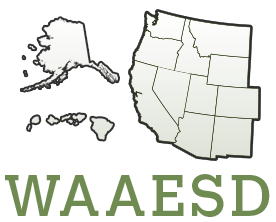
W168: Seed Biology, Technology, and Ecology
(Multistate Research Project)
Status: Inactive/Terminating
W168: Seed Biology, Technology, and Ecology
Duration: 10/01/1998 to 09/30/2003
Administrative Advisor(s):
NIFA Reps:
Non-Technical Summary
Statement of Issues and Justification
STATEMENT OF THE PROBLEM:
Seeds are fundamental to U.S. agriculture and natural ecosystems. Fundamental knowledge about mechanisms underlying seed development, germinability, dormancy, and storability is required to improve seed performance in the 21st Century. This information is needed to maintain the global competitiveness of the U.S. seed industry, increase food production, improve weed control, and take full advantage of advanced technology. In addition, a clearer understanding is needed of how environmental factors affect seed performance.
JUSTIFICATION:
Overview. Seeds are fundamental. Seeds are one of the most powerful forces in nature because they ensure the reproduction of most plant species. Seeds are the starting point for the production of annual crops. Of all the major human food sources in the world, only fish is based on a biological food chain that does not begin with seeds. The lives of 99% of the worlds population either directly or indirectly depend on seeds (Urmstrom, 1997).
Production of high quality seeds is fundamental to the success of U.S. agriculture. Crop production relies heavily on high quality planting seeds. Every farmer is sensitive to the need for rapid, uniform seedling emergence because it is the foundation on which stand establishment is based and potential yield is determined. The average American farmer spends over $2,500 on seeds for crop establishment annually. Total U.S. expenditures for seeds and transplants are over five billion dollars annually (USDA estimates).
The unique biology of seeds as life in a suspended state and the specialized nature of the seed industry have given rise to Seed Biology as a distinct scientific and technical discipline. During the last three decades, this discipline has provided the American seed industry with the biological understanding and technical expertise needed to deliver a stable supply of the finest seeds to the U.S. agricultural industry and the world market. Most of the crops contributing to the $85 billion in annual U.S. agricultural productivity are grown from seeds, and the seed industry is a significant agricultural sector in its own right, accounting for approximately $4.2 billion in U.S. market value (USDA estimates). In addition, exports of agricultural seeds were responsible for a positive trade balance of over $409 million for fiscal year 1995/96 (FATUS, 1996).
Renewed emphasis will be placed on maximizing the quality and performance of seeds in the next century to meet the demands of emerging technologies. For example, the physiological quality of seeds is of increasing importance in conservation tillage and revegetation programs, where seeds are often planted in hostile environments under adverse seedbed conditions. In these cases, establishment and survival of the seedlings is the most crucial step in the success of the entire enterprise. Rapid advances in biotechnology underscore the importance of maintaining all germplasm resources indefinitely into the future. These genetic resources, preserved in seeds, will provide the diversity upon which future advances in agricultural productivity will depend. Germplasm preservation assumes renewed importance since all genes are now potentially available for utilization in crop improvement. Seeds are also the vehicle for delivery of plant biotechnology to the field. Farmers will be required to invest greater capital in seeds derived from biotechnology or that incorporate value added treatments such as priming, coating, or optical sorting. Furthermore, the Global Positioning System (GPS) and Geographic Information Systems (GIS) have lead to the development of a new generation of farm equipment that allows farmers to vary seeding rates to match soil fertility. The benefits of these sophisticated technologies can best be utilized if seed performance is optimized.
Problem. During the previous five years of the W-168 project, progress was made on the two approved objectives. The objectives for the next five years have been redefined to include new problems of national concern in the next century. The new objectives are intentionally broad because a holistic approach is needed to investigate the large and diverse field of plant biology.
The new objectives address the following problems. Despite recent advances in understanding the molecular biology of seeds, we still know relatively little about how seeds germinate, why some seeds germinate better than others, what causes dormancy, and why seeds die in storage. New fundamental knowledge about mechanisms underlying seed development, germinability, and storability is required to solve the challenges of the 21st century that were described in the previous section. Seed performance must be improved: (1) For the U.S. to maintain our global competitiveness as an exporter of seeds as propagules. (2) To help increase food production in the next century. (3) To take full advantage of advanced technology. A clearer understanding of how environmental factors affect seed performance in natural as well as agricultural ecosystems is needed to ensure the continued vitality of native plant populations and the productivity of cropping systems.
The Coalition for Research on Plant Systems (CROPS) was organized to determine societal needs and to develop a comprehensive, coherent national research strategy. At the a national workshop entitled CROPS 99 held on November 9 to 11, 1997 in St. Louis, Mo., four research goals and 19 specific priorities were identified for the next century. These goals and objectives have been endorsed by more than 75 organizations, representing agricultural and commodity groups, scientific societies, industry, and government agencies. The research objectives outlined in this proposal for the next 5 year period contribute substantially to three of the four objectives and the following 9 CROPS priorities (CROPS, 1997).
- Conduct structural and functional genomics, initially focused on economically significant plant species.
- Explore plant diversity and genetics to identify unique traits with potential to produce new and improved products.
- Conduct structural and functional genomics on microbial, insect, nematode, weed, and other pest species.
- Preserve, analyze, and utilize genetic resources and diversity of germplasm of plants and associated organisms.
- Develop handling, post-harvest storage and processing systems to assure safety and quality while minimizing product loss.
- Strengthen the understanding of the interaction and compatibility among natural, urban, and agricultural ecosystems.
- Expand the understanding and use of plants and microorganisms
to remediate environmental problems economically and effectively.
- Develop methods to detect and quantify value-added traits in plants and their products to enhance the profit share of the grower producer.
- Develop the science applied to site-specific agriculture, including precision agriculture, for profitable production and minimal adverse environmental impact.
Among the top eleven national research priorities established by the Experiment Station Committee on Organization and Policy (ESCOP), the seed biology research objectives in this proposal contribute substantially to nine (ESCOP, 1994):
Need for a cooperative approach.
This Regional Research Project was originally initiated in the Western Region because of the concentration in this area of seed production of many horticultural, forage, and native species. Yet, the importance of seed production extends beyond this geographical area, as the U.S. is a major world producer of cereal and oil seeds as well as forage, vegetable, and flower seeds. Seeds of native plants for revegetation and forestry use are increasing in importance as well. Seeds are produced throughout the U.S. and are utilized throughout the world. For example, seeds of cool-season grasses, carrots, beans, alfalfa, sweet corn, and cole crops are produced in the Pacific Northwest. Diverse vegetable and flower seeds as well as rice, wheat, hybrid sunflower, cotton, and clover seeds are grown in California and the Southwest. Soybeans, corn, and sunflower seeds are grown in the Midwest. Revegetation shrub and chaffy grass seeds are produced in the Great Plains states. Peanut, cotton, and fir tree seeds are produced in the Southeastern states. These examples of the diversity of seed production throughout the U.S., and the lack of any other regional projects devoted to seed biology or technology, emphasize the role this project has played in expanding beyond a Western Regional effort to become a truly national research mission.
Despite the diversity of species and locations involved in this project, fundamental aspects of seed biology are common to all. For example, certain patterns of gene expression and the accumulation of storage products are shared across many species. Understanding dormancy, one of the primary objectives for the project, actually requires an array of examples due to the diversity of mechanisms and adaptations possible. Measurement and enhancement of seed quality presents similar challenges and opportunities regardless of the species or location. It is precisely by examining seed biology from diverse perspectives, from the ecological to the molecular, that the entire biological picture becomes clearer and specific applications can be devised.
The breadth of W-168 research is one of its strengths because seed biologists with diverse interests ranging from molecular biology to ecology can share their expertise. Regional Research Project W-168 provides an interdependent structure within which seed scientists across the U.S. can establish critical research objectives, focus and combine their efforts on projects of mutual interest to solve problems, discuss and critique results and hypotheses, and contribute to expanding scientific knowledge.
The W-168 project has a history of productive collaborative research. During the past 5 years, two W-168 project collaborations have obtained a BARD grant with scientists in Israel, and 29 collaborative publications have resulted from the W-168 project. Collaborations between W-168 members have resulted in undergraduate, graduate, and post-doctoral training opportunities. With recent declines in the number of both graduate and undergraduate seed training programs at land grant universities in the U.S., W-168 members will increasingly be looked upon to train the next generation of scientists and technicians for the seed industry. Student training and experience received from W-168 collaborative research, annual meetings, and symposia will continue to be an important aspect of the W-168 project in the future.
Seed quality is important to virtually all sectors in U.S. agriculture and in all regions of the country. Both foreign and domestic seed companies maintain production and research facilities at various locations throughout the U.S. due to the climatic diversity, the ability to produce high quality seed, skilled farmers who specialize in seed production, and the size of our domestic markets. Seeds are increasingly produced in one location and marketed in another. This interstate and global commerce requires a high quality product capable of withstanding the rigors of shipment and storage and performing reliably under a diverse range of field conditions. Meeting these demands requires cooperative research efforts in both production and utilization locations."
Related, Current and Previous Work
Relationship to other projects: There are at least three professional associations (AOSA, SCST, ISTA) dedicated to seed testing and an array of other organizations (AOSCA, AASCO, ASTA) concerned with the genetic, pathological, and physiological quality of seed. While these organizations support seed research, they do not generate fundamental research information. W-168 is the only research project currently focused solely on seed biology and technology. There is no significant overlap between the objectives of W-168 and other existing research projects.
A search of the CRIS database revealed five projects besides W-168 that peripherally deal with seeds as propagules. Cooperative research project NC-202, Biological and Ecological Basis for Weed Management Decision Support Systems to Reduce Herbicide Use, deals with weed management in corn-soybean production systems. One objective of this project is to develop weed emergence and seed bank information needed for corn-soybean weed management. Project S-279, Sustaining and Improving Profitability of Wheat/Stocker Cattle Enterprises in the Southern Great Plains, has one objective dealing with effects of genotype and environment interactions on wheat seedling emergence at various planting dates. Project NE-124, Genetic Manipulation of Sweet Corn Quality and Stress Resistance, deals primarily with sweet corn breeding and lists the study of physiological mechanisms regulating seed quality as an objective. Projects NC-007, Plant Germplasm and Information Management and Utilization, and NE-092, Integrating Cover Crops, Cultivation, and Herbicides to Optimize Weed Control, were listed in the CRIS database as seed research projects, but specific objectives for these projects were not listed. With the exception of NC-007, these projects deal with seeds only as they relate to problems with the production of specific crops. Other projects do not deal with problems of seed quality, dormancy, or longevity that plague many crop species or are of concern in natural ecosystems. Also, no existing project deals specifically with seed quality issues that relate directly to U.S. agricultural industries or the performance of seeds in nature.
The CRIS database presently lists a total of 98 active projects dealing with seed biology. Roughly 1/3 of these projects represent the current efforts of participants in the current W-168 Regional Project. There is no significant overlap among these projects. Many of the projects listed deal with seeds as food or livestock feed and are not concerned with the production and quality of seeds for planting or germplasm conservation. What is evident from reviewing individual projects is that the discipline of Seed Biology is multi-faceted, and a wide range of expertise is required to pursue fundamental scientific advances and translate that information into new technological solutions and practical agronomic practices.
Progress Made during the last Five Years of the W-168 Project.
Seed development - Our understanding of the biology of seed development has progressed rapidly in recent years. Seeds develop through a series of stages that may be tracked at morphological, physiological, and molecular levels. At each stage of seed development (early expansion, storage reserve accumulation, post-abscission, desiccation, and germination), specific gene expression patterns are evident and different complements of proteins are synthesized (Goldberg et al., 1989; Hughes and Galau, 1989). While the storage reserve accumulation phase has received the most research attention from the viewpoint of maximizing yield and nutritional content, the extent of reserve accumulation may not be the most important factor for seed quality. The W-168 project will continue research on seed development to identify molecular markers for seed quality and uncover fundamental information on seed development that will ultimately help the seed industry to produce higher quality seeds.
Understanding how developing seeds acquire desiccation tolerance is important because desiccation tolerance is one of the unique properties that may be related to seed longevity in storage. Sugars, maturation proteins, and free radical scavengers have been proposed to facilitate desiccation tolerance in developing seeds, and a low ratio of sucrose to the raffinose series (raffinose, stachyose, verbascose) of soluble oligosaccharides correlates to the half-life of seed storability (Horbowicz and Obendorf, 1994). Structures of several series of galactosyl oligomers of sucrose and cyclitols that may be involved in seed maturation, desiccation tolerance, and/or storability have been illustrated (Obendorf, 1997). Stachyose accumulation correlates with the onset of desiccation tolerance during slow drying of immature soybean embryos (Blackman et al., 1992) and has been confirmed for zygotic embryos matured in planta and in vitro (Obendorf et al., 1998a; Obendorf et al., 1997). A decline in myo-inositol and an increase in galactinol immediately preceding an increase in raffinose and stachyose are consistent with galactinol being the galactosyl donor for raffinose and stachyose biosynthesis. Galactopinitol A, galactopinitol B, and fagopyritol B1 (all galactosyl cyclitols) accumulate in axis tissues of developing soybean seeds in planta in association with the onset of desiccation tolerance, yellowing of axis tissues, and in parallel with stachyose accumulation (Obendorf et al., 1998b). Accumulation of pinitol and galactopinitols appear to be impaired during in vitro maturation of soybean zygotic embryos in liquid medium (Obendorf et al., 1997) and during maturation of somatic embryos (Obendorf et al., 1997; Horbowicz et al., 1995). Impaired ability to accumulate stachyose and galactopinitols may partially explain the reduced growth rate of seedlings produced from somatic embryos and zygotic embryos matured in vitro (Obendorf et al., 1997). Maize embryos do not accumulate stachyose or galactopinitols, but accumulation of raffinose in addition to sucrose (sucrose:raffinose ratio having a ratio of 20:1 or lower) correlates with desiccation tolerance in developing maize seeds (Brenac et al., 1997a; Brenac et al., 1997b). Results with several viviparous-1 (vp1) alleles show that desiccation tolerance may be regulated by the Vp1 gene but does not require the expression of a fully functional VP1 regulatory protein. Raffinose biosynthesis and accumulation occur in the absence of a fully functional Vp1 gene (Brenac et al., 1997b). Buckwheat seeds do not accumulate significant quantities of raffinose or stachyose, but become desiccation tolerant in correlation with the accumulation of fagopyritol B1, a galactosyl cyclitol (Horbowicz et al., 1997; Szczecinski et al., 1997). Based on the buckwheat results, galactosyl cyclitols may substitute for raffinose and stachyose in conveying desiccation tolerance and storability in seeds. Except for galactinol synthase, a detailed analysis of enzyme activity, transcription, and translation has not been reported for developing and maturing seeds.
Seed imbibition - Another highly dynamic stage of germination is imbibition. Water uptake is the first essential step to rehydrate the quiescent seed to permit the resumption of active growth. Assuming that water is not limiting from the environment, water uptake in seeds reveals a triphasic pattern with an initial rapid uptake phase, followed by a lag phase and then a second increase in moisture content associated with seedling growth (reviewed by Bewley and Black, 1994; Bradford, 1995). Water uptake has been shown to follow first order reaction kinetics for a range of seed species (Leopold, 1983). However, water movement into seeds may be characterized by two processes: first the initial wetting followed by the bulk flow of water into the hydrated tissue. The wetting front has been studied in pea seeds in time course studies (Waggoner and Parlange, 1976). Wetting is a function of the surface tension and the seed microstructure (cited by Vertucci, 1989). The bulk flow of water is accompanied with an increase in volume (swelling). The swelling coefficient (volume after hydration/initial volume) was estimated to be 2.5 for both soybean and common bean seeds after 24 hours (Leopold, 1983).
The process of imbibition is poorly understood in maize (Zea mays L.) primarily because of the difficulty in separating its contiguous parts (pericarp, endosperm, scutellum, and embryonic axis). The rates of water uptake into maize seed structures were studied using techniques that: 1) preferentially stained starch in the endosperm by iodine; 2) monitored embryo dehydrogenase activity with nitroblue tetrazolium chloride; and 3) determined the movement of 3H2O into seed parts (McDonald, Sullivan, and Lauer, 1994). The results indicated that water uptake was most rapid during the first 6 hours and was greater in the embryo than in the endosperm. At the time of radicle protrusion, seed moisture content increased from 5 to 50-55% in the embryo and to 25-30% in the endosperm. Whole seed moisture content at germination was approximately 31-34%. Placing the maize seed in various orientations in contact with a water source revealed that the portion of the seed containing the embryo consistently absorbed more total water than the endosperm. These studies suggested that when maize seeds were in contact with water, uptake occurred by two separate pathways. The first was primarily by rapid water entry through the black layer, then progressive hydration from the radicle to the shoot. Embryo hydration was nearly completed within 15 hours. The second was primarily by the movement of a defined wetting front that penetrated the pericarp radially and advanced slowly through the endosperm. This process was not completed even after 48 hours of soaking. These findings demonstrate that the pathway of water uptake into maize seeds is influenced by a complex of differing physical (pericarp) and chemical (seed part composition) factors. To better understand how maize seeds are hydrated and how hydration may affect germination in the field, new techniques are needed to study the movement of water in seeds.
In the past, several methods have been used to quantify water uptake by seeds. The most common method is to weigh seeds in time course studies under controlled environmental conditions. This technique is quantitative, simple to perform, and nondestructive; however, the pattern of water uptake is not revealed. The pattern of water uptake has been studied by imbibing seeds in water containing stains or labels. A limitation for using staining or labeling techniques is that seeds must be sectioned, a destructive procedure, to view the stain or to quantify the label (McDonald, Sullivan, and Lauer, 1994). Stains or dyes may not move with the water front and be restricted by the seed coverings (Beresniewicz, et al., 1995a, b). Collectively, methods are needed that are nondestructive to allow repeated measurements and quantify water directly to provide unbiased measurements of water uptake. Nuclear Magnetic Resonance (NMR) and Magnetic Resonance Imaging (MRI) have been used extensively by plant biologists, and both methods could potentially be used to non-destructively localize and quantify water as well as metabolites and oils in plants and seeds (Pfeffer and Gerasimowicz, 1989; Pope et al., 1993).
Seed germination - Seed germination is a highly dynamic stage of the plant life cycle, with many genetic and metabolic processes undergoing transition from a developmental/storage mode to a germinative/mobilization mode (Bewley and Black, 1994). The myriad of simultaneous physiological processes occurring in germinating seeds makes it difficult to study events strictly related to the initiation of germination per se. If the entire seed is sampled, the catabolic pathways in the storage tissues can confound measurement and interpretation of the anabolic and growth processes in the embryonic axis. This problem is exacerbated by the variation that exists among individual seeds in their rates of progress toward completion of germination.
Population-based models are valuable tools in analyzing and interpreting variation in populations of germinating seeds (Bradford, 1995, 1996). However, since these models depend upon germination or other essentially destructive criteria (enzyme assays, mRNA abundance, etc.), they are inevitably statistical and post facto with respect to the behavior of an individual seed. It is generally not possible to assay a given seed for its biochemical or molecular activities and also know when or whether that seed would have germinated if it had not been sampled. This problem has been identified by a number of authors as being a critical limitation to progress in understanding the physiological and molecular basis of germination (Bradford, 1996).
A solution to this dilemma would be to develop nondestructive reporters that could signal the metabolic status of a seed prior to visible germination. It would then be possible to identify whether expression of a given gene is invariably linked to subsequent germination or dormancy of the seed. An ideal assay should permit repeated, nondestructive sampling of the same individual seeds over a time course of normal germination or during various physiological and developmental transitions (e.g., dormancy alleviation, hormone or light titration, osmotic stress, etc.). Such a reporter system would reveal the temporal variation in germinative metabolism and permit the staging of seeds at similar points in the cascade of events leading to radicle protrusion. If pooled samples are required, the reporter signal could be used to group seeds into specific expression categories. Alternatively, population-based approaches could be used to analyze the distributions of reporter expression with respect to germination patterns (Bradford and Trewavas, 1994).
As reporter genes are controlled by specific promoters, is there evidence of variation in gene expression within seed populations? Wide variation is evident in GA sensitivity of individual GA-deficient (gib-1) mutant tomato seeds with respect to induction of germination (Ni and Bradford, 1993). Both wild type and mutant seed populations exhibited at least a 1000-fold range of endo-b-mannanase activity among individual seeds prior to radicle protrusion (Still and Bradford, 1997). Using the tissue printing technique (Cassab and Varner, 1989; McClure and Guilfoyle, 1989), expression of several genes was found to vary over a wide range among individual seeds (Mella et al., 1997). Pooled samples of extracted mRNA show strong GA-induced expression on a northern blot, but this obscures the range of expression among seeds that may underlie the variation in germination behavior. To establish a causal relationship between expression of a given gene and germination, we need to know both expression level and subsequent germination timing for individual seeds. The variation in germination percentage and rate, especially when the seeds are under environmentally imposed stress, should be reflected in the physiological and molecular events occurring in individual seeds. Since gene expression and enzyme activities vary widely among seeds, experiments must be designed accordingly, using single-seed approaches whenever possible.
Responses of seeds to environmental stress - Several environmental stresses, including chilling stress, have been associated with an increased production of reactive oxygen species (Jennings, 1994; Prasad et al., 1994a, b; Smirnoff, 1995) and oxygen radical scavenging enzymes (Zhang and Kirkham, 1994). These toxic compounds may accumulate to a level exceeding the capacity of oxygen radical enzyme scavenging systems (i.e. catalase, peroxidases, superoxide dismutase), thus setting the stage for stress induced damage to cellular proteins, DNA, and lipids.
Some of the more common symptoms of chilling injury listed in order of their response time are: cellular changes in membrane structure and composition; cessation of protoplasmic streaming; plasmolysis and increased cellular leakage; altered metabolism with increased production of carbon dioxide, ethylene, and products of anaerobic respiration; reduced plant growth; surface lesions, pitting, sunken areas, and discoloration; water-soaking of the tissue indicating cellular breakdown and loss of membrane integrity.
The character and severity of chilling symptoms is a function of the species, the variety, the plant tissue and its maturity, as well as the severity and duration of the exposure. For example, cucumber seeds imbibed at 2.50C are resistant to chilling injury, but become increasingly sensitive during the early stages of germination (Jennings and Saltveit, 1994a). Seeds imbibed for 16 hours could withstand 144 hours at 2.50C, whereas survival of seeds imbibed at 32 hours was greatly reduced after only 72 hours of chilling.
Both temperature and chemical shock treatments may induce tolerance to biological and environmental stresses. For example, heat shock and ethanol treatments are capable of inducing chilling tolerance of cucumber seedling roots (Jennings and Saltveit, 1994b). Additional knowledge about the causes of chilling injury, will lead to the development of genotypes and treatments that will reduce the susceptibility of seeds and seedlings to chilling injury.
Seed dormancy - Physiological seed dormancy (dormancy not due to physical restrictions preventing water uptake) is a state of suspended animation that prevents normal germination under conditions usually favorable for plant growth. Although dormancy has been widely investigated (Simpson, 1990; Hilhorst, 1995; Khan, 1997; Li and Foley, 1997), knowledge about underlying mechanisms is lacking. This lack of fundamental knowledge is largely because traditional physiological, biochemical and molecular approaches confound germination and dormancy-breaking processes (Bradford, 1996; Cohn, 1996a).
Genetic approaches related to the question of what physiological factors affect germinability offer an alternative to conventional approaches. Individual loci controlling a quantitative trait like dormancy are referred to as QTL. Some QTL in wheat and barley appear to mark orthologous loci. Orthologous loci are genes in different species derived from a common ancestral gene. Coincidence of genetic map positions in several grass species would indicate that some loci underlying germinability have been conserved during periods of evolutionary divergence and domestication (Bennetzen and Freeling, 1997). This convergent domestication of cereal grain crops (Paterson et al., 1995) can be exploited to investigate the genetics of dormancy in one grass species like wild oat, and clone dormancy QTL from another more tractable species like rice. Much more information exists on seed dormancy in wild oat than for any other species; thus, wild oat is the best system for investigating the genetics and fundamentals of dormancy.
Wheat with red seed coat color generally has greater dormancy and/or sprouting resistance than wheat with white seed coats (McEwan, 1976). These observations have lead to the hypotheses that the R (red) genes have pleiotropic effects on coat color and dormancy (Gale, 1989) or that the R genes are closely linked to dormancy genes (Belderock, 1976). The actual situation may be some combination of the two hypotheses. Red-seeded wheat without post-harvest dormancy (Mares and Ellison, 1990) and white-seeded wheat with dormancy (DePauw and McCaig, 1983; Bhatt et al., 1983) provide support for the linked gene hypothesis. Dormancy in white wheat (Mares, 1993) is conferred by recessive genes similar to the situation in barley (Hordeum vulgare) (Burass and Skinnes, 1984). On the other hand, dormancy in red wheat exhibits maternal effects associated with red color (Freed et al., 1976) suggesting that the red pigment (phlobaphenes) in the seed coat contributes to the expression of dormancy in wheat. Little is known about the function of the R genes in wheat. In maize, the P1 gene regulates phlobaphene biosynthesis in the pericarp and the soft floral tissues of the cob by controlling expression of at least three genes required for phlobaphene biosynthesis (Grotewold et al., 1994). The function of P1 is not known, however, it is thought to have an effect on post-harvest dormancy in maize. If the R gene encodes a DNA binding protein similar to P1, it is conceivable that not only could it have pleiotropic effects on several traits including pericarp color and dormancy, but also different alleles could result in differential expression of the genes controlling these traits. For example, some P1 alleles permit phlobaphene development in the cob or the pericarp but not in both (Peterson, 1990). The function of the R gene will be further investigated to see whether it is involved in wheat seed dormancy.
Other genes are believed to be involved in the induction of dormancy. An antioxidant gene has been identified that is upregulated when dormant seeds are imbibed. (Goldmark et al., 1992; Walker-Simmons and Goldmark, 1996; Stacy et al., 1996). This gene has sequence homology to a novel group of thiol-requiring antioxidants, called peroxiredoxins. The peroxiredoxin gene has been shown to be upregulated in hydrated seeds of wheat, barley, and wild grasses. However, more work is needed to determine the role of peroxiredoxins in the induction of dormancy.
Since metabolic and genomic control have been implicated in physiological dormancy, elucidation of the mechanisms involved will require collaboration between physiologists and molecular biologists. Although work is continuing to determine how chemical treatments are able to break dormancy, no experimentally verified hypothesis completely accounts for the mechanism of action of any dormancy-breaking treatment (Bewley and Black, 1982; Cohn, 1987; Cohn, 1989). However, using chemical structure vs. physiological activity analysis, results with dormancy-breaking monocarboxylic acids and their derivatives (reviewed in Cohn 1997) suggested that tissue acidification should play an important role in the rapid activation process (Cohn, 1996a, b) leading to germination. This hypothesis has been confirmed via analysis of embryo tissue homogenates (Footitt and Cohn, 1992). Non-acid dormancy-breaking compounds can be metabolized to weak acids (Footitt et al., 1995), and this metabolism is absolutely required to activate seed germination (Lin and Cohn, 1997). Acidification may then initiate a signal transduction chain involving calcium and subsequent modification of gene expression (Felle, 1989). Cytosolic and/or vacuolar acidification may be a general prerequisite for germination and growth (Irving et al., 1992; Gehring et al., 1994; Swanson and Jones, 1996). Indeed, a vacuolar H+-ATPase is expressed early in tomato seed germination and is regulated by gibberellin, a potent dormancy breaking hormone (Yang et al., 1996).
A compound that shows promise for breaking dormancy is a common food additive called liquid smoke (Baldwin et al., 1994). Natural smoke contains nitrogen dioxide, a substance already known to break dormancy of red rice grains (Cohn and Castle, 1984; Keeley and Fotheringham, 1997). Doherty and Cohn (unpublished, 1997) have shown that commercial liquid smoke in highly dilute aqueous solutions will break dormancy of dehulled red rice and penetrate the hull of intact dispersal units, as well. More research is needed to determine whether natural and liquid smoke break dormancy by the same mechanism.
Effects of abscisic acid (ABA) on seeds - Current evidence indicates that protein phosphorylation/dephosphorylation processes are part of ABA-mediated events in seed development and germination. ABA-responsive protein kinases and ABA-insensitive mutants that are apparently defective in protein phosphatases have been identified. An important role for protein phosphorylation is expected because of the ease of reversibility and the demonstrated ability of protein phosphorylation to respond to internal and external stimuli (Verhey and Walker-Simmons, 1997). An ABA-responsive protein kinase (PKABA1) mRNA has been identified in wheat embryos (Anderberg and Walker-Simmons, 1992). Expression of the message is highly sensitive to ABA. As ABA levels rise in desiccating wheat seeds, PKABA1 mRNA accumulates. Upon imbibition, PKABA1 mRNA levels are maintained in dormant seeds, but decline in germinating seeds. PKABA1 mRNA can be upregulated in germinating seedlings by dehydration or cold (Holappa and Walker-Simmons, 1995). Protein kinases that are gibberellic acid (GA) responsive have been identified in germinating seeds of Avena sativa (Huttley and Philips, 1995). Many Arabidopsis mutants exhibit reduced dormancy due to lesions in ABA synthesis or sensitivity (Koornneef and Karssen, 1994). Mutants exhibiting increased intensity of dormancy have only been recently identified (Cutler et al., 1996, Albert et al., 1997). Further evidence of the importance of phosphorylation processes in seeds has been shown with an ABA-insensitive mutants, abi. The abi mutant exhibits reduced dormancy levels. The abi locus has been cloned (Leung et al. 1994; Meyer et al., 1994), and sequence analysis revealed sequence homology to protein phosphatase 2C from rat and yeast.
The plant hormone abscisic acid (ABA) is also involved in the acquisition of dormancy. Reduction of ABA by inhibiting ABA synthesis or by mutation reduces dormancy (reviewed by Hilhorst, 1995). Dormant seed embryos are more responsive to ABA as a germination inhibitor compared to nondormant embryos. Structural analysis indicates that the inhibitory effects of ABA on seed germination are caused by the 7'-methyl group, while the other ABA ring residues are less critical (Walker-Simmons et al., 1994). Alteration of the 8'-methyl group causes ABA to be metabolized more slowly than unmodified ABA. (+)-8'-methylene ABA is metabolized slowly and is even more effective than ABA as an inhibitor of cress seed and wheat embryo germination (Abrams et al., 1997). Abscisic acid metabolites do have some activity as germination inhibitors and are effective in upregulating some, but not all ABA-responsive genes (Walker-Simmons et al., 1997).
The ABA-responsive, heat stable proteins of wheat embryos have been shown to have unusual hydration properties (Walters et al., 1997). All the protein kinases, antioxidant enzymes and other ABA-responsive proteins identified in seeds offer potential controls for ABA-regulation of seed dormancy and germination and will be the object of future study.
Seed deterioration - Seed deterioration during storage is caused largely by damage to subcellular components, including DNA, that eventually lead to low vigor and loss of viability. Upon imbibition, seeds must repair this accumulated damage prior to the initiation of growth. There is considerable evidence that DNA repair must be carried out before germination can take place (Osborne, 1982; Roberts, 1988). Following DNA repair, DNA replication is initiated in the embryonic meristem, often coincident with or shortly after the initiation of radicle growth (Dasgupta et al., 1982). The cycle of cell division progresses from the G1, or quiescent phase, into the DNA synthesis (S) phase, the second resting phase (G2), and the mitotic or cell division phase (M). The entire genome of a cell is replicated during the S phase, increasing the normal 2C chromosome number to 4C, or four copies of each chromosome.
When plant cells undergo a transition from proliferation to quiescence, such as in seed maturation, the cell cycle arrests in either G1 or G2 with 2C or 4C chromosome complements, respectively. Although there are exceptions, the seeds of most species arrest cell division in the G1 phase of the cell cycle and thus contain the 2C chromosome complement in the majority of cells of the embryo axis at maturity (Deltour, 1985; Bino et al., 1993). The physiological significance of this is uncertain, but there is evidence that cells arrested in G2 are more sensitive to stresses such as radiation and free radicals than are cells arrested in G1 (Deltour, 1985). The cell cycle resumes upon imbibition in preparation for germination. During priming in tomato and pepper seeds, DNA synthesis is initiated and the percentage of 4C nuclei in meristematic tissues increases (Bino et al., 1992; Lanteri et al., 1993, 1994). Thus, when primed seeds of those species are redried for storage, their embryonic meristems have high percentages of 4C nuclei. Primed seeds also often exhibit reduced longevity in storage (Kraak and Weges, 1989; Tarquis and Bradford, 1992; Bradford et al., 1993). It has been hypothesized that the change in DNA status may render primed seeds more susceptible to deterioration during storage (Bino et al., 1992; Lanteri et al., 1993, 1994; Saracco, et al. 1995; Liu et al., 1996). Other studies, however, have shown that the loss in seed longevity occurs early in the priming process, while cell cycle advancement is a later event (Baker, 1995). In addition, germination advancement during priming can occur regardless of whether embryonic meristem cells exhibit cell cycle activity (Cheng, et al., 1997). Nonetheless, initiation of cell cycle activity is evidently an important event in the development of a normal seedling following germination, and impairment of cell cycle capacity may be an early consequence of seed deterioration.
Biochemical markers may provide insight into the aging process. These markers may be helpful for assessing seed quality and aging. Browning reactions may provide such a biochemical marker, and indicate aging at the whole seed level. Priestley (1986), cited earlier work on the browning of wheat grain termed sick wheat and suggested that the Maillard reactions may be involved with the loss of germination. The role of Maillard products was studied in soybean by Wettlaufer and Leopold (1991). Maillard products, as measured by fluorescence, increased only with accelerated aging treatment and were accompanied by a loss in germination. Sun and Leopold (1995) also found that Maillard products accumulated in soybean axes and cotyledons after aging at 360C and 75% RH. The increase in Maillard products was correlated with the loss of seed germinability; however, the correlation between seed viability and the accumulation of Maillard products was less obvious under the long-term storage conditions with low temperatures. The accumulation of Maillard products were studied in relation to seed viability in several small-seeded vegetable crops (Baker and Bradford, 1994). No consistent relationship was found between the fluorescence emission and the seed germination for either rapid or naturally aged seeds, and greater differences were measured between species than between aging treatments within species. Baker and Bradford did not rule out the possibility that Maillard reactions were involved in seed deterioration; however, they questioned the use of the fluorescence assay to detect the Maillard product accumulation in seeds. One problem may be due to the methodology employed, as many seeds contain fluorescent compounds that may confound measurements (Taylor, 1997). Partially purified protein extracts have been shown to reduce variation in fluorescent readings (Sun and Leopold, 1995).
Cellular mechanisms of desiccation tolerance and of the longevity of dried tissues have converged in recent years on a unifying hypothesis involving oligosaccharides and their ability to preserve membrane integrity and influence the formation of biophysical glassy states (Horbowicz and Obendorf, 1994; Leopold et al., 1994; Sun and Leopold, 1994; Sun et al., 1994; Bernal-Lugo and Leopold, 1995). Sucrose, raffinose, stachyose, or verbascose are commonly found in desiccation tolerant seeds (Amuti and Pollard, 1977). Loss of desiccation tolerance during germination or priming may be associated with a reduction in oligosaccharide content (Williams and Leopold, 1995). The re-induction of desiccation tolerance in germinated seeds was associated with the accumulation of sucrose (Bruggink and van der Toorn, 1995). Changes in soluble sugar composition in seeds might be important in longevity as well (Koster and Leopold, 1988; Chen and Burris, 1990; Horbowicz and Obendorf, 1994). Accumulation of disaccharides and oligosaccharides aid in desiccation tolerance by preserving membrane and protein integrity during dehydration (Crowe et al., 1992). An attractive hypothesis is that sugars are critical for maintaining membrane integrity during desiccation (i.e., the water-replacement hypothesis; Clegg et al., 1982) while also fostering the formation of glassy states that retard deteriorative reactions (Leopold et al., 1994). Work by Bernal-Lugo and Leopold (1995) identified two glass transition peaks; one associated with sucrose, and a second stronger signal due to raffinose. The magnitude of the raffinose signal was greater in cultivars which had better storability. Because the oligosaccharide composition and content varies, it is likely that different oligosaccharides will have the ability to amplify the glass signal. Most research seems to suggest that longevity in seeds is due to the stabilizing effect of oligosaccharides (Bernal-Lugo and Leopold, 1992; Ooms et al., 1993; Lin and Huang, 1994), especially in their effect on glass formation (Ooms et al., 1993; Sun and Leopold, 1993; Hoekstra et al., 1994). It has also been reported that longevity (P50 >10 year) in a variety of orthodox seeds is associated with sucrose to oligosaccharide ratios of < 1.0 (Horbowicz and Obendorf, 1994). The molecular and biochemical regulation of the synthesis, accumulation, and catabolism of oligosaccharides in seeds will continue to be a fruitful area to explore for potential mechanisms to enhance seed longevity and quality.
The physiological mechanism responsible for the rapid loss of peanut (Arachis hypogaea L.) seed quality during storage is unknown. Changes in esterase activity of unimbibed and imbibed peanut seeds previously exposed to ambient storage conditions were correlated with a decline in seed quality (Aung and McDonald, 1995). Total specific esterase activity of unimbibed seeds declined from 100% to 91% to 84% in the cotyledon and 100% to 92% to 87% in the axes of high, medium, and low quality seeds during storage, respectively. After 3 days imbibition, total specific esterase activity for the low quality seed cotyledons and axes was 94% of the high quality seeds. Seven isoesterase isoenzymes increased and four decreased in the cotyledons, and two isoenzymes increased in the axes following imbibition. These changes were not associated with damaged isoesterase species found in unimbibed seeds following seed storage. Therefore, specific isoesterases are prone to deterioration during aging of peanut seeds, and other isoesterases associated with germination are preferentially synthesized in deteriorated peanut seeds during imbibition.
Production, Assessment, Enhancement, and Preservation of Seed Quality:
Environmentally sound seed production practices to improve seed yield and quality - Little is known about the role of root and rhizomes in the persistence and long-term productivity of cool-season grass seed production fields. Increased understanding of root and rhizome systems may be key to enhancing the economic and environmental sustainability of grass seed production enterprises. The above ground growth of grass plants may be strongly influenced by hormonal signals that originate in the root system (Passioura, 1988; Saab and Sharp, 1989; Davies and Zhang, 1991; Meinzer et al., 1991). In other words, roots sense suboptimum conditions present in the soil and send a signal to tillers and leaves to modify growth and developmental behavior accordingly. These signals may also affect flowering and seed yield of grass plants. Tiller (shoot) growth and development is affected by field burning in Kentucky bluegrass (Chastain et al., 1997) and in creeping red fescue (Meints et al., 1996), and by drought stress in perennial ryegrass (Velloza et al., 1996). These observed responses by tillers may in fact, originate in the root system or at least have significant effects on the root system. In some cases, management factors that cause differences in seed yield are not heralded by changes in tillering. Seed yield responses to suboptimal soil pH, mineral nutrition, stand age, and population density may be initiated in the roots or their strongest effects may be felt by roots.
Increased numbers of rhizomes in both Kentucky bluegrass and creeping red fescue have been linked to reduced flowering and seed yields. Ethylene increased the rhizome:root ratio in creeping red fescue, and consequently, flowering was reduced (Meints et al., 1996). Increased early spring nitrogen greatly increased rhizome production in Kentucky bluegrass and reduced seed yield (Chilcote and Ching, 1973). Seed yield was greatest when N was applied in the late spring which minimized rhizome production.
Root production increased when Kentucky bluegrass plants were defoliated. However, defoliation decreased root growth in creeping red fescue and Chewings fescue and increased rhizome weight (Ensign and Weiser, 1975). It is not known whether root growth and longevity are affected by rhizome production, nor is it clear whether the two underground components interact. Parsons and Robson (1981) found that in perennial ryegrass, partitioning of assimilates to roots declined in the fall, remained low in the winter, and increased during early spring. However, assimilate partitioning to roots decreased with internode elongation and flowering in late spring and summer. Sugiyama (1995) showed that root biomass was inversely related to the rate of tillering among tall fescue genotypes because plants that produce few large tillers rather than many small tillers had more carbon available for root growth.
The indeterminate growth habit of peanuts (Arachis hypogaea L.) presents numerous challenges to seed producers. Spears and Sullivan (1995) found that germination and vigor of peanut seed is related to genetics, the stage of development at harvest, and environmental conditions during kernel development. In years when weather conditions adversely affected peanut seed growth and development, immature seeds exhibited low germination. Seed vigor, as measured by accelerated aging and conductivity, increased significantly as seeds matured and was very sensitive to the environmental conditions that occurred during development and maturation. Further studies in North Carolina have shown that seed size and maturity interact to influence germination and vigor. Seeds of the same size but different levels of maturity, differ in quality. The level of maturity at harvest has a substantial influence on germinability and vigor. Seed conditioning practices can be modified to remove lighter, immature seeds to improve quality.
The stage of development at the time of muskmelon seed harvest also impacted quality. Muskmelon (cv. Top Mark) seeds from eight stages of development were harvested and analyzed after 6 years of storage for viability and vigor using controlled deterioration, germination at reduced water potential, and four-day-root lengths as indices of vigor. Immature 30 and 35 days after anthesis (DAA) seeds lost viability rapidly during long-term storage under favorable conditions (3.3 to 5.7% MC, 100C) (Oluoch and Welbaum, 1996). Top Mark seeds harvested after edible maturity but before severe deterioration (50, 55, and 60 DAA) showed the greatest resistance to controlled deterioration, were the most tolerant of reduced water potential during germination, and produced the greatest four day root lengths (Oluoch and Welbaum, 1996).
Qualitative and quantitative evaluation of seed physical characteristics - Computer and other advanced technologies offer hope for improving seed quality in the future. For example, Misra and Shyy (1995) have developed an instrument for nondestructive evaluation of soybean seed quality. Both computer imaging and ultrasound technology have been combined in this instrument (Misra et al., 1993). The instrument was also found to detect asymptomatic diseased soybean seeds. Panigrahi et al. (1995) developed an automatic threshholding technique for scanning and segmenting the images of ear corn from the background for characterization of corn germplasm. Shyy et al. (1996) described a method of scanning ear corn images with a flat-bed scanner and integrating the images with the descriptors in the GRIN database.
Impedance Spectroscopy is a biophysical method (MacDonald, 1992) that may also have application in seed quality assessment. A system has been developed that measures electrical impedance of plant tissues with an ICR (inductance, capacitance, and resistance) meter or impedance analyzer (Zhang and Willison, 1991a). This computer-controlled analyzer can record and analyze data over a desired spectrum of frequencies (Zhang and Willison, 1991b). Individual components of the double-shell model have been proposed to represent the biological condition of the vacuole, cytoplasm, plasma membrane and extra-cellular space. Therefore, the relationship between biophysical parameters and plant cellular functionality is hypothesized. Electrical impedance readings can detect frost hardening and frost injury in leaf tissue (Repo et al., 1994). Impedance Spectroscopy has not yet been used to assess seed viability or quality.
Evaluation of genetic purity - Random amplified polymorphic DNA (RAPD) has emerged from biotechnology research as a practical genetic identity determinant useful in plant breeding, seed production, and seed testing programs. Research in the last five years described a DNA extraction procedure from dry seeds of maize (Zea mays L.), cotton (Gossypium hirsutum L.), soybean (Glycine max [L.] Merr.), wheat (Triticum aestivum L.), and red clover (Trifolium pratense L.) that could be successfully employed in subsequent RAPD amplification of DNA fragments (McDonald, Elliot, and Sweeney, 1994). Further studies with soybean seeds revealed that seed deterioration, contamination by fungi, and seed production environment did not affect the stability of RAPD markers (Zhang, McDonald, and Sweeney, 1996a). These results suggest that RAPDs can be used for genetic purity studies of soybean (Zhang, McDonald, and Sweeney 1996b). Studies with maize seed demonstrated that the RAPD markers were dominant, and hybrid seeds generally possessed the same markers for both parents. However, RAPD markers obtained from the maize seed coat and endosperm were not representative of their genetic heritage while the embryo was (McDonald, 1996). Further work has shown that RAPDs are useful in determining the genetic purity of petunia and cyclamen seed (Zhang, McDonald, and Sweeney, 1997). These results demonstrate that the use of RAPD markers is inexpensive, simple, fast, avoids the use of growing plant tissue, is applicable to a number of crops, and can be successfully used in a variety of ways to enhance and determine the genetic purity of seeds.
Evaluation of physiological quality and vigor - The accelerated aging test is an important seed vigor test for many large-seeded crops, but its value in small-seeded crops has been limited because moisture uptake is too rapid, resulting in fast seed deterioration for some species. A method was examined to retard small seed moisture uptake in an accelerated aging test (Zhang and McDonald, 1997).
High and low quality impatiens (Impatiens wallerana Hook) seed lots were exposed to three different saturated salt solutions producing differing relative humidities (KCl - 87%, NaCl - 76%, and NaBr -55% RH) at 38 and 410C. All other equipment and procedures were the same as recommended for a standard accelerated aging test. As relative humidity of the accelerated aging chamber declined, seed moisture content decreased. Seed deterioration of the small-seeded crop increased with increasing relative humidity, so that useful accelerated aging results that correlated with seed vigor after 4 days germination could be obtained with either KCl or NaCl by aging for 72 h and 96 h at 380C for KCl and NaCl, respectively, or 48 h at 410C for both salts. Saturated salt solutions modify the relative humidity of an accelerated aging test so that accelerated aging can be used to test the vigor of small-seeded crops such as impatiens.
Germination testing at cold temperatures is another common way to assess the physiological quality and vigor of seeds. Records at the Iowa State University Seed Laboratory over the past few years have indicated that cold test values for soybeans normally are about 5 to 10% lower than the warm germination tests. Samples for the 1994 crop averaged 23% lower for cold test compared to the warm test values. Other laboratories in the Mid-West have reported similar declines in cold test values. These problems were repeated in samples from 1995. Preliminary analyses indicate no obvious association between cold test germination decline and any of the common causes of germination loss, including disease, low seed moisture, frost damage, mechanical injury, or cultivar. Additional work is planned to uncover the reason for these inconsistent test results.
Variable-temperature germination response has been modeled for many crop species (Benech-Arnold et al., 1990; Bierhuizen and Wagenvroot, 1974; Covell et al., 1986; Ellis et al., 1987; Garcia-Huidobro et al., 1982a, b; Probert, 1992). Heat-sum models define germination rate as the reciprocal of the time taken for a certain percentage of the population to germinate (Garcia-Huidobro et al., 1982a). It has been observed that germination rate usually increases linearly between a base temperature and the optimal temperature for a given seed lot (Heydecker, 1977). At supra-optimal temperatures, germination rate often appears to exhibit a linear decrease.
Thermal germination response models can also predict cumulative germination response under field-variable temperature conditions but are derived from laboratory experimentation conducted at constant temperature. The predictive validity of these models is based on several assumptions that are usually made in order to simplify analysis and to facilitate comparisons among seed lots. Common assumptions include the following: subpopulations within a given seed lot share the same base temperature; germination rate response is linear over the suboptimal temperature range; germination rate response is linear over the supraoptimal temperature range; instantaneous germination rate is independent of temperature pattern; instantaneous germination rate is independent of thermal history; and subpopulations maintain their relative germination rate regardless of the thermal environment to which they are subjected (Garcia-Huidobro et al., 1982a; Covell et al., 1986; Benech-Arnold et al., 1990; Probert, 1992).
Besides temperature, other abiotic and biotic seedbed variables also affect germination response (Hegarty, 1973). Soil water availability is often the limiting factor for seedling establishment, particularly in wildland revegetation efforts in the Intermountain West (Roundy and Call, 1988). Fortunately, a similar modeling approach can be used to quantify the interactive effects of temperature and water stress on seed germination and emergence (Gummerson, 1986; Bradford, 1990). Predictive models for germination response to water stress are based upon the observation that germination rate is also linearly related to water potential for many species (Hegarty, 1976). Gummerson (1986) developed a model for estimating ""hydrotime"", the water potential equivalent to ""thermal time"" for temperature response. Previous application of the hydrotime concept has been tested primarily on agricultural species, such as, muskmelon (Welbaum and Bradford, 1991), sugar beets (Gummerson, 1986), and lettuce (Bradford, 1990). Thermal and hydrothermal-response models require additional validation under field-variable temperature and moisture conditions.
Seed health and pathological testing - With increasing international trade of seeds, there is concern over introducing and spreading diseases around the world. One disease of concern is Erwinia stewartii which is problem in the U.S. but not several important foreign markets. Research at Iowa State University has proven that there is zero risk of seed transmission of Erwinia stewartii from any seed lot with less than 10% infected seed. Naturally infected seeds comprise only 0-5% of a lot. Infection levels higher than this are extremely rare and only occur for susceptible cultivars infected well before anthesis. There is essentially no risk of introducing this pathogen by seed into another country (Block, 1996).
Seed enhancement and conditioning technology - Seed priming is a technique by which seeds are partially hydrated to a point where germination processes begin but radicle emergence does not occur (Heydecker and Coolbear, 1977; Bradford, 1986). Primed seeds usually exhibit increased germination rate, greater germination uniformity, and sometimes greater total germination percentage (Heydecker and Coolbear, 1977; Brocklehurst et al., 1984). Increased germination rate and uniformity have been attributed to metabolic repair during imbibition (Bray et al., 1989; Burgass and Powell, 1984), a buildup of germination-enhancing metabolites (Coolbear et al., 1980), osmotic adjustment (Bradford, 1986), and, for seeds that are not redried after treatment, a simple reduction in the lag time of imbibition (Bewley and Black, 1982; Brocklehurst and Dearman, 1983; Heydecker, 1977).
The following presowing hydration treatments have been used to enhance seed germination response: equilibration under conditions of high humidity (Perl and Feder, 1981; Finnerty et al., 1992), soaking in water (Bleak and Keller, 1974; A-As-Aqui and Carleto, 1978; Coolbear and McGill, 1990) or osmotic solution (Heydecker et al., 1975; Knypl and Khan, 1981), equilibration with a matric-potential control surface (Gray et al., 1990; Hardegree and Emmerich, 1992a, b), intermixture with a porous matrix (Peterson, 1976; Taylor et al., 1988; Khan et al., 1992; Beckman et al., 1993), and simple water addition to subgermination water content (Austin et al., 1969; Lush et al., 1981). The degree of enhancement with priming depends upon the temperature, water potential, duration, and other conditions specific to the treatment medium (Heydecker and Coolbear, 1977). Some long-duration, low-water potential, and/or high-temperature priming treatments can have a negative effect on subsequent germination response (Coolbear et al., 1980; Ely and Heydecker, 1981; Gray et al., 1990; Hardegree and Emmerich, 1992a, b).
Presowing seed hydration treatments are limited only to those conditions that do not result in premature radicle extension (Heydecker and Coolbear, 1977). This limitation does not greatly narrow the range of potential treatment combinations that could be tested to determine optimal priming conditions. Previous studies have estimated optimal priming conditions to occur at the least negative water potential that prevents radicle emergence (DellAquila and Tritto, 1990; Evans and Pill, 1989; Hardegree and Emmerich, 1992a; Hardegree, 1994). Hardegree (1996) determined that optimal priming conditions for some species occur at water potentials that would eventually result in root growth given a longer treatment duration.
Practical application of seed priming requires scaling up of experimental procedures to treat large quantities of seed. Bulk priming of seeds has been achieved in bubbling columns of osmotic solution (Bujalski and Nienow, 1991; Bujalski et al., 1991; Finch-Savage, 1991). Most priming treatments are thermodynamically equivalent to simple water addition to an optimal subgermination water content (Heydecker and Coolbear, 1977). Experimental-scale priming methods may be useful in determining the optimal priming conditions of temperature and water prior to bulk priming with simpler gravimetric techniques such as those described by Heydecker and Coolbear (1977) and Gray et al. (1990).
Combining biological seed treatments with chemical and physiological enhancements may further improve germination and emergence of plants under most field conditions. Biopriming (a combination of preplant seed hydration and biological seed treatment) is especially useful for crops that are susceptible to imbibitional chilling injury such as lima bean (Phaseolus lunatus L.) and sweet corn (Zea mays L.) (Bennett et al., 1992; Khan, 1992; Paine, 1993; Bennett, 1997). Biological agents may also be combined with seed during a priming process using aerated, dilute salt solutions. Tomato (Lycopersicon esculentum Mill.) seeds were bio-osmoprimed in aerated -0.8 MPa NaNO3 at 200C for 7 days with nutrient broth and Pseudomonas aureofaciens (bacterial strain AB254) added on day four (Warren, 1997). Bio-osmopriming allows rapid seed colonization by the beneficial organism(s), and more uniform coverage of the seed surface compared to other techniques (Smith, 1996; Warren, 1997). Recent reports of drum priming technology (Rowse, 1996; Warren and Bennett, 1997) suggest these systems may also allow concurrent seed hydration and application/colonization of beneficial microbes.
The failure of seeds to germinate at elevated temperatures is called thermoinhibition (Small and Gutterman, 1991). Seeds that fail to germinate due to thermoinhibition enter into a secondary dormancy called thermodormancy (Bewley and Black, 1982). When returned to lower temperatures, thermoinhibited seeds are capable of germinating while thermodormant seeds will not. Most lettuce cultivars are inhibited by soil temperatures greater than 25 to 320C (Borthwick and Robbins, 1928; Gray, 1975; Thompson et al., 1979). Thermoinhibition of lettuce is also accompanied with an increased sensitivity of germination to both reduced water potentials and high NaCl concentrations (Bradford and Somasco, 1994; Coons et al., 1990).
The temperature range at which lettuce germinates is genetically determined and can be influenced by environmental conditions while the seed is developing. Various seed priming treatments have been employed to alleviate thermoinhibition (Guedes and Cantliffe, 1980; Cantliffe, 1981). Priming does not extend the predetermined temperature range, but allows a greater fraction of the seed to germinate at temperatures which were previously inhibitory. The mechanism by which priming overcomes thermoinhibition is by the irreversible initiation of cell elongation (Cantliffe et al., 1984) and by lowering the force required to penetrate the endosperm (i.e. the embryo yield threshold, Bradford and Somasco, 1994). Priming treatments may also make primed seeds more susceptible to deterioration in storage (Tarquis and Bradford, 1992), as well as increase the incidence of abnormal seedlings when seeds are germinated at high temperatures (Guedes and Cantliffe, 1980; Cantliffe, 1981).
Seedling establishment - The success of seedling establishment has a substantial impact on final crop yield, since yield is dependent on the plant population for many crops. The main components which characterize a field seed-bed are: 1 ) temperature, 2) moisture, 3) physical characteristics of the soil, and 4) biotic factors, mainly pathogens. The response of emergence to these characteristics has been examined primarily under field conditions, where these variables were experienced, but not controlled. Generally, no differentiation was made between the effect of the various components on germination and on pre-emergent growth. In the instances where this distinction has been made (Taylor, 1977; Hegarty, 1979; Finch-Savage, 1987), retrieval studies were approximations, and the aspect of vigor was not included.
Differences in seed vigor, regardless of the crop studied, can be demonstrated in the laboratory. However, the effects of vigor, as measured in the laboratory, on field performance are much less demonstrable (Burris, 1976). A discussion of seed vigor and field performance presupposes that vigor is a tangible entity (Burris, 1976). This specific entity has yet to be identified. In a recent survey which includes ten years of data (Egli and TeKrony, 1995), a relationship was sought between germination, vigor (as expressed in accelerated aging and cold tests), and field emergence. These authors concluded that the relationship between the results of seed quality tests and field performance was directly related to seed-bed conditions. Others have also concluded that both seed quality and the seed-bed environment determined seedling emergence in the field (Wheeler and Ellis, 1992). Thus, the ability of a laboratory test to predict field performance decreased as the seed-bed conditions became more stressful. Although seed vigor tests were generally better predictors than the traditional standard germination test, when seed-bed conditions are poor, no laboratory test could adequately predict field performance. The interaction between seed quality and the seed-bed environment (soil) is complex and needs further examination.
Objectives
-
Elucidate fundamental mechanisms underlying seed development and germinability
-
Develop methods for improving seeds as genetic delivery systems
-
Define the ecological interactions of seeds with their environment
-
Methods
1. Elucidate fundamental mechanisms underlying seed development and germinability. A. Development Obendorf (NY-I) will continue to study the role of carbohydrate accumulation in seed development. This research will include assay of enzyme activities, transcription of mRNAs (cDNA blots), and translation of enzyme proteins (antibody precipitation) involved in the biosynthesis of soluble carbohydrates in developing and maturing seeds with emphasis on soybean and maize. Galactinol synthase, raffinose synthase, stachyose synthase, verbascose synthase, and galactopinitol synthase will be assayed in axis and cotyledon tissues during seed and zygotic embryo development in planta and in vitro, during precocious maturation of zygotic embryos and during maturation of somatic embryos. In preliminary experiments with immature soybean seeds, enzyme activities have been identified and assay techniques developed for in vitro biosynthesis of raffinose, stachyose, galactopinitol A and galactopinitol B. A galactinol synthase cDNA identifies soybean galactinol synthase mRNA of the appropriate size and will be used in cDNA blots to assay the expression of the soybean galactinol synthase during seed development and maturation. Bradford (CA-D) has isolated cDNA for tomato galactinol synthase and is studying expression of this gene in relation to seed development and longevity. As cDNA probes and antibodies become available (e.g. cloning of stachyose synthase has been reported), transcription and translation of each enzyme will be assayed during seed development and maturation and in selected physiological studies. When available, mutant genotypes will be used to test the relationship of soluble carbohydrates to seed desiccation tolerance and storability. Allen (UT-BYU) will take a sabbatic leave in the Obendorf lab in 1998 to study sugar transitions during drying of germinating seeds. High sugar levels present in developing and germinating maize kernels may represent a physiological or osmotic stress that reduces the vigor level of some cultivars. This stress reduces starch reserve deposition and accumulation of stress-related hormones which adversely affects germination. DeMason (CA-R) will determine whether jasmonic acid or methyl jasmonates are involved in the stress response during kernel development of sh2, su1, and se maize cultivars. Tissue culture will be used to test significant parameters affecting the stress response. Field trials will test whether applications of ethylene or jasmonic acid biosynthesis inhibitors can increase kernel weights and improve germination performance. This project is planned to complement the research of Still (AZ) who will determine how sugar concentrations in these genotypes affect germination as described below. Westgate and Boyer (1985) found that a block in embryo development in corn was correlated with low photosynthetic reserves in the maternal plant. Further studies revealed that sugar uptake was less in corn ovules isolated from dehydrated plants (Schlusser and Westgate 1991a, b). These studies indicate that the influence of water potential on seed development, maturation, and germination requires further clarification. McDonald (OH) will study dent corn to identify the water potentials at which specific enzymes and nucleic acids are deactivated/activated during seed maturation/germination. Welbaum (VA) will assist McDonald (OH) during this project by providing expertise on measuring water potential of developing seeds using psychrometry. B. Germination Arabidopsis has become a useful model system for studying germination because of the large number of mutants available (e.g., Koornneef et al., 1989; Lhon-Kloosterziel et al., 1996). Studies of A. thaliana germination are hampered by the large variability among individual seeds and the relatively high rate of after-ripening (Derkx, 1993). Tomato has, therefore, become a preferred model system for mechanistic germination studies (Hilhorst and Karssen, 1992; Black, 1996). In the past two years, Bradford (CA-D) has developed a collection of cloned germination-associated genes and germination-specific cDNA libraries for tomato. Clones are available for a number of potential cell wall hydrolyzing enzymes (pectinase, b-1,4-glucanases, b-1,3-glucanase, arabinosidase), a subunit of vacuolar H+-translocating ATPase, and potential protein-kinase related proteins (Yang et al., 1996; Yang, et al., 1997; Dahal et al., 1997; Sitrit et al., 1996). In addition, endo-b-mannanase has also recently been cloned from tomato seeds (Bewley et al., 1997). An objective of Bradford (CA-D) will be to obtain promoters for these genes to use as drivers for nondestructive reporter genes. The reporter gene of choice is firefly luciferase (LUC), as it is uniquely suited to the non-invasive reporting of regulated gene expression and is short-lived, allowing transient expression to be monitored (Koncz et al., 1990). Collaborators at the Wageningen Agricultural University, Wageningen, The Netherlands (S. van der Krol and H. Hilhorst) have transformed Moneymaker tomato (parent line of the gib-1 mutant) with luc driven by the cauliflower mosaic virus (CaMV) constitutive promoter and obtained seeds expressing the LUC protein. They have detected luminescence from individual intact seeds following application of luciferin, demonstrating the feasibility of the approach (Dr. Henk Hilhorst, personal communication). A single population of seeds will be repeatedly assayed for LUC activity and also followed to the completion of germination. Bradford (CA-D) and Walker Simmons (ARS-WA) will collaborate to investigate the role of protein-kinase related proteins cloned from germinating tomato seeds. Since germination is asynchronous among individual seeds, the nondestructive reporter will allow assessment of expression of particular genes in relation to the timing of radicle emergence. In addition, a given reporter could be used as an indicator to sort seeds into common expression categories. The utility of the reporter would be multiplied, as the reporter would be used to overcome the temporal asynchrony of individual seeds, but the seeds in a given expression category could then be assayed for expression of several additional genes via tissue printing. This could reveal coordinate or modular expression patterns among groups of genes, which is common in seed development and germination (Comai et al., 1989; Hughes and Galau, 1989). Reporters linked to promoters of genes thought to be involved in endosperm weakening, which is believed to be a key event leading to radicle emergence, could be used to select seeds for puncture force measurements to determine whether gene expression is consistently followed by weakening. Endosperm weakening is also a key event preceeding radicle emergence in muskmelon seed germination (Welbaum et al., 1995). It is likely that endosperm weakening is a likely control point for germination in other seeds with similar anatomy. Muskmelon seeds will be used as a model system to study enzyme-induced endosperm weakening in advance of germination. Welbaum (VA) and Bradford (CA-D), jointly funded through a BARD grant with collaborators in Israel, will construct an expression library in E. coli from mRNA collected from germinating muskmelon (Cucumis melo L.) seeds. The expression library will be used to identify and characterize cell wall hydrolases that degrade micropylar endosperm tissue prior to muskmelon seed germination. Bradford (CA-D) will concentrate on identifying genes associate with endosperm weaking, while Welbaum (VA) will do associated work to characterize the proteins involved with this process. Taylor (NY-Geneva) and Stanwood (CO-NSSL), will use video imaging equipment to study seed germination. Taylor (NY-G) will observe water uptake fronts in the near infared (NIR) range from 960 to 980 nm (Gaffey et al., 1993) to show water movement during Phase I imbibition using spectral specific video imaging equipment in both reflectance and transmittance modes. CCD camera technology is sensitive to NIR radiation and, using selected interference filters and/or illuminating sources such as IR emitting LEDs, band widths will be narrowed to view specific water absorption bands. A dedicated computer with a frame grabber will be used to capture and store images. Appropriate software will then facilitate further enhancements and analysis of these images. Stanwood (CO-NSSL), is developing an automated video imaging system to analyze seed germination. Stanwood (CO-NSSL) and Taylor (NY-G) will share technical expertise on video image analysis. Using fluorescent dye and volumetric analysis, Still (AZ) will document the rate and pathway of water uptake into su, se-9, and sh-2 sweet corn to describe how differing sugar concentrations influence the rate and pattern of water uptake. This work will complement the research of DeMason (CA-R) who will describe how sugar concentrations in these genotypes affect development as described above. Together these studies will provide valuable information about the cause of poor seed vigor usually associated with these sweet corn genotypes. Still (AZ) will also characterize the water relations of raw, pelleted, and primed lettuce seed germination by imbibition in distilled water or polyethylene glycol solutions of 0.3 or 0.5 MPa from 10 to 320C to assess the effects of these treatments on germination performance. Murphy (AR) will screen a large number of genetic lines of spinach (Spinacia oleracea) for differences in sensitivity to high temperature thermoinhibition of seed germination. Lines representing extremes of the response will be analyzed for mechanisms contributing to sensitivity or resistance to thermoinhibition. Aspects to be studied include physical and chemical characteristics of the pericarp, differences in respiration and carbohydrate metabolism, differences in heat shock proteins, and differential display analysis of gene expression at permissive and non-permissive temperatures. Chilling injury often reduces stands of warm-season crops planted during the spring in the U.S. Jennings (KS) will investigate the role of oxygen free radicals and the systems involved in radical scavenging processes as they are related to chilling induced injury symptoms in cucumber and corn seedling roots shortly after germination. Preliminary work has shown that both natural and synthetic radical scavengers are capable of ameliorating the chilling stress response. Radical scavenging enzyme systems to be studied include catalase, ascorbate peroxidase, glutathione reductase, and superoxide dismutase. Comparisons will be made between chilling sensitive and tolerant cultivars when available and effects of treatments (heat and ethanol shocks, ABA) which induce chilling tolerance. C. Dormancy Cohn (LA) will study the interaction of liquid smoke and environmental/physiological variables such as depth of dormancy, temperature, light, etc. using bioassays and other established procedures (Footitt and Cohn, 1992). Dehulled red rice grains also will be pulsed with a concentration range of selected aliphatic and aromatic alkenols in aqueous solutions using the standard laboratory protocol (e.g. Footitt and Cohn, 1995). From germination response curves, the concentration of each chemical that elicits 50% germination will be obtained via graphical analysis. Values will be utilized in QSAR analysis (Cohn, 1997). As an offshoot of a project examining the inheritance of herbicide resistance in rice (collaboration with Drs. J. Oard, LSU Dept. of Agronomy, and S. Linscombe, LSU Agricultural Center), a standard array of F1, F2, and backcrosses between Bengal rice and native strawhulled, awnless red rice (biotype Sonnier) will be assayed for dormancy immediately after hand-harvesting and through a post-harvest afterripening period of up to 8 weeks at 300C. In collaboration between Cohn (LA) and D.R. Gossett (Dept. Biology, LSU-Shreveport), reduced and oxidized free glutathione as well as glutathione conjugates will be determined (Gossett et al., 1996) after red rice is dry-afterripened at 300C for up to 8 weeks. Enzymes characteristic of oxidative stress will be determined as time permits. Arabidopsis mutant seed stocks will be increased and appropriate crosses made to ascertain mutant purity. Dormancy will be characterized using a variety of the germination stimulants previously developed (Cohn, 1989, 1997). [Cohn (LA) in collaboration with Dr. J. Larkin (Dept. Biol. Sci., LSU)]. Knapp (IA) and Cohn (LA) will collaborate to test the ability of germination stimulators and other chemicals to break dormancy in eastern gamagrass. Gamagrass is promising forage crop for the U.S., but complex seed dormancy has prevented the crop from reaching its potential. Welbaum (VA) and Cohn (LA) will conduct a collaborative project to determine effective ways to break dormancy in switchgrass and ramps (Allium tricoccum). Both switchgrass and ramps have economic potential as crop plants if reliable ways can be found to break dormancy and achieve consistent germination. Foley (IN) will complement the work of Cohn (LA) with dormancy breaking compounds by conducting a genetic analysis of dormancy in wild oats. Foley and colleagues have identified quantitative trait loci (QTL) molecular markers for dormancy. The use of QTL has revealed a genotype by environmental interaction for dormancy in wild oat (Fennimore, 1997). Future research will investigate grass genome co-linearity. Comparative mapping will be used to identify the chromosomal position of dormancy QTL and to determine whether molecular markers corresponding to dormancy QTL in other species detect orthologous dormancy QTL in wild oat. In addition, new markers and/or markers more tightly linked to dormancy QTL in wild oat will be identified using bulked segregant analysis (Michelmore et al., 1991) and AFLP procedures (Vos et al., 1996). Tightly linked molecular markers and a recombinant inbred line population will be used to investigate gene action and the genotype by environmental interaction related to germination temperature. The molecular markers obtained will further our understanding of the genetic and environmental basis of seed germinability. Foley (IN) will also determine positioning loci for future cloning of dormancy genes from rice in collaboration with Cohn (LA). Studies of the red seed coat color gene (R) in wheat by Warner (WA) will determine whether: the R gene is linked to a gene conferring dormancy, or the R gene is pleiotropic causing red seed coat color and dormancy. White-seeded mutants induced and selected from Chinese Spring wheat, a single gene red genotype (R1R1r2r2r3r3), will be characterized by Warner (WA) for depth of dormancy, after-ripening properties, and sensitivity to ABA, and will be compared with conventional white-seeded genotypes for inheritance of dormancy and seed color. Walker-Simmons (ARS-WA) will collaborate with Warner (WA) to study changes in sensitivity to ABA during afterripening. Use of single gene white mutants will eliminate some of the difficulties in distinguishing between tight linkage and pleiotropic gene action. The projects of Warner (WA) and Foley (IN) are designed to add complementary information about the genetic nature of dormancy in grain crops. Walker-Simmons (WA-ARS) will use molecular biology to characterize genes expressed in hydrated dormant seeds that remain growth-arrested. Regulatory genes in the ABA signal transduction pathway will be targeted for study. Specific objectives include: Identify proteins phosphorylated in response to ABA and in hydrated dormant seeds. Express and characterize PKABA1 kinase activity and identify interacting proteins using the yeast two-hybrid system. Obtain a genomic clone for PKABA1 and characterize other members of the PKABA1 protein kinase subfamily. In collaboration with Walker-Simmons (WA-ARS), Walters (CO-NSSL) will determine whether protein kinases, antioxidant enzymes, and other ABA-responsive proteins identified in seeds offer potential controls for ABA-regulation of seed dormancy and germination. Bradford (CA-D) has also obtained a tomato cDNA corresponding to a protein-kinase related protein from yeast whose expression is correlated with dormancy and germination inhibition (Yang et al., 1997). The expression of this gene is also stimulated by ABA. Bradford (CA-D) and Walker-Simmons (WA-ARS) have exchanged cDNAs and have tested for interaction between the respective proteins using the yeast two hybrid system. Bradford (CA-D) will use complementation of yeast mutants to determine whether the tomato protein is a functional homolog of the yeast protein, which is a key sugar sensor and regulator of sugar metabolism. With cooperation from the National Seed Storage Laboratory (CO-NSSL), Foster (MT) will collect and evaluate lines of beard;ess wildrye (Leymus triticoides) for seed dormancy. The hydrothermal time model has recently been shown to be applicable to describe the loss of dormancy and changes in germination behavior during afterripening (Bauer et al., 1997). Bradford (CA-D) will use the hydrothermal time approach to assess changes in dormancy and vigor of true potato seed. Use of botanical seed for potato propagation is becoming widespread in many developing countries, but seed dormancy and low vigor are obstacles to adoption. Methods to assess and uniformly break seed dormancy without loss of vigor will be investigated using the hydrothermal time model. D. Deterioration Obendorf (NY-I) Bradford (CA-D), and Allen (UT-BYU) will jointly test the hypothesis that oligosaccharide content (or oligosaccharide/disaccharide ratio) is important in formation of the glassy state and therefore in seed longevity. In particular, changes in soluble sugars during imbibition and priming will be determined and related to effects of these pretreatments on subsequent seed longevity in storage. One key enzyme in the synthetic pathway of oligosaccharides, galactinol synthase, has been cloned from tomato by Bradford (CA-D) and will be shared with the other W-168 collaborators. Attempts will be made to manipulate gene expression and seed oligosaccharide content to test their role in seed longevity. Bradford (CA-D) will continue to investigate the relationship between cell cycle activity and storage longevity. Mechanisms regulating entry into the cell cycle could be a target of deteriorative processes, reducing the storage life of seeds and compromising the ability of the seedlings to develop and grow. Bradford (CA-D) will also apply molecular biology techniques to monitor changes in DNA/RNA with seed aging and determine whether the proposed degradation of such molecules during aging is subsequently repaired during imbibition and/or priming. Walters (CO-NSSL) and Roos (CO-NSSL) will collaboratively investigate effects of hydration/dehydration on seed deterioration. This project will emphasize how hydration cycles affect aging processes and desiccation tolerance thresholds. Cantliffe (FL), in cooperation with research at the University of California (CA-D) and the USDA-ARS Experiment Station at Salinas, CA, will determine the metabolic block(s) of lettuce seeds imbibed at high temperatures in order to obtain physiological markers for derviation of thermotolerant lettuce breeding lines. The storage life of primed seed is generally reduced compared to nonprimed seed (Perera and Cantliffe, 1994). To test the potential storage life of lettuce seeds, treated (pelleted or pelleted and primed) and untreated seeds will be subjected to controlled deterioration at 400C over a saturated salt solution for various aging periods by Still (AZ). Germination of aged seeds will be tested at 200C to determine whether pelleting and priming treatments have an aging effect on lettuce seeds. 2. Develop Methods for Improving Seeds as Genetic Delivery Systems A. Production i) Crop management Spears (NC) in collaboration with TeKrony and Egli (KY) will investigate the influence of adverse temperature during seed development in the field on soybean germination and vigor. Spears (NC) will also study the effects of peanut vigor, kernel size, and maturity on field performance and yield. Chastain (OR) will investigate development of crop seed yields in burned vs. nonburned stands. Rhizome-forming red fescue (cv. Shademaster) will be compared with a cultivar that produces few rhizomes (Seabreeze) and Chewings fescue cultivars (SR5100 and Jamestown II), which produce no rhizomes. Three stubble treatments (i) no stubble removal, (ii) complete mechanical removal of stubble, and (iii) removal of stubble by burning will be compared. Above ground plant weight, weight of rhizomes, and weight of roots will be determined during late fall, early spring prior to stem elongation, and near peak flowering in late spring. Roots and rhizomes will be excavated, washed, and separated into roots, rhizomes, and tillers to determine the distribution and abundance of root and rhizome systems, root:shoot and root:rhizome ratios, and to compare burned and non-burned plants. Chastain (OR) will also investigate the impact of stand density and age on root development of bunch-type and creeping-type grass seed crops. Perennial ryegrass, tall fescue, and creeping red fescue will be sown at row spacings of 15, 30, 45, and 60 cm without changing within-row seeding rates. Root and rhizome production will be monitored over a four-year period. The coordination of plant water uptake and crop rooting responses will be determined by time domain reflectometry (TDR) probes placed in plots to measure the impact of soil water on root production and distribution. Seasonal/temporal changes in root systems will be determined by following root formation and development throughout the year. Flowering and seed yield will be measured to determine optimal spacing for grass seed production. ii) Seed quality Still (AZ) will harvest rapeseed (Brassica napus) and broccoli Brassica oleracea L. group Italica) seeds at various stages of maturity near Yuma, AZ. Seed fresh weight, dry weight, moisture content, color, and embryo or endosperm development will be investigated as practical phenotypic markers for specific physiological events during seed development. Overall, seed quality and potential longevity will be determined by controlled deterioration in a water bath at 400C for various times. Time to 50% germination (t50) and mean time to loss of viability (p50) will be calculated from probit-transformed germination time courses to help determine the optimal stage of development for highest seed quality. iii) Seed pathology and pest management McGee (IA) will test a predictive model developed for aflatoxin contamination of corn. This model predicts that an epidemic will occur if extreme hot and dry conditions prevail in June and July in Iowa. Under these conditions, the size of the soilborne population of Aspergillus flavus is predicted to greatly increase as abundant spores of the pathogen are released into the air to infect corn inflorescences causing an epidemic if hot dry conditions persist. B. Assessment i) Physical techniques to evaluate seed quality Misra (IA) will develop a germplasm cataloging system for maize that will be tested and refined for management of the germplasm accessions at the Plant Introduction Station (Ames, IA). The final system will be shared with all of W-168 members, the National Seed Storage Laboratory, plant breeders, and germplasm curators for integrating seed images with the descriptor database. An instrument that combines ultrasound and computer imaging technology to nondestructively predict seed germination will be tested by Misra (IA). Modifications will be made in the sensor configuration and software as needed to obtain the highest possible correlation between the ultrasound analysis parameters and germination. The capability of ultrasound systems to detect asymptomatic diseased seeds has been demonstrated by detecting soybean seeds with reduced germination potential resulting from asymptomatic infection by the storage fungi (Aspergillus and Penicillium spp.). Further work is needed on the application of the technology to particular types of pathogens and seeds. This project is complementary to the projects of Taylor (NY-G) and Stanwood (CO-NSSL), who are using video imaging technology to study water uptake and seed germination. All three groups will share information on system design and capabilities. In a related project, Taylor (NY-G) will develop a non-destructive, computer-based method for testing large-seeded legumes. Individual seeds will be probed through the seed coats in either cotyledon by pin electrodes custom designed and constructed at Geneva. In-line probing fixtures will consist of four stainless steel pins equally spaced and 2 mm apart. Four pin fixtures provide three pairs of electrodes at increasing inter-electrode distances and will allow three series of impedance measurements which will be computer analyzed to remove electrode impedance artifacts. The ring electrode is a similar electrode configuration which has been used with success (Zhang et al., 1993) and could be implemented if the experimental system described above does not work satisfactorily. The potential exists for smaller pin electrode fixtures to be fabricated that could be used on small-seeded species as well. An impedance analyzer (HP 4192A) will sweep a frequency range from 10 Hz to 10 MHz providing the stimulus and measuring responses for each pre-set frequency point. The analyzer control, data storage, and analysis will be handled by a Power Mac computer. This system provides a unique nondistructive tool to study viability and cellular function at the whole seed level. This work will complement related computer seed analysis projects projects by Stanwood (CO-NSSL) and Misra (IA). Foster (MT) will collect data on pure seed, multiple floret-pure seed, and insert matter weights for thickspike/streambank wheatgrass (Elymus lanceoplatus) and Newhy wheatgrass (Elymus hoffmanii) and develop multiple unit factors in order to improve analysis efficiency and uniformity of these seeds. This work will be performed in cooperation with the Utah, Colorado, and Nebraska seed laboratories. For flower crops, little information is available on seed anatomy/morphology. There are currently no descriptions of normal or abnormal seed characteristics for flower crops. McDonald (OH) will develop a Flower Seed Testing Handbook to help standardize flower seed testing. ii) Genetic identity and purity Molecular biology techniques have the potential to improve the accuracy and speed of genetic analysis of seeds. McDonald (OH) will continue to develop and refine polymerase chain reaction techniques for routine application in genetic purity testing of seeds. iii) Physiological quality or vigor The Pacific Northwest has long had a reputation for producing high quality seed. Recently, the Washington State University Seed Laboratory has received several seed lots of wheat that produced a high frequency of split coleoptile seedlings. Although these seed lots had excellent viability (germination test), emergence under field conditions was significantly less than produced by a comparable seed lot without split coleoptile seedlings. These observations indicate a need for tests to predict cereal seed quality and vigor. Warner (WA) will a) investigate the physiological mechanisms leading to split coleoptiles in wheat, b) identify the environmental and production practices causing split coleoptiles, and c) develop tests for predicting cereal seed quality and vigor. Foster (MT) in cooperation with the National Seed Storage Laboratory (CO-NSSL) will collect and evaluate lines of Altai wildrye (Leumus angustus) for improved seedling vigor. The accelerated aging test is a common seed vigor indicator for many large-seeded crops, but its utility for sweet corn evaluation may be limited due to the anatomical and compositional differences among su, se, and sh2 genotypes. A saturated salt accelerated aging (SSAA) test will be evaluated by Bennett (OH) in collaboration with McDonald (OH) using three sweet corn endosperm groups, plus field corn seed lots. Thermogradient table germination tests, cold test results, and field emergence will also be used to rank the seed vigor of various cultivars. Observations will also be made on microbial growth among seed lots in the various saturated salt environments (NaCl, KCl) compared to aging over 100% water. Preliminary vigor test results suggest that the saturated salt accelerated aging test may be a useful technique for small-seeded agronomic, vegetable, floral, and turf crops as well. The optimum salt, duration, and temperature of aging for these crops will be developed by Bennett (OH) and McDonald (OH). iv) Pathological quality and seed health The Iowa State Seed Center is collaborating with CAB International in England and the Danish Institute for Seed Pathology in Denmark to construct an electronic database on seed-borne diseases that will help phytosanitary authorities make consistent decisions on regulations. McGee (IA) will write or edit approximately 500 data sheets on seed borne diseases of economically important crops. These data sheets will provide information on economic importance; geographic distribution; incidence of seedborne pathogen; effect of seedborne pathogens on seed quality; transmission of pathogens by seeds; seed treatments; and seed health tests. All information is supported by appropriate citations. This database is part of an Electronic Crop Protection Compendium (CPC) produced by CAB International. Module 1 of the CPC was released on a CD-ROM disk in May 1997 and contains 80 seed borne pathogens. C. Quality preservation Bradford (CA-D) is coordinating a collaborative study among seed companies to conduct seed storage studies to develop constants for the viability equation for a number of horticultural species. The individual companies will conduct the storage and germination studies, and the data will be analyzed in collaboration with Dr. Richard Ellis. This collaborative approach will greatly expand the number of species for which viability constants are available. The constants will be reported to W-168 members for verification and further analysis. D. Enhancement of performance Hardegree (ID-ARS) and Taylor (NY-G) will work together to interface seed priming optimization techniques (Hardegree, 1996). Taylor will provide methodology for using solid-matrix and drum-priming to treat bulk quantities of seed. These procedures will provide an optimization model for the intermixture of seed, water, and solid matrix materials to achieve maximum germination advancement of bulk quantities of seed. This procedure will also provide species-level information as to the optimal conditions for priming important agricultural and wildland species. Biological seed treatments present many advantages for crop protection, but formulations and delivery systems of biologicals have not developed to the point where they can consistently compete with chemical seed treatments under all situations. Bennett (OH) and Taylor (NY-G) will collaborate on seed enhancement research (bio-osmopriming + drum priming) and on developing film coating strategies. 3. Define the ecological interactions of seeds with their environment. A. Behavior in response to the seed environment Successful ecological restoration of degraded plant communities, whether through management or direct intervention via seeding, requires an understanding of regeneration biology. This includes knowledge of the cause and effect relationships between relevant environmental variables (e.g., temperature and water potential) and seed- and seedling-level ecophysiological responses of wildland species. In semiarid ecosystems, we need to know how seeds integrate responses to field experiences over months or years in a highly fluctuating physical environment. Laboratory experiments have provided a wealth of information on the way seeds respond to temperature and water potential. Our current and future efforts will focus on using seeds and seedling responses of desert species under laboratory conditions to predict long-term phenological patterns in the field, including dormancy loss and induction, germination, emergence, and establishment. Meyer (UT-FS) and Allen (UT-BYU), with input from Bradford (CA-D), will develop population-based threshold models based on hydrothermal time, as described previously, to characterize the responses of seeds and seedlings of a sagebrush steppe ecosystem. In a collaborative project, Hardegree (ID-ARS) will develop hydrothermal germination response data for a wide number of native and introduced rangeland species. This database will be used jointly by Hardegree (ID-ARS), Meyer (UT-FS), and Allen (UT-BYU) to examine both inter and intra-specific variability in potential germination response. These efforts will determine whether a conceptual model that considers only seed, seedling zone temperature, and water potential and their effect on soil physical properties can be used to predict field responses in the context of additional abiotic and biotic factors that may vary in complex ways. Population-level threshold models with temperature and water potential as independent variables will be developed for seed dormancy loss and germination, as well as seedling radicle and shoot growth. Microclimate monitoring will be conducted in the field to provide temperature and water potential data for driving the resulting simulation models. Concurrent data on seed and seedling responses in the field will be collected from retrieval and emergence plot experiments. Field experiments will first provide data for model development and later will provide a data set for model validation by the W-168 scientists. Important Intermountain native species will be included, with the constraint that they have similar seed maturation and dispersal phenologies, but differ in their seed germination and seedling establishment phenologies. These differences will provide for contrasting tests of the modeling approach. The proposed research will provide insight into establishment processes in a semiarid ecosystem as well as a modeling approach to seed-environment interactions, with potential application over a wide range of environmental conditions. The ARS research unit in Boise, Idaho, has recently completed construction of a germination-control system that can simulate continuously variable temperature regimes from field environments and will be available for germination modeling studies. This germination system has 36 separate units that allow for triple replication of up to 12 continuously variable temperature regimes. Hardegree (ID-ARS) has also developed a matric-potential control system to control water availability in germination response studies. These tools will be used to test hydrothermal germination response models under simulated conditions of variable temperature and moisture patterns. Germinators will also be programmed to simulate field-temperature regimes to compare laboratory germination and field emergence response. Laboratory germinators can be programmed in real-time to allow for simultaneous evaluation of laboratory and field performance. These systems are available to W-168 members collaborating on this project. B. Stand establishment The process of seedling establishment with reasonable survival potential can be separated into three stages: germination, heterotrophic seedling growth (emergence), and early autotrophic seedling growth. Knapp (IA) will evaluate low temperature tolerance of maize during each of these stages. Laboratory protocols will be refined for screening maize germplasm collections for low temperature tolerance. Seed populations have been obtained from selection programs in Iowa, Nebraska, Minnesota, and Wisconsin, and germplasm collected from New Zealand, CIMMYT and Agriculture Canada. Populations will be produced at Iowa State University and screened to identify unique genes or gene groups which might be responsible for low temperature tolerance. Continued survival and spread of reintroduced native plants requires germination and establishment of naturally dispersed seeds. In some cases, as with winterfat and sagebrush, seed germination is very tolerant to low temperatures, but seedlings may be killed by subsequent frosts. Booth (WY-ARS) will define the conditions under which imbibed seeds, germinants, and seedlings of winterfat are tolerant of freezing temperatures. Welbaum (VA), Taylor (NY-G), and Booth (WY-ARS) will collaborate to measure oxygen uptake rates of sagebrush and winterfat seeds at the low temperatures to gain a greater understanding of the physiology of low temperature germination. Lethal temperatures and cooling rates will be determined for specific growth stages to aid in predicting when seedling establishment, either from natural or sown seed, will be most successful. Cohn and colleagues (LA) will employ commercially available liquid smoke products as model chemical stimuli for fire-stimulated germination of seeds. The interaction of liquid smoke and environmental/physiological variables such as depth of dormancy, temperature, light, etc., will be evaluated with established bioassays. In most native species, dormancy mechanisms synchronize germination with the optimal period for seedling establishment. In conjunction with the work of Booth (WY-ARS) and Shaw (ID-FS), Meyer (UT-FS) will study dormancy mechanisms and environmental factors that synchronize germination of native plant species important in the restoration of western shrubland ecosystems. (Meyer and Kitchen, 1992). Common garden techniques as well as controlled crossing experiments have been used to separate genetic and environmental components of seed germination variation. Between-population variation has been correlated with collection site climate variables for a number of important wide-ranging species (e.g., big sagebrush, rubber rabbitbrush, antelope bitterbrush, mountain mahogany, bottlebrush squirreltail, firecracker penstemon, lewis flax). Shaw (ID-FS) will study the reproductive biology of intermountain Juncus and Carex species to determine the influence of seed storage and germination rate on seedling establishment. Field studies will investigate the depth to the water table as a factor in seedling survival. Skunkbush sumac (Rhus trilobata Nutt.) may be particularly valuable for revegetating degraded streams where lowered water tables are likely to prevent recovery of willows. Shaw (ID-FS) in collaboration with Booth (WY-ARS) will study the seedbed ecology of natural populations of skunkbush sumac and apply the information gained to revegetation projects using this species. Effects of temperature, moisture, physical characteristics of soil, and biotic facts (mainly pathogens) on seedling emergence are fairly well-documented. However, the distinction between effects of seed-bed environments and germination and on pre-emergent growth are lacking. TeKrony (KY) and Egli (KY) will collaboratively study the seedbed environment to determine whether seeds will germinate and the resulting seedlings emerge to develop into healthy plants. Corn and soybean seed lots with high germination, but a range in seed vigor will be screened for initial seed quality using a broad range of vigor tests (AOSA, 1983). Seedbeds that reflect various controlled environmental conditions will be created in a greenhouse. The emergence rate of the seedlings will be recorded, and once emergence is completed, non-emerged seedlings and seeds will be retrieved and categorized as normal and abnormal seedlings or dead seeds. Parallel plantings of dry and pre-germinated (radicle protrusion of 2 mm) seeds will provide a comparison to determine whether germination, pre-emergent growth, or both, makes a greater contribution to emergence performance. Field experiments will investigate the relationship between seed quality and emergence using the same categories of emergent seedlings as were used in the greenhouse retrieval studies. Seed lots of various levels of quality will be hand-planted and emergence rates will be recorded. Once emergence has been completed, the seedlings will be carefully retrieved and categorized. In precision agriculture, a field is not treated as a single entity but as many variable subunits. Field studies will be continued (Bennett-OH) with sweet corn to test whether GPS/GIS data can be used with seed quality data to improve precision seedling options and plant population targets.Measurement of Progress and Results
Outputs
- The W-168 project research will address fundamental questions relating to seed development, germination, and dormancy that are important to the seed industry. Other research objectives will focus on short-term problems affecting U.S. agriculture in the areas of seed production, stand establishment, germplasm preservation, agricultural sustainability, seed ecology, seed enhancements, and seed assessment. One area of emphasis during the next five years of the project will be real time collaboration between W-168 members and seed scientists and technologists around the world through the Seed Biology Listserver SEED-BIOLOGY-L maintained by Obendorf (NY-I) and the Seed Expertise Database maintained by Stanwood (CO-NSSL). These electronic communication tools were created to allow the rapid exchange of ideas and research results among W-168 members, seed biologists around the world, and between W-168 members and the seed industry. Communications are archived at the world wide web site http://wwwscas.cit.cornell.edu/seedbio/seedbio.html. This site archives certain information passed through the listserver. Some of the information posted on this web site includes: postings of available positions in seed biology and technology, discussions on an international seed society, upcoming meetings and events in seed biology and technology, and discussion of research results and problems. All W-168 members are subscribed to this listserver. The listserver currently has a total of 399 subscribers representing approximately 45 different countries. During the next five years, we intend to increase the collaborative use of and information available through SEED-BIOLOGY-L. In addition, Obendorf (NY-I) and Stanwood (CO-NSSL) will develop a home page web site on the NSSL server for W-168 to promote additional exchange of information on research activities, project reports/productivity, and participants. W-168 members cooperatively planned, organized, and conducted the Symposium on Seed Biology and Technology: Applications and Advances, August 13-15, 1997, at the National Seed Storage Laboratory in Fort Collins, Colorado. This highly successful meeting was attended by over 200 people representing more than 20 countries. The Symposium featured 21 oral presentations on topics such as seed development, production, enhancement, dormancy, quality assessment, germination, deterioration, and preservation by W-168 members and other leaders in seed research from around the world. The Symposium proceedings, including 4 review articles collaboratively written by W-168 members, will be published in a special June 1998 edition of Seed Science Research. Driven by the success of last years event, W-168 plans to organize and host a 2nd Symposium on Seed Biology during the next 5 years of the project.
Outcomes or Projected Impacts
- Other specific research objectives for the project include: 1. Identification and characterization of genes and gene products that are critical in the control of germination and dormancy, so they can be manipulated to improve stand establishment. 2. Development of improved methods for assessing and upgrading seed physical, genetic, physiological, and pathological quality to enhance the performance of seeds as a vehicle to deliver improved crop varieties, including bioengineered germplasm. 3. Use of predictive models will improve seed production, seed use efficiency, and seedling establishment. 4. Implement crop management strategies for improving the economic and environmental sustainability of seed production enterprises. 5. Apply biochemical and biophysical principles to extend the longevity of stored seeds to preserve the genetic diversity of species important to US agriculture and native plant communities. 6. Improve establishment of desirable species and inhibit invasion by noxious weeds on lands disturbed by mining, fire, grazing, and other ecological perturbations by understanding the influence of abiotic factors on seed germination behavior. The W-168 project is somewhat unique among regional projects because rather than collaborate on a single project, many collaborative projects are conducted by subgroups of W-168 members within the overall objectives. This is inevitable, given the diversity of species and problems being addressed, but is also desirable, as information gained from a specific collaboration is shared within the group and can be applied to other species or problems. This has occurred in the past in the seed testing, seed enhancement, and germination modeling areas, and is now occurring in the biochemical, molecular, and ecological fields. At least 19 specific collaborations are identified in the current project proposal as follows: 1. Allen (UT-BYU), Bradford (CA-D), and Obendorf (NY-I) oligosaccharides and seed quality 2. Bradford (CA-D) and Welbaum (VA) joint BARD project on cucurbit seed quality 3. Shaw (ID-FS) and Booth (WY-ARS) seedbed ecology 4. Bennett (OH) and Taylor (NY-G) seed enhancement research 5. TeKrony (KY) and Egli (KY) stand establishment 6. Welbaum (VA) Taylor (NY-G) and Booth (WY-ARS) winterfat seed respiration 7. Foley (IN) and Cohn (LA) rice seed dormancy 8. Hardegree (ID-ARS) and Taylor (NY-G) priming 9. Spears (NC), TeKrony (KY), and Egli (KY) effects of high temperatures on soybean seed development 10. Meyer (UT-FS) and Allen (UT-BYU) population-based modeling 11. Cohn (LA) and Welbaum (VA) dormancy breaking 12. Walker-Simmons (WA-ARS) and Walters (CO-NSSL) seed protein hydration properties 13. Walker-Simmons (WA-ARS) and Warner (WA) seed dormancy research 14. Roos (CO-NSSL) and Walters (CO-NSSL) seed deterioration 15. McDonald (OH) and Welbaum (VA) water potential of developing seeds 16. Knapp (IA) and Cohn (LA) dormancy in gamagrass 17. Walker-Simmons (WA-ARS) and Bradford (CA-D) protein kinase activity 18. McDonald (OH) and Bennett (OH) accelerated aging with salt solutions 19. Obendorf (NY-I) and Stanwood (CO-NSSL) seed biology web site development These extensive collaborations were estimated and are maintained through the organizational structure of the W-168 project. Through W-168, a critical mass of researchers in seed biology has been coalesced to address scientific issues critical to agriculture and environmental quality in the U.S. The increasing importance of seeds in the delivery of improved agronomic traits, including disease, insect, and pest resistance as well as nutritional quality and environmental restoration, makes the maintenance of this collaborative research expertise of critical importance.
Milestones
(0):0Projected Participation
View Appendix E: ParticipationOutreach Plan
Organization/Governance
The Technical Committee is responsible for planning and implementing the approved regional project. The Technical Committee will consist of one voting member appointed by the Director of each participating experiment station. USDA research agencies represented by contributing projects under the proposed objectives will be voting members. An administrative advisor will be appointed by the Western Regional Association of Directors. USDA-CSRS, Washington, DC, will assign a representative to assist the administrative advisor, both of whom will be non-voting members.
Committee meetings will be held annually. A secretary will be elected at each annual meeting. The officers will annually be promoted in the following sequence: secretary to vice-chairperson, vice-chairperson to chairperson, chairperson to past-chairperson. These four officers will then constitute the executive committee to provide leadership and continuity and/or immediate action.
In addition, the chairperson, in consultation with the administrative advisor, notifies the Technical Committee members of the time and place of meetings, prepares the agenda, and presides at the annual meeting. The vice-chairperson is responsible for final preparation and submission of the annual report of the regional project to the administrative advisor, who will approve and forward it to USDA-CSRS, Technical Committee members, and selected individuals. The secretary records the minutes and performs other duties assigned by the Technical Committee or the administrative advisor. Subcommittees may be named by the chairperson as needed for specific assignments.