of textiles: 1) bioprocessing, 2) plasma application, and 3) digital printing.
Recent advances in biotechnology have given the textile industry new auxiliaries (enzymes) for wet processing (9). Sarkar and Etters (94-97) have extensively studied the biopreparation of cotton and enzyme kinetics using alkaline pectinases. Other enzymes used in biopreparation of cotton are amylases for desizing and cellulases to enhance the surface characteristics (smoothness). Ramaswamy et al. are studying the use of enzymes for biofinishing kenaf and kenaf/cotton blends. Proprietary treatments have resulted in dramatic increases in the whiteness, appearance, and hand. Similarly, protein disulfide isomerases are commercially available for improving the shrinkage behavior of wool (103). Work by Buschle-Diller (8) at AL investigated the effectiveness of five proteases from plant and microbial sources on wool fabrics. KS is focusing on developing enzymatic methods for purifying/biofinishing of wool and specialty hair fibers using xylanases and pectinases. Gatewood and Ramaswamy (36) have shown that the wool subjected to chlorination and isomerases was less prone to insect attack. The surface properties of enzyme-treated fabrics have been studied by Ramkumar (85) using a simple sliding friction apparatus to objectively quantify the enhancement.
Research on modifying the surface characteristics of polymers/fibers using plasma technology has been the focus of research by Sarmadi et al. (26, 27, 31-33, 49, 50, 101-104) at WI s Plasma Research Institute. Plasma modification methods serve as a pivotal contribution to the research work at the other contributing institutions in this project. These studies have shown new demands for syntheses of special property plasma polymeric layers, through the incorporation of additional elements, such as various metals. Studies carried out at WI have demonstrated that low electron energy (20-30 eV) MS spectrometry of plasma monomers can offer valuable data in this field.
Development and Evaluation of Textile Systems for Protective and Medical Applications
A relatively new area of textile development has been the design, production, and evaluation of textile systems for protecting individuals from chemicals, heat, harmful electromagnetic radiation, and high-speed impact (air bags and bullet-proof vests). Recent studies by AU, NE, and KS have focused on quantifying the degree of UV protection afforded by textile materials and in identifying the various factors that affect it. These states have the expertise and instrumentation for determining UPF values for textiles. Many parameters influence a fabrics UV transmission. NE and KS (24, 109) have generated databases of UPF values for a range of fiber and fabric types. Recent work by Crews et al. has shown that fabric porosity is the best predictor of UV blocking properties, and polyester, silk, and wool fabrics exhibited better UV blocking properties than cotton or rayon. Zhou and Crews (123) demonstrated that detergents with optical brighteners improved the UV blocking ability of fabrics. Srinivasan and Gatewood (109) investigated the influence of 95 dyes on the UV protection provided by cotton and nylon fabrics and the relationship between chemical constitution and UV absorption. Most of the dyes caused a substantial reduction in the UV transmission, and the reduction was linear with concentration up to a point. The interpretation of the absorption spectra of the dyes with respect to their chemical structure indicated that in most dyes, UV absorption depended on specific structural attributes rather than the chemical class to which they belong. Work is needed on how environmental conditions (water and light exposure) and other additives influence UV transmission.
Previous studies by WI (88, 89, 98-100), TN, GA (51-58) and KS (64) have addressed the barrier properties of textile substrates for human health and safety. Fabric penetration and worker contamination depend on the fiber chemistry, yarn/fabric construction, surface treatments, and environmental conditions. Growing concern about HIV and other infectious diseases attributed to blood borne pathogens has resulted in the development and testing of the barrier properties of textiles to fluids and microorganisms. Many medical textiles do not have adequate barrier properties to effectively protect medical personnel from pathogens. Sarmadi et al. (88, 89) and Leonas and Huang (52, 55, 58) have shown that fabrics treated with low surface tension liquids (fluorochemicals) have enhanced barrier properties. They (7, 53, 54-57) also have identified parameters critical to barrier properties, e.g., fabric surface chemistry, fabric geometry, challenge liquid, and organism properties. The complex nature of fabrics resulted in many challenges for investigators. Advances in microscopy technology have provided insight into transmission mechanisms. Researchers at GA evaluated the mechanism of small particle transmission necessary to develop new fabrics with suitable barrier properties using Laser Scanning Confocal Microscopy. Work continues on developing moisture permeable fabrics that provide barriers to liquids and microorganisms. Other studies by Cloud et al. (11, 12) have focused on the comfort of protective apparel by objective and subjective methods. Correlations were found between objective and subjective data, comfort and barrier efficiency, and specific fabric characteristics. As new materials are developed, comfort assessment is critical.
Development of Textiles with Enhanced Resistance
(or Susceptibility) to Environmental Degradation
The term "sustainability" has been adopted to convey the realization that our environment is more favorably served by conserving resources, be it by improving the durability of textiles, making materials more biodegradable, or recycling of plastics or fabrics. For example, reclaiming the cellulosic component from a polyester-cotton blend would provide an excellent source for the production of the more environmentally friendly fiber, lyocell. Negulescu et al. (69) proposed a process for separating the cellulosic component from blends from which lyocell fiber can be spun.
Researchers at KS and WI are focusing on the biological resistance of wool. A recent study (36) has shown that most wool and specialty hair fibers are readily attacked by webbing clothes moth and black carpet beetle larvae, but the extent of degradation is influenced somewhat by animal type, fiber fineness, and chemical treatments. With the elimination of dieldrin and the potential ban on other insecticides (59), research is needed on alternative methods of insect control. Current work at KS and WI is evaluating multifunctional reactive dyes and plasma treatments for imparting insect control.
New biosynthetic polymers, such as PLA polymers and some copolyesters are easily synthesized from renewable resources via fermentation from carbohydrate-rich material, such as food industry by-products (dairy wastes and potato peels). PLA polymers are bio-/hydro-degradable and offer a broad balance of functional performance, making them suitable for many applications. They are expected to compete with hydrocarbon-based thermoplastics on a cost/performance basis.
Cotton has many outstanding properties suitable for constructing effective capillary control in absorbent nonwovens. Internal microscopic pore spaces of cotton contribute to its ability to swell during the transition from the dry to the wet state. The excellent absorbency and water retention properties of cotton-core laminates with melt-blown polypropylene webs have been demonstrated (65, 117), but these nonwovens are not biodegradable. Nonwovens made of PLA (47) or polyesterimide (67) at TN could further expand the use of cotton in the large absorbent cores market and make the products completely biodegradable to further increase consumer appeal.