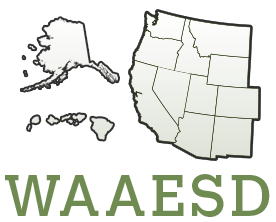
W186: Genetic Variability in the Cyst and Root-Knot Nematodes
(Multistate Research Project)
Status: Inactive/Terminating
W186: Genetic Variability in the Cyst and Root-Knot Nematodes
Duration: 10/01/1998 to 09/30/2003
Administrative Advisor(s):
NIFA Reps:
Non-Technical Summary
Statement of Issues and Justification
STATEMENT OF THE PROBLEM
The cyst and root-knot species are the most important groups of plant-parasitic nematodes in the United States. The management of these nematodes in United States agriculture during the past
four decades has largely been via, the application of broadly efficacious nematicides. Nematicidal activity, especially of soil fumigants, is generally nondiscriminating, even between nematode
species and genera. Therefore, understanding the genetic variability among nematodes was not important; in effect, ""the only good nematode was a dead nematode."" In contrast, desirable
alternative nematode management involves combinations of rotation, host plant resistance, cultural manipulations and biological control, all of which may have specific genotype-level interactions with nematodes. Hence, the genetic variability existing in target nematode
populations must be considered to successfully develop and deploy alternative nematode management strategies. This regional project was initiated because the membership recognized the increasing importance of characterizing the genetic variation in nematode populations and
understanding how that variation influences the success of alternative nematode management strategies. An example highlights the value of this project: Years of research went into the development of cyst-nematode resistant soybeans but the potential benefits of the resistance were limited due to the rapid selection of resistance-breaking nematode isolates.
During the.previous life of this project, the membership effectively initiated research to apply fledgling methodologies to obtain knowledge of the genetic variability in nematode populations.
The W-186 membership proposes to continue and extend our efforts in this regard, to identify and characterize the genetic variability in the most important cyst and root-knot nematode groups. The participants share research interests on primary nematode pathogens and bring complimentary, but not overlapping, expertise to the project. In this iteration of the project, we will address gene frequencies, genetic stability, and adaptation and fitness, such that genetic variability can be managed and manipulated in agricultural production systems by appropriate alternative management strategies. President Clinton has pledged to implement integrated pest
management, and the objectives of this project are a significant step toward such implementation.
The need for integrated nematode management has also been significantly enhanced by the Montreal Protocol of 1995 and the Food Quality Protection Act (FQPA) of 1996. Because of the inherent scientific uncertainties involved in' risk assessment, and the FQPA requirements to consider cumulative, aggregate exposures, and endocrine effects, it is likely that several widely-used and efficacious nematicides will be unavailable in the future. For example, a widely used nematicide, 1,3-dichloropropene (Telone 1I), is a B2 carcinogen, and will be subject to review under FQPA. In addition, methyl bromide will be unavailable in the near future due to the U.S.
Environmental Protection Agency phase-out of production and importation of methyl bromide starting January 1, 2001.
New molecular and genetic methodologies and knowledge will facilitate the study of nematode genetic variability at much greater resolution than has been possible. Development of interactive database programs that provide information and advisory information on combinations of
nematode management tactics will facilitate the adoption of integrated nematode management.
Such on-line databases and knowledge-based systems will assist in information transfer to user groups in the relevant agricultural communities.
JUSTIFICATION
Plant-parasitic nematodes cause an estimated 10-14% average annual yield loss among the world's major crops (Sasser and Freckman, 1987). Losses in United States' major crops due to plant-parasitic nematodes are estimated to range from minimal in some localities to as high as
15% in other areas (McSorley et al., 1987). Increasingly, scientific evidence and public awareness have heightened concerns about environment quality, food quality, and human health and safety relative to pest management in agricultural production (Pesek et al., 1989). Since the late 1940s, nematicides have been the primary tactic used to manage nematode pathogens in agriculture. As with other pesticide-based management programs, reliance on nematicides has
limited the planning horizon in many cropping systems to an annual, preplant basis. The primary objective of management tactics has been to reduce densities of nematodes and other pests.
These strategies force the system to behave as required by the manager, with associated financial, environmental and long-term costs. For years, the soil has almost been considered as an efficient
and reliable sink, wherein residual nematicides are readily degraded to benign components.
However, there are frequent examples of groundwater pollution with pesticides including several widely-used nematicides (Thomason, 1987). As an example, some 1,800 wells in Fresno County, California are contaminated with the nematicide 1,2-dibromo-3-chloropropane (DBCP), and problems with nematicides in groundwater are documented in Hawaii, Florida, Wisconsin, Rhode Island, and elsewhere. Other nematicides such as 1,3-dichloropropene (1,3-D) and methyl bromide are being studied as air pollutants, and in the latter case as a significant ozone depleter.
The public is demanding that the use of pesticides be decreased. The Food Quality Protection Act (FQPA) amendments to the Food Drug and Cosmetic Act mandate that the EPA consider multi-faceted risk assessment in setting tolerances for pesticides, and that currently available nematicides (for example) undergo reregistration. The end result? An urgent need for alternative nematode management.
A dramatic shift in nematode-management strategies is beginning to occur. A change from almost exclusive reliance on soil-applied nematicides, to the use of combinations of alternative strategies such as crop rotation, host plant resistance, cultural manipulations and biological control (Ferris et al., 1992; Pesek et al., 1989). An important difference between these alternative management approaches and nematicides is that the alternatives are influenced directly by genetic variability existing in target nematode field populations. Hence, the successful use of alternatives requires more information to implement than nematicide-based strategies (Caswell and Roberts, 1987; Noe, 1992; Riggs et al., 1981; Riggs and Wrather, 1992; Roberts and Thomason, 1989; Starr, 1989; Stirling, 1991). Herein lies the logic and raison d'etre of W-186: assessment and characterization of genetic variability extant in nematode populations will assist in the successful application of alternative management strategies, in addition to guiding the initial development and deployment of new strategies. As an obvious example, knowing the frequency of virulence genes in a nematode population will allow deployment of
corresponding resistance genes so that the utility of the resistance genes in new cultivars is maintained over time.
Except in the clearly demonstrable instances where resistance-breaking nematode populations are detected, the subtle influence of genetic variability in nematode populations has been considered
only to a limited extent. However, there is considerable evidence that this variability is important. We hypothesize that genetic variability in nematode populations is responsible for the
aberrant results of many experiments assessing resistance, crop rotations, host ranges, cover/trap cropping, and biological control. The plasticity of nematode responses to abiotic environmental
factors such as temperature, moisture, and host nutrient status stems from genetic variability, and such responses are poorly characterized. Greater understanding of nematode genetic response to
abiotic factors will be important in optimizing the design of cultural management tactics such as manipulations of planting and harvest times, wet or dry fallow, and solarization.
The root-knot and cyst nematodes are distributed throughout the United States and are damaging pathogens, parasitizing a wide range of important crops. Three groups of nematodes have been selected as the primary focus for this project: Group I - The warm-temperature root-knot species (Meloidogyne incognita, M. javanica, M. arenaria); Group II - The temperate root-knot species (M. chitwoodi and M. hapla); Group III - The cyst species (Heterodera schachtii, H. cruciferae and H. glycines). These nematodes are the subject of research efforts in the designated participating states. Current research is addressing many areas of management for these three
groups, including: development and deployment of nematode-resistant plants; rotation to reduce population densities of these pathogens; cover crops and trap crops to reduce population densities; characterization of resistance genes and resistance responses; and the development of biochemical and molecular diagnostics for nematode identification. Thus the project participants share a strong common interest that will provide the central focus for the project members and other collaborators.
A. Characterizing genetic variability - a critical need:
The unifying theme of this proposal is that genetic variability is a critical biological feature of species and populations of the highly specialized cyst and root-knot nematode parasites of plants.
It is within the past ten years that nematologists have begun to document the extent of genetic variability within populations, and the plasticity of plant-parasitic nematode genomes relative to
selection pressures. The information gained has arisen primarily because of continuing new developments in the techniques of molecular biology, and this project will continue to increase our understanding of the phenomenon involved. As increased insight has been gained into
genetic variability in nematode populations, it has become clear that the ""race"" concept does not provide a particularly effectual characterization of nematode populations vis a vis management.
This is particularly true because the importance of mutation compared to maintained variability in field populations is unclear, and this is a research area that will be pursued.
Genetic variability can impact both the effectiveness and longevity of alternative nematode-management strategies based on host plant resistance, crop rotation, cultural manipulations and biological control. As a consequence, greater understanding of genetic variability should provide rational guidance for the design and development of management strategies. The W-186 project
focuses on understanding nematode genetic variability, such that it can be identified, characterized, and managed or manipulated to benefit agricultural production systems. The underlying principals to this focus require research on the phenotypic and genotypic
characterization of variability and gene frequencies, including aspects of stability and adaptability, of host range, response to resistance, response to environmental conditions, biological processes (e.g. fecundity) and morphology. This approach will be complemented and aided by development of markers to identify variability by molecular, biochemical, histochemical, and morphological polymorphisms. Within the context of this project, some
contributors will also investigate, as appropriate, genetic variability within several other important nematode genera (see Table 1, pg. 8).
Our first project period as W-186 has allowed participants to make inroads in some of these 'research areas. However, as this is a new research area, much remains to be accomplished. Accordingly, the research we initiated under W-186 can hardly be considered ""complete.""
Relative to our objectives, it is exciting that the arsenal of new tools used to address our applied research questions is increasing rapidly (e.g., Avise, 1994; Grosberg et al., 1996; Karl and Avise,
1993; Lynch, 1996; Vos et al., 1995; Williams et al., 1990; Williams et al., 1991).
Within the context of this project, there are key considerations central to development and deployment of alternative management strategies that are influenced by genetic variability in cyst
and root-knot species. Some of the component considerations are highlighted as follows:
Host plant resistance - The genetic composition of nematode populations are changed by the selection pressure imposed by growing resistant cultivars. The changes include shifts in species composition, and shifts in presence and frequency of nematode virulence alleles matching specific resistance genes in crop cultivars. Similar potential shifts may occur in response to nematode-resistant trap crops. Little is known of the existing frequency of
virulence alleles, the frequency with which new alleles are generated, or the underlying mechanisms that regulate changes in genetic variability in root-knot and cyst nematode populations. As more sources of resistance are bred into cultivars, knowledge of gene
frequency and stability effects assumes greater importance in determining the direction and requirements of breeding for nematode resistance, and the effective long-term deployment of
available resistant cultivars. With the.exception of H. glycines responses to soybean genotypes (Dong et al., 1997; Dong et al., 1998; Dong and Opperman, 1997; Riggs and Schmitt, 1988), race or biotyping schemes to categorize virulence/avirulence genotypes for
the cyst and root-knot species are nonexistent or inadequate. Sugarbeets with resistance to H. schachtii have been subject to preliminary tests in Europe, and isolates of H. schachtii
capable of overcoming the resistance reveals the presence of virulent races or biotypes (Muller, 1992). The recent successful cloning of nematode resistance (Cai et al., 1997) from Beta procumbens will allow evaluation of sugarbeets carrying resistance, and hence accurate determination of the frequency of resistance-breaking alleles in field populations of sugarbeet cyst nematode. Genetic variation in North American H. schachtii populations has been
documented, but not specifically in relation to the cloned resistance allele. In root-knot species some race differentiation within certain species e.g. M. incognita, M. arenaria, M. chitwoodi, has been made using diverse plant host differentials (Sasser and Carter, 1985).
Field isolates of root-knot nematodes in California that are capable of breaking nematode resistance (Mi-based resistance) in tomato have now been documented (Kaloshian et al., 1996). Currently no differentiation scheme exists for biotyping population responses to
resistance genes within a crop plant; such genotypic differences are known to occur but their extent, distribution relative to cropping systems, and stability are poorly understood. In this regard, the implementation of an improved biotyping scheme via activities of W-186 is highly desirable (Roberts, 1995).
Host range for rotations and cover-cropping - The host ranges of important cyst and root-knot nematode species have been defined within general limits, but the extent of variability in
host range among populations within species is not well-characterized. Thus, although we know that most cyst nematodes have narrow host ranges and are amenable to control by nonhost rotation programs, little is known about the extent of apparently aberrant reports of hosts outside the typical host taxa, or the likelihood or rapidity of shifts in host range. For example, although H. schachtii hosts are found almost entirely within the Brassicaceae and
Chenopodiaceae, tomato (Solanaceae) has been reported to host H. schachtii in California (Lear and Miyagawa, 1972) and Utah (Griffin, 1982a). This has serious consequences for rotation planning in western sugarbeet production areas. Weed hosts of H. schachtii were
found to be better hosts for nematode populations collected from the same weed host populations in the same locality than for nematode populations collected from separate localities, suggesting genetic adaptations had occurred for modification of host range
(Griffin, 1982b). The geographic origin of H. schachtii populations appears to influence the host suitability of weeds, as California isolates of weeds and H. schachtii interacted differently than was observed in Utah (Bloom, 1997). Hosts of differing host capacity have
been shown to act as a selection pressure on populations of H. schachtii, and different geographic isolates of the nematode responded differentially to the selection pressure in as
little as four generations (Kaplan, 1997; Kaplan et al., 1998).
In contrast to cyst nematodes, root-knot nematode species have characteristically broad host ranges that include more than 2000 plant species from diverse plant families (Roberts and
Thomason, 1989). Much of this host range information has been compiled from numerous tests and observations based on non-standardized host testing procedures, and in most cases
with only one or a few isolates of a root-knot species. While considerable genetic variation for host range can be expected in any of the warm- and cool-temperature root-knot species, comparisons under uniform, standardized conditions are needed to determine whether differences are due to variability in nematode populations or to differences in susceptibility in the plant germplasm lines or accessions used. Resolving the true levels and stability of host
range relationships will be critical to development and implementation of nonhost crops in rotation and cover-cropping programs, and for determining the role that host weed species
play in maintaining nematode population levels during rotation and cover-cropping.
Cultural controls - Most are based on some form of manipulating abiotic effects on nematode populations so that population levels are reduced or nematode activity and
infection are avoided. Examples of reducing populations include the application of wet or
dry fallow so nematodes starve while active (wet fallow) or exposed to extreme conditions of
moisture stress (dry fallow). Similarly, soil solarization involves natural heating of soil under
plastic cover such that the thermal death point of nematodes is reached under set conditions
of moisture and high temperature. Avoidance may include changes in planting and harvest
dates, such as delaying vegetable planting in the fall to avoid infection activity of M.
incognita, which is minimal at soil temperatures below 18C (Roberts, 1987). Other
alternatives include early planting of sugarbeets in H. schachtii-infested fields or late harvest
of potatoes in M. chitwoodi-infested fields to avoid additional nematode generations
developing in late season or on overwintering plants (Roberts and Thomason, 1981;
Pinkerton et al., 1986). The genetic variability in nematodes for response and adaptation to
different imposed conditions of temperature and moisture has been demonstrated (e.g.,
Hominick, 1979), but how quickly, how widespread, and how stable such adaptive changes
are, and the frequency of such adaptive genotypes in populations are not known.
Biological controls - Some potential biological control agents of cyst and root-knot
nematodes are known to have quite specific host ranges within and between their target
nematode species, such as the host specificity of the bacterium Pasteuria penetrans among
Meloidogyne species and populations (Davies et al., 1992; Stirling, 1991). Such specificity
may be controlled genetically, through surface protein binding and recognition between
bacterium and nematode (Davies et al., 1992), suggesting that genetic variability in
Meloidogyne may influence the potential of the bacterium and similar organisms as useful
biological control agents.
B. The Need for, and Advantages of, Cooperative Research:
The cyst and root-knot species are of primary importance as major nematode pathogens in most
agricultural production areas and cropping systems in all of the United States. This is reflected in
the proposed contributions from participating states across the country. The diversity in cropping
systems and rank of importance of nematode groups among participating states clearly provides
opportunities for conducting meaningful collaborative research on major nematode pathogens
exposed to similarities and variations in crop (host), resistance, environmental and
agroecological conditions. Via this project, the participants utilize the opportunity to collaborate
in ways that enhance the benefits accrued from the research, as opposed to what might be gleaned
if the researchers were to simply pursue individual projects within limited geographic
boundaries. For example, the warm climate root-knot species will be studied by participants
from nine of the 12 participating institutions encompassing 11 states (see Table 1) - a group
effort that will pay large dividends in understanding nematode population genetics relative to
management.
We believe that the similarities in the target nematode groups and the problems for nematode
management imposed by genetic variability can be researched most efficiently through this
coordinated regional project. This team approach will enable a pooling of scientific expertise
and resources to maximize the amount and quality of the information that can be generated. The
resources available to researchers working within the Agricultural Experiment Stations have been
continually declining in recent years, and are particularly limited for nematology programs at this
time. Conversely, the demands and expectations for new, environmentally friendly management
tactics have never been greater. Accordingly, the membership has experienced a ""do more with
less"" environment. This regional project can provide some relief, as a necessary forum for rapid
scientific advancement in aspects of both basic and applied research directed toward
development of alternative management strategies. For example, it is unlikely that all
participating states will have programs devoted to molecular research on nematodes, and yet the
need for molecular-level approaches to assess genetic variability is required to address significant
problems. This project has ongoing molecular-based programs in a few states (e.g. Arkansas,
California, Nebraska, North Carolina) that can act to facilitate research by other participant
states. In turn, those states focusing on phenotypic differences in nematode populations can
provide nematode populations and isolates for the molecular scientists. This coordinated
approach minimizes unnecessary duplication of research programs, and provides fertile
opportunities for a seamless, interactive approach to development of integrated nematode
management. Most importantly, the project also enhances the quality and applicability of the
research findings across a range of geographic locations.
Where possible and appropriate, participants will conduct research and will share information on
common and closely-related nematode species and crop systems. Participants will use similar
research approaches to the underlying questions of genetic variability, stability and adaptability.
Table 1 provides the matrix of common and collaborative research and information transfer
efforts proposed in this project relative to participant states, nematode groups, scientific and pest
management approaches, and crops. Within this framework we have regional commonalties, in
addition to those that are project-wide in scope. For example, Heterodera schachtii is a
significant problem in sugarbeet production in California, Idaho, Michigan, Nebraska, and
Wyoming; H. schachtii is important in cole crop production in California and Hawaii; H..
glycines is a primary pathogen in Arkansas, Michigan, Nebraska, and North Carolina; M.
chitwoodi and M. hapla wreak havoc in small grain-potato-alfalfa cropping in Oregon, Idaho,
Washington, and Northern California; and, M. incognita is often devastating in cotton and
vegetable cropping systems in Arkansas, California, New Mexico, and North Carolina. The
information generated by this project will be essential in formulating local, regional, and national
nematode management programs. Opportunity for exchange of nematode isolates, plant-
germplasm, and genetic marker sets (e.g. DNA probes) for comparative study will facilitate
strong collaborative linkage among participants. This has been formalized effectively through
this regional project. The molecular-oriented participant programs at California-Davis,
California-Riverside, Nebraska, and North Carolina will provide unifying research scope for
investigators from other states. Likewise, the emergence of classification schemes, such as root-
knot nematode biotyping at California-Riverside and host-range computer database and
knowledge-based advisory system to aid in nematode management decision making at
California-Davis, will provide unifying approaches for information transfer efforts among all
participating states.
The W-186 project is poised to make substantial contributions to the development and use of a
new biotyping scheme for use in describing intraspecific variation within the root-knot
nematodes (Roberts, 1995). The scheme provides an alternative to the current ""race""
designations, and will allow a more defined and flexible approach to the problem of genetic
variability in the root-knot nematodes.
Integral to the submission of this proposal are the extensive activities during the highly
successful first 5-year period of W-186. Project W-186 originated among members of WRCC-
78 (Griffin and Roberts, 1994), who shared the potential for collaborative involvement with
some 30 additional peer scientists in North America. Following the WRCC-78 annual meeting
in October, 1992, those investigators who shared significant common scientific interest in genetic
variability of cyst and root-knot nematodes formed the current group of listed participants,
resulting in W-186. The previous iteration of W-186 has resulted in a truly collaborative,
convivial regional research project. All meetings were well attended, with recent meetings
including St. Louis, Ft. Collins, Honolulu, and Riverside (this year) acting as hosts for the group.
This revision encompasses the nucleus of existing scientific expertise and experience for making
significant advances in this subject area. The application of the project findings should expedite
the development of new management strategies to minimize economic losses from nematodes.
This in turn should help boost the international competitiveness of our agricultural production
systems, at a time when competitive advantage is being eroded by the loss, and potential further
loss, of nematicides.
Related, Current and Previous Work
The general population level characteristics of roo?Sknot and cyst nematodes have received considerable examination. The requirements of accurate estimation of population densities as required for predictive management have received considerable attention (Barker, 1989; Ferris and Noling, 1987; Griffin and Roberts, 1994). A main research focus of western region and other nematologists has been in this subject area, including the research organized under a previous regional project, ?S134 (Griffin and Roberts, 1994). However, the genetic structure of nematode populations is the least understood and arguably the most important aspect of nematode population biology (Caswell and Roberts, 1987; Hyman and Whipple, 1996; Nadler, 1996).
Leonard and Czochor (1980) noted that any comprehensive consideration of the population biology of infectious disease must account for the shor?S and lon?Sterm changes in the genetic composition of the parasite and host populations. The opportunity for assessment of nematode genotypes is considerable given the continuing explosive development of molecularbased analytical techniques (Avise, 1994; Grosberg et al., 1996; Karl and Avise, 1993; Lynch, 1996; Vos et al., 1995; Williams et al., 1990; Williams et al., 1991).
Phenotypic variation: Host ranges of the major root?knot nematode species have been compiled from numerous reports worldwide (Sasser and Carter, 1985). The host ranges of M. arenaria, M. javanica, M. incognita, and M. hapla are extensive, including more than 2000 hosts from diverse plant families (Roberts and Thomason, 1989; Sasser and Carter, 1985). Although we know the general host range for root?knot species, the host range variability among populations of a species has not been analyzed in detail. Most host tests have been conducted with a single isolate of each species, with the goal of comparing species differences in host range. Thus, host?range phenotypes at the population level are unclear, and can only be determined under standardized test procedures in which a range of population isolates are compared.
Currently, the only scheme that allows discrimination of intraspecific variants is the differential host test devised by Sasser (Hartman and Sasser, 1985). This was designed primarily to aid in the species differentiation of M. arenaria, M. hapla, M. incognita, and M. javanica. The six differential hosts are designated cultivars of cotton, peanut, pepper, tobacco, tomato, and watermelon distinguish host?specific ""races"" within M. incognita and M. arenaria. The four putative lost races of M. incognita differ in ability to parasitize tobacco (cv. NC95 possessing a single dominant resistance gene to M. incognita) and cotton (cv. Deltapine 61 with no or unknown resistance factors). Two races of M. arenaria are separated by parasitic ability on peanut (cv. Florunner) (Hartman and Sasser, 1985). Unfortunately, and this is where problems arise, the races detected by the host?range differential do not correlate with other morphological, biochemical, or additional (a)virulence and host range factors or markers. For example, there are numerous reports in the literature that Coffea arabica is parasitized by M. javanica and by races 1 and 3 of M. incognita, although some California isolates of these species and races as defined by the host?range differential did not parasitize coffee (Araya and Caswell?Chen, 1996; Vaast and Caswell?Chen, unpbl. data). Accordingly, the management utility of the race designations for M. incognita is restricted to cotton and tobacco production areas. In fact, the application of the race designations in other geographic areas has led to some confusion in the literature, as races were (initially) essentially treated as uniform biological entities. As the designations were applied in different areas of the world, it was observed that biological variability existed within the races.
Lack of correlation of M. incognita races with (a)virulence factors matching a wide range of host?plant resistance genes can be illustrated by tomato and cowpea resistance gene systems. Both virulent and avirulent isolates for tomato gene Mi occur within M. incognita race 1, and both virulent and avirulent isolates for cowpea gene Rk occur within each of M. incognita races 1 and 3 (Roberts et al., 1995). Where additional resistance genes in the host have been identified, e.g. MO in tomato and Rk2 in cowpea, further differentiation of these Meloidogyne isolates can be made. Thus an isolate can have differential (a)virulence interaction with two genes of the same host species. Similarly, a resistance gene can have a differential (in)compatibility with two isolates of not only the same Meloidogyne species but the same traditional host race. This is no doubt the common pattern to be expected for distribution of (a)virulence factors matching a broad range of resistance genes. Thus it is unlikely that resistance gene homologies and hence virulence gene homologies are that close at the host/parasite recognition level across the spectra of Meloidogyne?host interactions, even if underlying mechanisms of interaction may be similar. Interactions of a nematode isolate with resistance genes from different plant taxa can be expected to vary widely. For example, Roberts (1995) has shown that routine use of certain root?knot nematode isolates in our resistance program that M. incognita isolates virulent on cowpea gene Rk are avirulent on tomato genes Mi and MO, and vice versa (Roberts, 1995). Resistance in Lima bean is effective against isolates virulent on cowpea gene Rk. Gene Mel in common bean is effective against M. javanica isolates that are virulent on cowpea gene Rk, and so on.
A possible explanation for these patterns is that each Meloidogyne species isolate (or field population) has an extensive series or bank of (a)virulence genes and alleles. These match the array of resistance genes found within an extensive host range of diverse plant taxa. Presumably each nematode isolate has a unique combination of the genes and alleles determining (a)virulence. The particular (a)virulence spectrum present in an individual will be the result of inheritance and the mutation and selection forces acting upon the initial or ancestral complement of genes and alleles, and changes in ploidy level. In the polyploid Meloidogyne species (a)virulence expression ma be modified by gene action on homologous chromosomes.
Because of the problems associated with race designations in root?knot nematodes, an alternative designation system has been proposed (Roberts, 1995) for characterizing Meloidogyne spp. (A)virulence phenotypes. Because numerous combinations of (a)virulence/host resistance interactions are possible for each of the several common Meloidogyne spp. and their hundreds of host crops, a useful characterization scheme must include a designation of both the nematode species and the resistant host plant species (or crop recipient of wild species?donated resistance genes). Roberts (1995) has worked with multiple resistance genes to M. arenaria, M. incognita, and M. javanica in several diverse host crops, and incorporating some. earlier suggestions put forward by Triantaphyllou (1987), the following scheme for characterizing Meloidogyne spp. (a)virulence variants is offered. I choose the term ""biotype"" for these variants, as a means to circumvent the debate over pathogenicity (implied by ""pathotype"") and to avoid confusion with the existing ""race"" scheme for Meloidogyne species. Triantaphyllou (1987) also argued a case for the term biotype. Biotype is used to describe insect populations differentiated by host resistance, but has only been used for citrus nematode thus far. As suggested by Roberts (1995) biotype is applied to Meloidogyne spp. isolates distinguished by (a)virulence reaction to hostplant resistance factor(s). It is based on a measurement of nematode reproduction (parasitic ability) on a host plant in comparative tests with known susceptible and resistant host?plant genotypes. The index of reproduction is measured by numbers of egg masses (gravid females) or preferably of eggs produced on a differential plant genotype, as a proportion of the numbers produced on a standard susceptible host genotype.
The scheme involves a simple code description indicating virulence or avirulence with respect to a resistance factor in a crop, and is a multiple code if more than one resistance factor (allele, gene, or combination of genes when resistance conferred by two or mom genes) occurs. For example, in Table I a partly hypothetical series of reactions of four M. incognita isolates to tomato resistance genes is given. The biotype code of isolate (or strain) ""DEF' is given as:
M. incognita biotype L.esc 1,2/3,4,5 where Lesc is an abbreviation of the Latin binomial of the host plant species (i.e. Lesc abbreviated from Lycopersicon esculentum for tomato); the numbers preceding the slash symbol (/) represent compatible interactions of the nematode (i.e. virulence) on resistance factors of the same number, in this example virulence on resistance factors l and 2; and the numbers following the slash symbol (/) represent incompatible interactions of the nematode (i.e. avirulence) on resistance factors of the same number, in this example avirulence on resistance factors 3, 4, and 5. Isolate ABC is avirulent on all five tomato resistance factors, and so a 0 numeral is placed before the slash line, to indicate that tomato is a host and the designations are for avirulence to all resistance factors. This prevents possible confusion with a nonhost scenario, where all reactions would also be placed after the slash, but no preceding 0 is applied. Isolate GHI has a combination of virulence and avirulence different from isolate DEF, whereas isolate JKL would be virulent on all resistance factors (Table 2). The isolate names used here are merely for convenience. In practice they have a laboratory? or researcher?designated number, collection site name, etc, and the name should be retained as unique to that isolate.
The scheme is an improvement over the current race designations because if does not allow misinterpretation that the biotypes are unique biological entities. The biotype scheme also accommodates expansion as new resistance factors are identified within a crop host species, and by inclusion of any number of host species in the designation. The potentially large numbers of biotype designations per isolate (because of multiple hosts with resistance) would require a computerized database system, for data storage and updating, from which subsets of data could be selected and published or otherwise circulated. The biotype designations, once published, could easily be incorporated into NEMABASE (see Objective 4) and the knowledge?based advisory system described for W?186. A central collation and organization of biotype data would provide an important framework for analysis of possible trends of homology, divergence, and relationships to host systematics, agricultural histories, geographical distributions, etc. Although the entire biotype designation for any one nematode isolate could be extensive on numerous hosts, in most situations only a small relevant portion of the total biotype designation may be necessary for communication. For example, one may need only to convey the designations relevant to the host plant(s) reported in a research paper (e.g. Lesc biotypes for tomato research). Only biotypes discriminated on those crops studied or involved in nematode management decisions on a cropping sequence would be desirable, e.g. Hvul, S.tub and M.sat designations for M. chitwoodi in a barley (Hordeum vulgare), potato (Solanum tuberosum), alfalfa (Medicago sativa) rotation in the Pacific Northwest (30).
Breeders, seed companies, and nurseries could report biotypes of the common Meloidogyne species designated on a particular host genotype
(e.g. seed of a tomato cultivar with gene Mi would be indicated to control any biotypes designated Lesc 0/1 but none designated Lesc 1/).
Adoption of the proposed scheme will require standardization of the differential tests using acceptable methods (Starr, 1989) and their interpretation to designate biotypes. An accepted numerical assignment of resistance factors must be offered/created and adhered to. A mechanism of making available standard differential genotypes will also be desirable. A weakness of the potato cyst nematode pathotype scheme is the inclusion of differentials with polygenically controlled, quantitatively expressed resistance that do not distinguish pathotypes unequivocally and may not represent the resistance gene spectra within derived commercial cultivars (see Roberts, 1995). Determination of Meloidogyne resistance gene inheritance would strengthen the proposed biotype scheme and identify quantitatively inherited resistance that may give intermediate levels of resistance expression. These could still be incorporated into the designation scheme as a ""resistance factor.""
Host range studies on M. chitwoodi have distinguished two host races determined on alfalfa and carrot differentials, and have revealed a wide host range for this species (Santo and Pinkerton, 1985). The extent of host range variation among populations of M. chitwoodi in the Pacific Northwest has not been completely revealed, but important variations have been noted, and more are expected. For example, host range studies had revealed onion to be a non?host for M. chitwoodi, although subsequently M. chitwoodi was found infecting and injuring field onions (Westerdahl et al., 1993).
Root?knot nematode species show variability for response to resistance genes in several crops, including tomato, cotton, alfalfa, beans, peanut, soybean and others (Cook and Evans, 1987; Noe, 1992; Roberts, 1992a and b; Roberts and Thomason, 1989; Starr, 1990; Triantaphyllou, 1987). Many of these resistance genes have been identified recently, and they provide tools for studying genetic variability of parasitism and virulence in nematodes. Key combinations include M. chitwoodi and potato, M. arenaria and peanut, and M. incognita and M. javanica on numerous vegetables, legumes and cotton. Putative resistance alleles must be assessed for the spectrum of their effectiveness against a broad range of nematode populations. Root?knot populations are in need of a clear and concise biotyping scheme for indexing virulence status (Roberts, 1992b). Although Mendelian?based analysis of genetic variation is not feasible for the self?fertilizing root?knot nematode species, the spectrum of resistance gene and virulence/avirulence responses suggests that gene?for?gene matching systems occur in root?knot nematodes (Roberts, 1995). This is most clearly indicated in crop plants such as tomato or beans where multiple single dominant resistance genes occur that differentially resist root?knot nematode populations (Omwega and Roberts, 1992: Roberts et al., 1990). The sexually?reproducing cyst nematodes are amenable to genetic analysis, and resistance gene interactions between some cyst species and their hosts have been demonstrated genetically (Dong and Opperman, 1997; Dong et al., 1997; Dong et al., 1998; Jones et al., 1981). At least sixteen host races of H. glycines have been differentiated on soybean resistors, and more are likely to be found (Riggs and Schmitt, 1988; Niblack, 1992). As new sources of resistance are discovered, such as soybean PI 437654, they must be studied for differential reactions governed by the considerable genetic variability in H. glycines populations, as rational deployment of resistant cultivars is critical to future sustained soybean production (Dong et al., 1997).
Markers to identify variability: Identification of cyst and root?knot species has been based primarily on morphological characters and host range. Various anatomical, biochemical and molecular characteristics have been used as aids in nematode identification (Hyman, 1996). Species identification based on the inspection of perineal patterns for example, is tedious and time consuming and not always unequivocal. Recently, efforts have been made to develop molecular markers to aid in the identification of root?knot nematodes (e.g., Cenis, 1993; Williamson et al., 1997). In addition, morphological characteristics do not assist in the identification of pathotypes, races, or geographic isolates. Thus, genetic variability within and between populations of root?knot nematodes are still of concern (Cenis et al., 1992). Within the past ten years, biochemical and molecular techniques have been used with greater frequency to identify nematode species, pathotypes, and geographic isolates. These newer techniques, include assessment of biochemical characters (isozymes), mitochondrial DNA markers, DNA restriction fragment length polymorphisms (RFLPs), random amplification of polymorphic DNA (RAPDs), amplified fragment length polymorphisms (AFLPs), and anonymous single copy nuclear (ASCN) loci. The polymerase chain reaction (PCR) (Saiki et al., 1988) has been a significant advance that has increased the ease of DNA analyses for plant?parasitic nematodes. This technology has presented tremendous opportunities for application within nematology. Some of the research on the use of molecular diagnostics to identify nematode species or isolates has been on nematodes not directly addressed by this project. However, the application of the techniques to the cyst and root?knot species is simple and direct, and will become a high priority during this renewed period.
Isozymic variants have been used successfully as characteristics for the identification of rootknot nematode species. It is possible to distinguish between Meloidogyne arenaria, M. javanica, M. incognita, M. hapla, M. chitwoodi, M. naasi, M. exigua, and M. graminicola using isozyme differences in electrophoretic mobility of esterases and malate dehydrogenase (Esbenshade and Triantaphyllou, 1985; 1990). Variation in esterase patterns has allowed identification of different forms within M. incognita and M. arenaria (Cenis et al., 1992). However, isozyme analysis is not sensitive enough to identify single juvenile nematodes, and isozyme variation has not allowed successful characterization of intraspecific variation among populations or isolates.
DNA techniques have been used to distinguish nematode species and populations with marked success. Various DNA polymorphisms that allow identification of the major root? knot nematode species have been found (Burrows and Perry, 1988; Piotte et al., 1992). Differences among Meloidogyne arenaria, M. javanica, M. incognita, M. chitwoodi, and M. hapla using RFLPs generated after PCR amplification of mitochondrial DNA have been identified (Cenis et al., 1992; Powers and Harris, 1993). Restriction patterns of repetitive DNA have been used to distinguish physiological races within the four major Meloidogyne species (Curran et al., 1986), and primers located in the mitochondrial cytochrome oxidase subunit II and in the 16S rRNA have been used to generate different size fragments that contain RFLPs for the enzymes Dra I and Hinf I. The differences allow discrimination of M. arenaria, M. chitwoodi, M. incognita, M. javanica, and M. hapla (Powers and Harris, 1993).
Random amplified polymorphic DNA (RAPD) analysis has been used to distinguish successfully between Meloidogyne arenaria, M. javanica, M. incognita, and M. hapla (Cenis, 1993). A particularly valuable feature of RAPD analysis is that it can be performed easily on small amounts of DNA, such as that from single juveniles or single cysts (Caswell?Chen et al., 1992; Cenis, 1993). Unique RAPD markers may be cloned to develop sequence?tagged site (STS) markers, and this approach has been utilized to develop PCR primers that will allow differentiation of M. hapla and M. chitwoodi (Williamson et al., 1997). Another powerful DNA finger?printing technique uses selective amplification of DNA restriction fragments to generate AFLPs. The AFLP technique offers a more specific, reproducible, and potentially more efficient means of employing PCR technology to identify useful DNA markers (Vos et al., 1995). The more stringent amplification conditions used for AFLPs offer a more reproducible, and thus a more reliable molecular marker method for population studies than do RAPDs. AFLPs can generate 5?to?10 times as many markers as RAPDS (Kaplan, 1997; Kaplan et al., 1998).
Utilizing yet another approach, Vrain et al. (1992) have used a 1.5Kb internal transcribed spacer (ITS) from rDNA that contains RFLPs allowing differentiation of members of the Xiphinema americanum complex. The potential for more wide?spread use of rDNA ITS as a taxonomic marker is excellent (Powers et al., 1997). Characterizing pathotypes or biotypes, races, and geographic isolates of root?knot nematodes using DNA polymorphisms is the next major challenge for these new technologies, and several laboratories are addressing this question. Cyst nematodes are typically identified by host range and morphology. Identification of closely related species and pathotypes using morphology is difficult or impossible. Molecular techniques have provided new approaches to these problems. The potato?cyst nematodes, Globodera pallida and G. rostochiensis, can be identified using appropriate DNA probes (Burrows and Perry, 1988) and by monoclonal antibodies (Schots et al., 1989). DNA probes obtained from repetitive sequences identified in G. rostochiensis, G. pallida, and the G. pallida Pal pathotype are diagnostic, and are useful for the direct identification of individual cysts of these species using dot blot procedures (Stratford et al., 1992). Folkertsma et al. (1996) used AFLPs to distinguish species and populations of cyst nematodes belonging to the genus Globodera. The influence of host?plant selection on populations of H. schachtii have been assessed using AFLPs, and marker shifts associated with host plant have been detected (Kaplan, 1997; Kaplan et al., 1998).
Besal et al. (1987) identified a 500bp mtDNA probe that allowed detection of single females of Heterodera glycines. Mitochondrial DNA RFLPs were found to allow differentiation of H. glycines and H. schachtii (Radice et al., 1988). Kalinski and Huettel (1988) reported on RFLPs from genomic DNA that allowed the race identification within H. glycines. The use of RFLPs to identify races is somewhat time consuming. The advent of RAPD analyses (Williams et al., 1990) has increased our ability to obtain genetic markers indicative of variation among nematode isolates. It is possible to distinguish between Heterodera cruciferae and H. schachtii using RAPD markers, and genetic variability among geographic isolates of H. schachtii has been documented using RAPD markers (Caswell?Chen et al., 1992).
Adaptation and Fitness: The limited studies on nematode adaptation and fitness with respect to genetic selection pressure have been reviewed (Caswell and Roberts, 1987; Triantaphyllou, 1987; Viglierchio 1990). Plant?parasitic nematodes have been shown to adapt to resistant plants and to temperature. Adaptations appear to be genetically stable in some cases, although tests for fitness are not conclusive. Selection in root?knot populations for virulence to resistance gene Mi in tomato has been achieved by artificial selection (Riggs and Winstead, 1959; Roberts et al., 1990; Dalmasso et al., 1991; Jarquin?Barberena et al., 1991) and has been observed in the field (Kaloshian et al., 1996). Naturally?occurring virulent populations also have been found (Roberts et al., 1990). Artificially?selected populations appear variable in genetic stability of the acquired virulence and in their relative fitness (Riggs and Winstead, 1959; Jarquin?Barberena et al., 1991). Some of us have found artificially?selected isolates difficult to maintain in culture, suggesting decreased fitness. Some artificially?selected isolates virulent for resistance in tomato apparently lost their parasitic ability on susceptible pepper plants (Castagnone?Sereno et al., 1992), also suggesting costs to fitness associated with virulence selection. These unresolved questions of genetic variability have obvious agricultural implications for resistance and rotation planning.
Selection for virulence has also been demonstrated for some cyst nematodes (Turner, 1990; Whitehead, 1991). Continuous exposure of avirulent H. glycines populations to resistance in soybean caused dramatic increases in the frequency of virulent individuals in the population (Young, 1992). Experiments designed to subject different geographic isolates of H. schachtii to selection have revealed that the isolates respond differentially to the selection (Kaplan, 1997; Kaplan et al., 198). Field plots infested with Globodera pallida were planted with resistant potato cultivars, and after 8 years, the G. pallida populations grown on the resistant cultivar had an ability to parasitize the resistant cultivar that was greater than populations on susceptible cultivars (Beniers et al., 1995). Interestingly, there was no correlation between virulence and reproductive rate on a susceptible cultivar (Beniers et al., 1995). Detailed tests to determine baseline frequencies for virulence, rates of mutation to virulence, and population fitness, are rare. Thus, as previously stated, the relative importance of mutation compared to maintained variability in field populations is unclear. Analysis of G. rostochiensis inbred lines near isogenic for virulence and avirulence to gene HI resistance in potato is underway, using RFLP and protein comparisons (Bakker et al., 1992).
Management and Database Needs: Nematode management in the absence of nematicides requires knowledge of species diversity, status of the soil biology, a means of identifying resident nematode populations, and host?status of potential crops to resident nematode populations. Alternative management often requires rotation of crop plant species and cultivars to reduce nematode numbers and damage. Rotations and deployment of resistance are selection pressures that can result in nematode populations being dominated by resistance?breaking (virulent) pathotypes (Caswell and Roberts, 1987). Information on the host range of nematode species and races is fundamental to the successful integration of management tactics, and to avoid selection for virulence and expanded host range. It is difficult to obtain such information, and consequently it is difficult to provide management advice to clientele in a timely manner.
Alternatives to nematicides include: growing nematode?resistant cultivars, growing nonhost primary crops (rotations), growing nonhost cover crops, using fallow periods, enhancing natural biological control, and implementing cultural practices. Each of these choices is made in relation to the nematode species, pathotypes or races, and environmental conditions at a given location. For example, the use of cultivars resistant to the main nematode species is effective. Frequently, however, several genetically distinct subpopulations (pathotypes or races) are present in a field, and the cultivars with resistance to multiple pathotypes are rare. In addition, nematode species differ in their host ranges, and plants differ in their host status to various nematodes. An abundant, although incomplete and often inaccessible, literature exists on many different plantnematode interactions, and a primary goal of Objective 4 is to organize such existing information.
A classic example of the importance of genetic variability in management is the presence of host races within Meloidogyne spp. Despite parthenogenetic reproduction, these species are polyploid and have substantial genetic variability, making race designations, and therefore management difficult (Roberts, 1990). Pathotypes or races of Heterodera schachtii (Muller, 1992), H., glycines (Schmitt and Riggs, 1989), and other cyst nematodes are well known. The cyst nematodes have substantial genetic variability, and much is probably as yet unrecognized, although such variability makes management difficult. The opportunity for genetic recombination available with the sexual mode of reproduction exhibited by cyst nematodes doubtlessly provides for generation of new variants that can not be achieved by the obligate parthenogenetic reproduction in many of the agronomically important Meloidogyne spp. Genetic variability is especially critical when management depends on the use of resistant cultivars or rotation sequences, and knowledge of genetic variability is necessary for deployment of resistance alleles or selection of crop rotation sequences. The design of successful rotations requires the ability to assess the genetic variability in a given field, and subsequent access to the available information on host status relative to that genetic variation.
Toward the adoption of integrated nematode management, with consideration of genetic variability within nematode species, the development of a user?friendly, knowledge?based (""expert"") system has been initiated. The knowledge?based system will utilize and extend the information contained in the nematode?host plant database developed during the previous iteration of W?186 (Caswell?Chen et al., 1995; Ferris et al., 1995). The system will aid growers and processors in making nematode management decisions, specifically regarding nematicide application, and crop and cultivar selection. The system will be available via the Internet and World Wide Web (WWW)).
Other Regional projects:
Two previous projects in the southern region (S?154 and S?212) dealt with variability in selected cyst (H. glycines) and root?knot nematodes (M. arenaria and M. incognita). The variability was assessed with host differentials, and regional bulletins covered some of the findings (Riggs, 1982; Starr, 1989). However, those studies were not as extensive as the research proposed in this project, both in terms of the broader range of nematodes we plan to study, and especially with respect to the application of new molecular techniques that were unavailable for earlier projects. The proposed western region project takes up from where the southern projects finished. A previous western region project (W?134) focused on the general population biology of plantparasitic nematodes with respect to quantitative aspects of population density estimates, and the relationships between nematode population densities and their damage potential to crops for predictive management purposes. A bulletin of the primary findings has been published (Griffin and Roberts, 1994). The W?134 project did not consider nematode genetic variability as a research focus, but the results of the project did indicate the need for detailed research on genetic variability.
Objectives
-
Characterize genetic variability as related to PPN responses to resistance, environment, biological processes, and morphology
-
Determine nematode fitness and adaptability relative to environment, host plant, and host plant resistance
-
Design and develop management strategies for cyst and root-know nematodes relative to genetic variability
-
Methods
An organizational structure of four elected research coordinators will be used to enhance research planning and reporting. Each coordinator will be responsible for one of the three main target nematode Groups, and a fourth coordinator will have responsibility for database and information transfer relating to Objective 3. Each coordinator will have responsibility for 1) organizing research planning activities for the assigned nematode group, and 2) coordinate and summarize research results for reporting within the project and for external reporting. This structure should help to avoid unnecessary duplication of experimental effort, and also ensure that important gaps in methodology or nematode and plant materials will not occur. The research focus of each participating state is given in Table 1. The nematode group and main crop areas are indicated, together with the procedural research emphasis, as covered under the three objectives. The detailed procedures will vary somewhat according to the biological requirements and agricultural focus of each participating state, but the research coordination will ensure that standardized procedures are generally followed, so that research findings can be compared within and across nematode, plant and subject area categories. Following the description of procedures by objective, specific procedures and contributions of participating states are detailed in Appendix D. Experimental protocols and procedures are structured according to the three main target nematode groups under the three objectives. All objectives have a common focus of addressing critical aspects of nematode genetic variability, with the application and extension of the findings tailored to meet individual state and sub?regional needs, in addition to those at the project?wide level. While findings under all three objectives are considered important to each state, duplication will be avoided by partitioning individual state research activities. This structure will also ensure that all phases of the objectives are being met, while addressing local state needs. For example: Heterodera schachtii will be considered in California, Hawaii, Idaho, and Wyoming; Heterodera glycines will be considered in Arkansas, North Carolina, Michigan, and Nebraska, while the Group II cool?climate root?knot species (Meloidogyne chitwoodi and M. hapla) will be studied in detail in California?Davis, N. Carolina, Nebraska, Oregon, Idaho, and Washington. However, N. Carolina, Oregon, Idaho, Washington, and Wyoming will focus on potato and (or) small grain interactions. North Carolina, Washington, New Mexico, and California will focus on host?plant resistance, while Oregon, Idaho, and Wyoming will address host range and rotations, California, Idaho, and Wyoming will address cover?cropping. Similar partitioning of research activity will be made for the other nematode groups. Standardized procedures will be used for assessing of genetic variability (Objective 1), for analysis of nematode adaptability and fitness under selection (Objective 2), and for design and development of management strategies (Objective 3) (e.g., Barker and Davis, 1996; Cardwell et al., 1996; Dong et al., 1997; Hyman, 1996; Hyman and Whipple, 1996; Kaplan, 1998; Powers and Harris, 1993; Roberts, 1990; Roberts 1992b). Plant germplasm (accessions, breeding lines, cultivars) and nematode isolates of representative species and population variants will be available among the participants to maximize the comparative value of the research findings. Likewise, DNA probes for markers will be shared among the molecular?focused participant programs under the activities of Objective 2. Objective 1: Characterize genetic variability as related to PPN responses to resistance, environment, biological processes, and morphology. Phenotypic assessments will be made on isolates of nematode populations that are collected following survey and documentation of habitat, locality, and agronomic or cropping history of the collection site. This information will provide important background considerations for the level and nature of any phenotypic differences detected in comparative experiments. In most programs, investigators either have a partial or nearly complete collection of live cultures for genetic comparisons. For example, about twenty?five isolates of diverse warm?climate root?knot populations have been assembled at California?Riverside, and extensive collections of M. chitwoodi population isolates have been made and are being increased in Oregon and Washington (Cardwell and Ingham, 1997; Mojtahedi et al., 1994). For cyst nematodes, numerous diverse populations of H. glycines are being cultured at Arkansas, N. Carolina, and Michigan, and a collection of H. schachtii and H. cruciferae geographic and host?selected isolates is under culture at Hawaii and California?Davis (Caswell?Chen et al., 1992). Deficiencies will be met by additional collecting and by exchange of materials between participants. The cultures will be maintained in greenhouses under standard conditions suitable for each nematode species, and grown on standard susceptible host plants. For example, M. arenaria is cultured on peanut cv. Florunner, M. hapla, M. incognita and M. javanica on tomato cv. Rutgers, M. chitwoodi on wheat cv. Nugaines, and H. cruciferae and H. schachtii on cabbage cv. Copenhagen Market. Assays of virulence/avirulence response to resistant lines and cultivars and of host range will be made under greenhouse and controlled environment conditions using well?established experimental procedures (Barker and Davis, 1996; Noe, 1985; Omwega et al., 1988; Sasser and Carter, 1985; Starr, 1990). Key elements of these procedures are 1) use of nematode eggs or second?stage juveniles as inoculum; 2) running experiments at known temperatures to determine termination time based on accumulated heat units required to complete one or two generation cycles; 3) determine reproductive capacity of nematodes based on egg production indexed by the root?weight of inoculated plants; 4) analyze using standard statistical treatment of appropriate replicated experiments; 5) include known standard resistant and susceptible, or host and nonhost, control plants. The host range and virulence testing for the root?knot species (Groups I and II) will include the standard set of host differential plants (tomato, pepper, tobacco, cotton, watermelon, peanut) used to differentiate species and races of common Meloidogyne spp. (Sasser and Carter, 1985), and alfalfa, carrot and wheat for M. chitwoodi and M. hapla species and race separation (Santo and Pinkerton, 1985). In addition, investigators will include differential cultivars and crop plants applicable to their local cropping systems, as given in the Appendix D contribution statements. The biotype scheme for root?knot nematode will be vigorously pursued, using the simple code description indicating virulence or avirulence with respect to a resistance factor in a crop, a multiple code if more than one resistance factor (allele, gene, or combination of genes when resistance conferred by two or mom genes) occurs. The virulence biotyping will be made using known accessions, breeding lines, and existing cultivars of critical crops for the target nematodes. M. chitwoodi will be examined using diverse resistance gene sources in potato and related Solanum spp. gene donors (Brown et al., 1995, 1996, 1998; Mojtahedi et al., 1995; Santo et al., 1994). M. arenaria will be examined on diverse plant introductions and breeding lines of peanut, and related Arachis spp. materials. M. incognita and M. javanica will be examined on accessions, lines and cultivars of several crops including alfalfa, beans, carrot, cotton, cowpea, grape, potato, soybean, tomato and wheat, known to carry specific and different genes for resistance. For the cyst nematodes (Group III), H. glycines will be examined on resistant soybean differentials (Riggs and Schmitt, 1988; Niblack, 1992), including PI 437654 and cv. Hartwig, and inbred lines of H. glycines will be used for genetic analysis of virulence (Doug et al., 1997). H. schachtii will be studied on sugarbeet breeding lines with resistance derived from different wild Beta spp. (Muller, 1992; Cai et al., 1997). The other endoparasitic nematode Rotylenchulus reniformis (six populations) will be examined together with M. javanica on pineapple lines in Hawaii. The cyst nematodes are excellent ""model"" nematode systems for genetic studies because they reproduce sexually (bong et al., 1997). Conversely, many root?knot species are thought to be clonal due to parthenogenetic (asexual) reproduction. Current standard molecular biology techniques will be used for this objective, and the protocols and techniques, although new, are well established. Techniques for RFLP analysis of genomic (Piotte et al., 1992), and mtDNA (Powers and Harris, 1993) are well established and are being used in many of the project participants' laboratories. Techniques for RAPD analyses of single cyst and root?knot nematodes are available (Caswell?Chen et al., 1992; Cenis, 1993) and are being used more widely in nematology to identify genetic variation among populations. The additional techniques of AFLP (Vos et al., 1996) and ASCN (Karl and Avise, 1993) will be utilized (Hyman, 1996). If possible, the transmission genetics of phenotypes of interest will be assessed by segregation analyses of genetic markers (Dong et al., 1997; Dong et al., 1998; Dong and Opperman, 1997). Heterodera schachtii, H. glycines, and H. cruciferae populations will be characterized using mtDNA, RFLPs, RAPD, and AFLP analyses. Working from individual nematodes, a number of nuclear and mitochondrial genes will be compared for the nematode species in Groups I?III. Markers diagnostic for species or for phenotypic traits of interest will be sequenced to define new primer sets that have higher specificity (e.g., Williamson et al., 1997). The new primer sets will be used to identify field subpopulations that have characteristics of interest, and to determine the distribution of characters across geographic ranges. This will also allow us to monitor changes in gene frequency in fields, as we subject field populations to different cropping sequences. Our ability to amplify DNA from sip? ' ?. juveniles will allow us to assess inheritance and transmission genetics of interesting phenotypes, such as host range variants, resistance ?breaking pathotypes, or temperature?adapted geographic isolates. Analyses of H. schachtii and H. cruciferae in California will provide a model for assessing variability of California isolates tentatively identified as Heterodera avenae and H. mani. The emphasis on H. glycines at North Carolina and Arkansas with populations from other states such as Nebraska and Michigan will broaden the molecular characterizations of the various cyst species. Meloidogyne species populations will be typed phenotypically for virulence/avirulence with respect to numerous host plant resistance genes from different crop plant species and close wild relatives under Objective 1. These will provide a unique set of intraspecific variants from several root?knot species (M. chitwoodi, M. incognita, M. javanica, M. hapla, M. arenaria). Biochemical and molecular marker analyses of these populations, or selected subsets, will be made in labs of the collaborating project investigators to determine the potential for providing stable marker systems for nematode virulence/avirulence phenotypes. This approach may provide insight into genetic relationships common or unique in nematodes for reaction to resistance genes possessed by different crops. The quest for genetic markers specific for rootknot nematode races will continue in many participating states, with the primary techniques being mtDNA, RFLPs, and ASCN markers. For example, root?knot populations from cotton will be characterized on the basis of esterase isozyme profiles and DNA homology using diagnostic sequences. Different states will focus on particular species. For example, the diagnostic sequences will enable comparisons of genetic variability with M. incognita populations from cotton?producing states in the project, including Arkansas, California, New Mexico, and N. Carolina. Oregon, Washington, and Idaho will focus on M. chitwoodi and M. hapla in the potato?small?grain?alfalfa system. Under this objective, extensive collaboration among the participants is planned, because of the range of molecular expertise existing between participants. In each participating state, nematode populations of the Group I?III species will be characterized using combinations of the above techniques. For example, in Arkansas three populations of H. trifolii, ten of H. glycines, two of Cactodera betulae, five of M. incognita and several populations of M. hapla will be characterized according to RFLP, RAPD, and AFLP and other appropriate molecular techniques. The M. incognita populations will be selected from cotton, soybean, watermelon, or pasture. Similar types of examinations will be conducted with Meloidogyne spp. in California, Oregon, Nebraska, N. Carolina, and Washington. Objective 2: Determine nematode fitness and adaptability relative to environment, host plant, and host plant resistance. Two primary aspects of nematode adaptability and fitness will be pursued, that concerning host range and virulence, and that concerning adaptation and fitness to environmental factors, such as temperature and host nutritive status. Phenotypic characterization and genetic markers developed under Objectives 1 and 2 will provide the basis for selecting candidate populations that show attributes of variant adaptation to increased virulence and parasitism, and for variants associated with geographic (climatic) isolation and adaptation. The fitness of isolates found to be virulent for specific resistance genes will be assessed by culturing the nematodes on a susceptible cultivar of the same crop, and on different susceptible crops, under controlled conditions for multiple generations. Fitness will be assessed in a strict sense, as altered reproductive potential. A second approach to studying adaptation and fitness will be to conduct re?inoculations of rootknot or cyst individuals able to reproduce on resistant plants, back onto the same plant genotypes. This will impose continuous selection pressure for shifting from avirulence to virulence in wildtype populations. Changes in reproductive capacity over repeated re?inoculations will be assessed after each mean generation time, as a measure of virulence/avirulence frequencies. Any virulent selected subpopulations will be compared to the wild?type parent population for reproductive capacity on susceptible host plants, and for changes in genetic markers. This will measure the genetic stability of the virulence and the fitness of the selected subpopulation. Tests of selected isolates will be made on resistant or nonhost plants other than those used to impose the original selection pressure. Procedures for culturing and assessing reproduction rates will be the same as those used in Objective 1, with modifications for repeated inoculations. These modifications will follow standard procedures for virulence selection (Riggs and Winstead, 1959; Turner, 1990; Jarquin?Barberena et al., 1991). In the field, changes in virulence in H. glycines toward a soybean cultivar carrying specific resistance will be monitored. Continuous culture of the resistant plants in 3?year rotations will be used for assessing selection for higher frequency of virulent individuals in the population (N. Carolina). Adaptation to temperature regimes atypical of normal nematode temperature requirements, and the influence of temperature on resistance will continue to be a priority within the project (Veremis and Roberts, 1996). The influence of temperature on resistance alleles and nematode isolates will be studied by determining reproduction potentials of variant compared to ""normal"" populations of a nematode species under different constant temperatures. These will be conducted in controlled?temperature cabinets or in controlled?temperature Wisconsin waterbaths. Temperatures will include those that are not considered optimal for the particular species. Standard procedures for conducting the controlled temperature studies will be used (Inserra et al., 1983; Omwega and Roberts, 1992). In further consideration of the environment, the fitness and the adaptability of the H. glycines races and M. incognita to changes in soil nutrition and soil physio?chemical environments will be tested under controlled conditions (Melakeberhan, 1998a; 1998b). As part of the first phase of the project, sources of nitrogen and types and levels of nutrients have been shown to affect the interactions of M. incognita and/or H. glycines with host?plants of varying degrees of resistance. Therefore, quantifying the adaptability of the nematode races and isolates to changes in soilnutrient environments will be helpful to our understanding of possible factors that may contribute to nematode adaptations. OBJECTIVE 3: Design and develop management strategies for cyst and root?knot nematodes relative to genetic variability. The development of biotyping schemes for cyst and root?knot nematode populations for reaction to host and nonhost crops and to resistant cultivars of susceptible crops under Objective 1, and the potential for accompanying practical marker systems under Objective 2, will provide important information. This can be utilized by production agriculture for resistance implementation in annual cropping systems. Crop rotation sequences utilizing only poornonhosts grown in rotation with hosts at various intervals will be developed and evaluated for success in nematode management and profitability. Climatic conditions, different nematode species, and potential variability in host ranges among different populations will require that unique rotations be developed for the different growing regions covered by this project. The assessment of rotation schemes will be made in microplot or natural infested field sites within the relevant localities. The research coordinator will aid use of standardized field plot designs for rotation and resistance implementation studies. These will be based on randomized complete block, split?block and split?plot, and factorial designs with four or five replicates (Barker, 1985c; Little and Hills, 1978; Nelson, 1985; Noe, 1985; Starr, 1990) Standard procedures for sampling and extraction of nematodes from roots and soil will be used that quantify population densities and reproduction according to unit volume or weight of soil or roots, and are corrected for extraction efficiency and sampling intensity (Barker, 1985a&b; Roberts and Thomason, 1981; Riggs and Wrather, 1992). These techniques will be coordinated among the project participants to facilitate direct comparisons of results on different nematodes and cropping systems in various localities. Transfer of this information as guidelines to growers, pest control advisors, commercial and public plant breeders, and seed company personnel will involve the development of written materials, together with the use of a computerized database format that can be accessed centrally as described below. Information regarding the host status of plants to different nematode species is necessary to design rotations. However, the considerable information in the literature is spread out and difficult to access when needed for management decisions. At California?Davis, project participants Drs. Ferris and Caswell?Chen have created a personal computer database so that a current, accessible compilation of the information on nematode?plant interactions is readily available (Caswell?Chen et al., 1995; Ferris et al., 1995). The goal has been to complete a database that will assist in providing information on resistant cultivars, rotations, and covercropping for nematode management, and it currently contains approximately 45,000 records on nematode?plant interactions, culled from the primary nematological literature. The database is accessible via the WWW using a standard browser that is pointed to the following address: (http://ucdnema.ucdavis.edu/Tango/Tango.acgi$/Tutorial/INTERACT.QRY?function=form). One of the goals under this objective is to add and modify information in the database, so that the influence of genetic variability within species relative to host range is considered. The database content will thus be modified by participating states within the project. Such information will have applied and basic value. The database will assist in defining acceptable rotations or selection of appropriate resistant cultivars, an important application. The database will also allow evaluation of genetic variation within species across wide geographic areas. Such information will assist in a critical evaluation of current morphological species and designations, and races defined by differential hosts. The extant database will be used to develop a knowledge?based advisory system for nematode management. Scientific progress, with attendant proliferation of reductionist knowledge has led to overwhelming amounts of information, and a proliferation of informational databases (e.g., Caswell?Chen et al., 1995; Eeckman and Durbin, 1995; Ferris et al., 1995; Shoman et al., 1995). Although the reductionist research strategy is highly effective in generating information, it does not directly lead to access, organization, integration, and interpretation of information; the vital components that allow application of knowledge in practical, real?world problem?solving activities (Schmoldt and Rauscher, 1996). There is a critical need to be able to access and interpret knowledge on nematode management. Access to information via the WWW only assists in implementation, because access to informational databases is only one component necessary for application of knowledge. Nematode management relies on the synthesis of a range of information for informed decisions (Thomason and Caswell, 1987), and effective management strategies potentially involve the manipulation of multiple system attributes to achieve management objectives. A semantic network approach is being used in the development of the knowledge?based system (Lucas and van der Gaag, 1991; Schmoldt and Rauscher, 1996). When the system is functioning, the user will provide input to the system, including: the nematode species, the nematode ""race,"" the nematode density, the crop from which soil samples were taken, the desired crops for the next three years, willingness to use nematicides (yes or no), soil type, geographic location (user will also be able to note nearest location to specific cities), and priorities of management objectives (improving yield, growing high value crop, prefer short?term or longer?term benefits, and reducing nematode population increase). a) In response to user input the shell will inform the user of whether their planned cropping sequence (with up to a 3?year time window) will exacerbate their nematode infestation. b) The user will be informed of all possible plants and cultivars that are resistant to their problem nematode. c) The user will be informed of possible crop sequences that will not exacerbate nematode problems. d) The user will be informed of possible nematicides that could be used for the potential crops. e) The user will be provided an economic synopsis of costs for management.Measurement of Progress and Results
Outputs
- The research conducted under the auspices of this project will provide the information necessary to implement nematode management alternatives to nematicides. The project will be instrumental in evaluating new sources of resistance against a broad array of nematode genotypes, an approach that has not been used previously in bioassays for resistance. The molecular determinants of virulence and resistance will be much more completely understood based on results from this project. The phenotypes associated with nematode genotypes will be documented, and the ramifications relative to nematode management will be documented and investigated. This project will provide the insight necessary for developing methods that will assure the more successful deployment of resistance in sugarbeet, tomato, cowpea, cotton, soybean. Lastly, significant nematode management options will be further developed and made widely available via a knowledge?based nematode management advisory system.