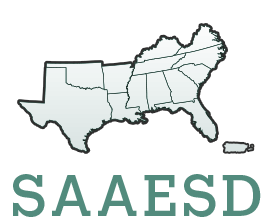
S1075: The Science and Engineering for a Biobased Industry and Economy
(Multistate Research Project)
Status: Active
S1075: The Science and Engineering for a Biobased Industry and Economy
Duration: 10/01/2023 to 09/30/2028
Administrative Advisor(s):
NIFA Reps:
Non-Technical Summary
Statement of Issues and Justification
A. The Land Grant University System, Resource Limitation, and the Impending Biological Revolution
The United Nations predicts that by 2050 agricultural production will need to increase by 70% to feed a population of 10 billion. However, in the upcoming decade, agriculture and forest systems are going to be plagued with opposing and intersecting demands: climatic threats, population growth and migration, increasing food and energy pressures, decreasing water and land availabilities, soil degradation, supply chain disruption, and rising energy, labor, and chemical costs as well as degrading natural habitats and ecosystem services. The breadth of these intersecting problems is so vast that constructive solutions can only be developed and implemented through collaborations that cross traditional disciplinary boundaries. Replacing existing petroleum-based energy and products with those that are stemming from biomass will require research and development with a broad scope.
Specifically, the objectives of this project are to address research that are aligned with current administration priorities, such as but not limited to, climate change, artificial intelligence, resilient infrastructure, carbon-smart agriculture, and sustainable aviation fuels. From a human capital perspective, renewable energy and by-product production systems usually intersect with those of biomass production such that the ensuing processing facilities are typically located in agrarian areas, promoting rural economic development. To transition from fossil-fuel-based-systems to economically-viable- biomass-based systems fundamental and applied research is urgently required. Without these substantial research efforts, the technical capacity to switch from a fossil fuel-based economy to a diversified bioresource-based economy will be severely limited by unanswered questions, undeveloped technologies, suboptimal bioresource production and utilization, and missed opportunities to thwart climate change.
Multistate linkages are needed to address the breadth of the needed research. The research framework proposed herein is designed to address these limitations with the expertise of professional scientists and engineers primarily associated with the Land Grant University (LGU) system, which partners with important policy-setting agencies such as the United States Departments of Agriculture (USDA), Energy (US DOE) and Defense (US DOD), as well as the National Science Foundation (NSF) and various state agencies to meet its research mission. Additionally, the recent unprecedented National Resources Conservations Service’s Partnerships for Climate-Smart Commodities investments will require development of knowledge and ensuing practices for the successful implementation of its findings. Thus, there will be a pressing need for the science and engineering that will be developed in this Multistate project, such that society can seamlessly transition to consuming sustainable and biobased commodities and enjoying renewable energy sources.
B. How S-1075 enabled new renewable energy industries.
Increasing the breadth of economically viable renewable energy and by-product production implies the generation of power and second-generation liquid biofuels, as well as bioderived chemicals that can be petroleum additions or even replacements. Such efforts lead to a more circular and carbon neutral bioeconomy. Researchers in the S-1075 Multistate project are advancing this goal through research into most facets of production systems for bioenergy and associated co-products, as detailed below.
B.1. Feedstock
Efficient feedstock supply chains are needed at commercial scale to enable the successful deployment of biobased systems. Progress was made in securing an abundant, economical supply of biomass feedstock delivered with predictable specifications that meet conversion needs. Biological feedstocks from areas representative of the various LGU partners were evaluated for biomass yield, composition, and other characteristics. S-1075 researchers at MN, MA, MT, ND, , NY, OH, PA, KY, TN, WA, and WI have tested a range of biomass feedstocks included switchgrass, wood chips and residues, giant miscanthus, energy cane, camelina, and sweet sorghum with an emphasis on improving feedstock yield and convertibility to fuels and products, lowing the fertilizer and energy input and better understanding logistics requirements [1-6].
Sustainable supply of a biobased feedstock requires efforts to not only improve the yield and convertibility of a biomass crop but also reduce the production costs. The boundary of feedstock logistics is being extended to incorporate fertilizer and energy costs as well as operations like harvesting, baling, infield aggregation, storage, hauling, value addition (densification), and so on. The year-round supply of commercial quantities of uniform-consistency biomass depends on the specific commercial deployment of biorefineries. Hence, the integration of supply and conversion should be as seamless as possible. Such a complex system demands a systematic understanding of feedstock type and characteristics, availability and costs, pre-conversion processes, and logistics systems.
Although, great strides were made in terms of predicting biomass crop yields and quality on selected areas, knowledge gaps still remain in: 1) expected yields of these energy crops throughout US available land; 2) spatial and temporal variations in yield and supply due to climate and soil conditions including development of new sensors and algorithms; 3) harvesting and handling of these low bulk density materials; 4) developing new tools and models that identify target physical properties important at the interface of processing and conversion; and 5) evaluating the sustainability and climate-smartness of the biomass feedstock production and transportation system.
B.2 Conversion
In the past years, S-1075 participants worked on researching and developing technically feasible, economically viable and environmentally sustainable technologies to convert biomass resources into chemicals, energy, and materials, setting-up the foundations for future biorefineries. Developing co-products from biomass feedstocks became a focus area because biomass derived non-fuel co-products can meet the needs of renewable chemicals and materials traditionally made out of petroleum. Such efforts include valuable products from lipids and residuals from lipid processing, lignin and other cellulosic components.
Thermochemical conversion (TCC) technologies, such as co-firing/co-combustion, direct combustion, gasification, pyrolysis, torrefaction, and hydrothermal liquefaction (HTL), play critical roles to convert various biomass feedstocks to bioenergy, biofuels, biomaterials, and/or bio-products. These technologies have been proven technically feasible, however, there are significant needs for solutions to effectively tackle the challenges (such as low conversion efficiency, cost intensive, greenhouse gas (GHG) emission, and potential environmental impacts) to improve conversion efficiency and processing costs, while reducing carbon footprint in the circular bioeconomy. Advanced data analytic tools, including machine learning/artificial intelligence, internet of things (IoT), big data, high performance computing, cloud communication and storage, as well as new technologies (e.g., plasma-assisted pyrolysis, and hydrogenation bio-oil upgrading) need to be integrated to overcome the existing challenges, and thus move forward the TCC platform. Such advances can only be achieved through a Multistate approach.
As opposed to thermochemical processing, biochemical conversion typically takes place at milder temperatures in an aqueous environment. This processing platform is based on the saccharification of the plant cell wall structural carbohydrates to produce high quality sugar streams that can be converted to biobased fuels, chemicals and products. System/synthetic biology approaches are being developed by S-1075 participants to improve the yield of high value products from biomass components. For example, Clostridium strains were engineered for fatty acid ester production from corn stover hydrolysates [7] with a butyl acetate yield of up to 32 g/L being achieved, which is the highest ever been reported from microbial production [7-9]. Bioengineered oleaginous yeast Yarrowia lipolytica facilitated conversion of lipid-based feedstock to terpene-based specialty chemicals [10, 11]. These studies help to understand the dynamic of the membrane and storage lipids and the role of lipid metabolism in development and stress response.
Lignin is the second largest renewable polymer after cellulose and is the largest source of aromatic compounds. Economically feasible biorefinery scenarios must use all the components (including lignin) from the biomass to produce a diverse mix of fuels, materials, and chemicals according to their highest value [12]. However, due to lignin’s amorphous structure and cross-linked nature, its valorization is challenging and requires additional research. S-1075 participants are actively involved in lignin valorization research using a variety of technologies. Lignin products have been converted to many products including adhesives, antioxidants and antimicrobials, 3D-printing materials, hydrogels, and resins [13-17]. Additionally, lignin derivatives have been used as a feedstock to produce liquid fuels/lipids, chemicals, and energy materials such as graphite, nanocomposite, and carbon dots [18, 19]. Challenges that exist for lignin utilization include both separation and purification from biorefinery streams. The chemistry of these streams significantly impacts isolation processes. After extraction and purification, lignin may also require additional modification to achieve the desired properties in material application.
Although great strides were made in co-product research, knowledge gaps remain related to integration of these technologies to produce second-generation production systems that are coupled to economically viable energy systems. Knowledge gaps also remain in understanding the environmental impacts of these systems, including carbon footprint. Sustainability metrics such as socioeconomic and environmental indices are needed to assess biorefinery systems [20]. Effective metrics can help to identify and quantify the sustainability attributes of biorefinery processes and products. However, methodologies and outputs need to be adapted to produce reliable and publicly understandable results. Additionally, tradeoffs between different socioeconomic and environmental indicators need to be considered. Conventional indicators describe both environmental impacts, such as GHGs, eutrophication, net energy, and fossil fuel usage, as well as socioeconomic impacts, such as social well-being, energy security, trade, profitability, and acceptability [21, 22]. This suite of indicators will need to apply to the full biorefinery supply chain including feedstock production and logistics; conversion to fuels, chemicals and materials; and end uses.
B.3 System analysis and logistics
The supply chain and conversion platforms for bio-based products exist in interconnected systems. Spatial and temporal variability is inherent in these systems and needs to be accounted for in any modeling effort [23]. For instance, spatial variability injects bias into LCA calculations when applied to the local conditions or decision-making parameters [24]. Integrated modeling frameworks characterizing variability and reducing uncertainty would provide better information to decision-makers and policy planning. Modeling frameworks also need to be sufficiently robust to account for emerging types of system attributes such as circularity and decentralization.
Modeling frameworks that capture spatial and temporal variability are especially important for decentralized biomass processing systems that employ small-scale depots to upgrade biomass to saleable commodities, e.g., pellets, briquettes, bio-oil, and biogas. Regionally distributed depots are predicted to reduce biomass transportation costs while providing income for farmers and forest landowners. Depots will also increase the tax base in rural communities, leading to resources that can be used to improve rural infrastructure, education, and health care. As investment in depots is much lower than in full scale refineries, overcoming the “chicken and egg” dilemma that has stymied bioenergy and bioproduct commercialization might be expedited by decentralization.
C. Research needs/justification for the next 5 years
Utilization of biobased resources for production of renewable energy and materials is key to enable circular bioeconomy. Biobased resources are highly variable and non-homogeneous, and climate and geographic dependent. R&D efforts are needed to develop robust technologies for low-cost and efficient harvest of different bioresources and to handle their non-homogeneity, and to achieve zero or near-zero emission conversion. Furthermore, there is a need for increased attention on bioprocesses optimized for carbon removal, or permanent removal of carbon dioxide from the atmosphere. Specifically, biofuels, biochemicals, and biomaterials that have net negative carbon footprints over their entire lifecycle. Examples include ethanol fermentation with geological storage of CO2 produced in the bioreactors, and pyrolysis with geological storage of bio-oil and soil storage of biochar.
For the biological conversation technologies, the microbial strains are the key players. Special attention should be paid to develop robust strains though the innovative metabolic engineering and synthetic biology strategies. The metabolic engineering and synthetic biology work will take advantage of the burgeoning machine learning (ML) artificial intelligence (AI), big data, new materials and novel sensors, and bioinformatics technologies. On one hand, it is necessary to develop workhorse technology that can handle low-value recalcitrant waste streams (including the emerging organic waste of environmental concerns, such as municipal solid waste and plastics), with an aim to produce high-value biochemical and bioproducts, besides biofuels. In addition, integrating the biological process with other processes, including the chemical catalytic, electrocatalytic, and photocatalytic (artificial photosynthetic) processes can greatly improve the conversion efficiency and economic feasibility.
In addition to the improvement of biofuel technology itself, product diversification is an important aspect for promoting circular economy principles. Therefore, co-product development should remain a focus of the S-1075 research community. Given the diversity of biomass feedstock and multiple fractions of biomass, researchers of complementary expertise (e.g., biological, thermochemical, and electrocatalytic) will collaborate with each other to develop multiple streams of products and co-products from biomass. Conversion of biobased resources into value added products could be, but are not limited to, food and food additives, fiber, packaging, pharmaceutical and biomedical products. However, there are several interrelated gaps for the production of these commodities to become economically feasible and these include: scaling-up to an industrially relevant production system; achieving economically competitiveness; reconfiguring bioprocessing technologies to maximize negative carbon emissions and maximizing greenhouse gas benefits; identifying the correct funding landscape for transitioning to beta scale production; and, managing the business and corporate culture related issues that are associated with new technology adoption and commercialization.
There will be a need to address the shift from a carbon-emitting petroleum-based economy to a carbon-negative bio-based economy that does not disrupt the existing global food supply chain. Mapping bioproducts to their fossil counterpart and identifying materials transition pathways will be critical. In short, it will be critical to determine pathways for economically viable biobased commodities and renewable energy production within existing or realistic production constraints. The breadth of the problem is shocking and will require an interdisciplinary approach that will draw from engineering, biology, biochemistry, chemistry, material science, soil science, agronomy, and agricultural economy.
D. Funding landscape: Challenges and Opportunities for the next five years
Biomass-derived renewable energy and bio-products is finding renewed interest, especially because of the urgent need to mitigate climate change, minimizing greenhouse gas emissions and increasing atmospheric carbon capture. However, there is a sweet spot where climate mitigation practices and techniques need to intersect with economic feasibility, finding this coveted space, where these compromises can occur, requires research efforts. Additionally, there is interest in fitting all aspects of biomass-derived, manufacturing, distributing, and discarding of energy and bio-products within the confines of the circular economy. A variety of program funding continue to be available through AFRI FAS (USDA NIFA), Sustainable Agricultural Systems (USDA), Sun Grant Initiative (USDA), NSF, as well other targeted initiatives, such as the Sustainable Aviation Fuel initiative and the Bipartisan Infrastructure Law. Participants of the Science and Economy Multi-State project see this funding landscape as an important mechanism for maintaining connection, continuity, and versatility in meeting research demands in a dynamic funding environment.
Related, Current and Previous Work
This proposed Multi-State Project builds on the accomplishments of previous Multi-State Projects S-1007 and S-1041. The significant accomplishments resulting from participation in S-1075 include:
1) Despite the pandemic, in the past 4 years (2018-2022), researchers associated with S-1075 generate, on average, over 100 peer reviewed articles and over 150 conference presentations annually. They have also supervised over 90 MS theses or PhD dissertations, published over 10 book chapters and books. Many book or journal publications result from collaboration between experiment stations represented in S-1075. The full list of the publications is accessible at NIMSS website.
2) Collaborative funded projects developed among S-1075 members. List here are a few examples:
Shi, J., Y. Zheng, M. Mba-Wright et al. 2019-2023. Sulfur Profiling in Pine Residues and Its Impact on Thermochemical Conversion. US Department of Energy. $1,661,922. (KY, KS, IA collaborations)
Lansing, S., F. Yu, Z.W. Wang, I. et al. 2021-2025. Systematic Characterization of Variability in MSW Streams to Identify Critical Material Attributes for Fuel Production. US Department of Energy. $ 3,411,838. (VA, MS collaborations)
Sharda V., Wilkins, M.R., et al. 2022-2026. RII Track-2 FEC: BioWRAP: Bioplastics with Regenerative Agricultural Properties. National Science Foundation. $5,999,428. (KS, NE, SD collaborations)
Li, Y., Wang, D., Huang, H, 2021-2024. Value-Added Production of Antioxidants from Sorghum Protein to Enhance Economic Viability and Sustainability of Sorghum Fuel Ethanol, USDA Foundational and Applied Science Program, $500,000 (VA and KS collaboration).
With the pandemic now behind us, renewed collaborative efforts will be sought.
3) Consultations with industry and presentations at bioenergy conferences and professional society meetings. Also, industry representatives presented at several S-1075 annual meetings and networked with members at these meetings. It is anticipated that the proposed Multi-State Project will generate similar activity.
Objectives
-
Develop deployable biobased feedstock and supply knowledge, processes, tools and logistics systems that sustainably, timely and sufficiently deliver feedstock to meet efficient handling, storage and conversion process specifications
Comments: Objective A of the 2018-2023 project was dedicated to developing deployable biomass feedstock and supply knowledge, processes and logistics systems that economically deliver timely and sufficient quantities of biomass with predictable specifications to meet efficient handling, storage and conversion process requirements. This objective remains mostly unchanged in the 2023-2028 project but extended to meet the climate-smart farming, profitability and sustainability, climate change, and productivity matrix as identified in the research needs. -
Research and develop sustainable technologies to convert biobased resources into bioenergy, biological materials, and bioproducts, including co-products, to enable circular bioeconomy
Comments: Objective B of the 2018-2023 project was dedicated to research and develop technically feasible, economically viable and environmentally sustainable technologies to convert biomass resources into chemicals, energy, and materials in a biorefinery. This objective includes developing co-products to enable greater commercialization potential. In the 2023-2028 project, this objective remains dedicated to this topic, but has an increased focus on the new synthetic biology platforms and hybrid conversion technologies with an end goal of promoting a circular bioeconomy. -
Use advanced models to provide system level analytics to enable sustainable feedstock supply and conversion technologies
Comments: Objective C of the 2018-2023 project utilized system analysis to provide economically, socially, and environmentally sustainable technologies for a bio-based economy. In the 2023-2028 project, this objective is similar, but with a greater emphasis on system level analytics, including the use of AI and multidomain modeling tools, and the development of quantitative sustainability and circularity metrics and tools to guide research and policy decisions. Work force development and outreach are integral parts of the project. The S-1075 research community will therefore focus exclusively on research-based objectives.
Methods
This project is building on the research and findings of the previous cycles of the S-1075 project (2018 – 2023), and the following Objectives and Tasks are based on these findings. This Multi-state project proposes the following set of tasks that relate to each of the three objectives (Note: Two-letter state abbreviations identify the agricultural experiment stations that will conduct various tasks):
Objective A: Develop deployable biobased feedstock and supply knowledge, processes, tools and logistics systems that sustainably, timely and sufficiently deliver feedstock to meet efficient handling, storage and conversion process specifications.
Feedstock is characterized by high moisture content, low bulk density, and variable seasonal yields. Feedstock production is dependent on geographical location, specifically climate and soil conditions. Soil health being a salient issue, identification of feedstock crops that require minimal fertilizer and energy inputs must be established. Feedstock will be handled in a different manner than existing agronomy and forestry equipment; thus, newly yet-to-be designed handling systems need to be planned. As feedstock supply chain logistics are a major barrier, novel methodology for freighting must be developed through a multidisciplinary and multistate approach. The potential use of waste products as feedstock will be examined. Overall, this objective leads to production of technology and data for designing scalable supply systems to provide abundant quantities of cost-competitive feedstock.
Task 1: Feedstock in selected geographic (AL, CA, HI, KY, MN, MT, ND, NY, OH, PA).
Oilseed feedstock, critical for sustainable aviation fuels and for co-products will be examined in MT. Studies will focus on sustainability of oilseed feedstock production and supply through genetic and agronomic tools to identify camelina genotypes that can produce higher yield and oil concentration with less nitrogen input and higher use efficiency. The potential of growing energy crops on marginal and un-reclaimed mine land will be examined (NY, OH). Supply chain of low-grade forestry feedstock, such as that obtained from trees affected by insect disease, windstorms, and drought, will be investigated (PA). In addition to oilseed, energy crops and forestry feedstock, the use of purified wood pulp, modified and unmodified starches derived from potato and corn, and chitosan derived from chitin contained in shellfish will be evaluated to produce bioproducts (PA), while IL will develop new bioenergy crops that will produce vegetative lipids in leaves and stems for energy cane, sorghum, and miscanthus. Development of technologies and processes to characterize the physical, flow, and chemical properties of biomass including municipal solid waste will be examined (AL). ND will work on the development of remote sensing applications for forage prediction using machine learning and user-friendly software tools to support these applications. For the next five years, CA will conduct research on the circular economy of organic waste within the context of Senate Bill 1383 where the state has committed to diverting 75% of organic waste from landfills by 2025. This will include research efforts on treating urban food waste with anaerobic digestion to produce renewable energy and utilizing the digestate for improved crop cultivation within urban and peri-urban areas. Included in organic waste will be stillage from spirits distilleries, controlled environment agriculture clean-out material (KY) as well as agricultural residues, waste plastics and animal manures (HI and MN).
Task 2: Characterize and use feedstock (CA, HI, MN, PA, TN, WI)
Feedstock composition determination of agricultural residues, animal manures, and macro/micro algae (HI and MN), modified polysaccharides (PA), and post-consumer urban food waste CA) will be carried out. In terms of handling, mechanical properties, such as bulk flow performance of milled biomass perennial energy crops (PA), effect of size reduction on material flowability (TN), consequences of bulk format on moisture content (TN), and flowability of food waste into anerobic digestors (CA) will be determined. The impact of biomass variability on inhibition of downstream microbial conversion will be investigated (WI). Data will inform the design of machinery and protocols for the handling of these feedstocks (TN), including the development of a mechanical tester that can evaluate mechanical interactions between milled biomass particles (PA). In terms of coproducts, modified polysaccharide will be evaluated for their viscosity, degree of substitution of modified end-groups, and surface charges for effects on physical properties (PA). Mechanical and chemical properties of composites made from these modified polysaccharides will be examined for their solubility, mechanical stability, resistance to aqueous and oily media, resistance to salt, porosity, and swellability (PA). The use of remote and hyperspectral sensing, and machine learning techniques to understand biomass composition will continue to be adapted for yield determination and ensuing logistical planning (WI).
Task 3: Transport and store feedstock (AL, CA, KS, MA, ND, OH, PA, TN)
Field harvesting models for warm season perennial grasses will be developed to estimate harvesting costs and to plan for optimal harvesting protocols (PA), as well as evaluate a wide range of biomass supply logistics mechanisms, processes, and integrated systems to reliably supply bulk-format and bale-format biomass feedstock to biorefineries (TN). Design/modify equipment to harvest, collect and package different feedstocks as well as evaluate impact on transportation, storage and product quality will be conducted (OH). Feedstock storage, sufficient to support the needs of large-scale biorefineries, will be investigated, while the development of bioleaching techniques to produce feedstock suitable for thermochemical conversions will be designed (KS). Handling, storage and transportation of non-homogenous feedstock, including mixed woody and herbaceous feedstock as well as municipal solid waste, legacy coal and household plastics, will be examined (AL). The effect of scale and centralization/decentralization of anaerobic digestion facilities on food waste hauling requirements and related greenhouse gas emissions will be determined (CA). In addition to anaerobic digestion, hydrogen production will be examined in a thermophilic waste-to-hydrogen conversion process (MA). ND will work towards the development of circular bioeconomy initial frameworks and evaluate outcomes and tradeoffs applicable to agriculture and rangeland systems of ND, which will be extended to other regions in the future. Software tools of these initial circular bioeconomy frameworks around agricultural and rangeland operations will be developed in the five-year period.
Objective B: Research and develop technically feasible, economically viable and environmentally sustainable technologies to convert biomass resources into chemicals, energy, and materials in a biorefinery. This objective includes developing co-products to enable greater commercialization potential.
In addition to bioenergy production, such as bio-aviation fuels or other liquid biofuels, bio-hydrogen and combination of heat and power (CHP), exploration of new bio-based products and biomaterials must be continued. However, to be economically viable, and environmentally sustainable, circularity of all streams must be designed and implemented. In the cadre of this multistate project, advances in conversion technologies, development of industrial production of sustainable energy and products were made; however, re-circulating of waste streams remains challenging. Combination of emerging technologies like nanotechnology, advanced sensors, synthetic biology, data analytics, urban planning, geography, rural sociology and economics are critical for making advances for establishing the use and adoption of circularity principles. Use of inputs in a circular manner is critical to provide food and energy, while minimizing environmental degradation. This Multistate community can facilitate the integrations of specific pieces of this sustainability puzzle by establishing connections between feedstock logistics, energy and bio-product production and waste stream use.
Task 1: Develop and assess biological conversion technologies including synthetic biology (AL, CA, PA, KS, KY, MO, ND, OK, SC, SD, TN)
To handle the heterogenous and diverse biomass feedstocks, S-1075 participants in the next five years will develop bioprocesses tailored to convert various biomass materials to fuels, chemical, and co-products. IL and WI stations will work on hydrothermal and other deconstruction processes to recover oil and structural carbohydrates from new bioenergy oil crops. AL and WI stations will develop workhorse technology that can handle low-value recalcitrant waste streams (including the emerging organic waste of environmental concerns, such as plastics) to produce high-value biochemical and bioproducts, besides biofuels. To enable circular bioeconomy, CA, HI, KY, KS, MO, PA, SD, TN, VA, and WA stations will work on the conversion of byproducts from biorefinery into value-added coproducts including butanol, lactic acid, 2, 3-butanediol, lignin derived triboelectric nanogenerator for biomedical applications, biodegradable polyester polymers (i.e., polyhydroxyalkonates), hydrolytic enzymes, food additives, aldonic acids, biobased tissues for wound healing, prebiotic oligosaccharides, adsorbents to reduce water contaminants, and pharmaceuticals including antimicrobials. Approaches include optimizing the process and reactor designs or economical productions by utilizing low-cost raw biomaterials and innovative process designs, as well as expanding the breadth of protein engineering and metabolic engineering projects that can be accomplished by coupling massively parallel design, DNA assembly, and high-throughput testing, leveraging low-cost synthesis of oligonucleotide pools and also improve predictive models to accelerate organism engineering by designing genetic systems sequences with desired enzyme expression levels.
In addition, PA station will investigate methods to utilize bioproducts in various packaging applications that meet the needs of entities seeking to achieve zero waste in their venues. Research will examine acceptance by marketplace, cost differentials versus alternatives, end-of-life performance, and circularity relate to closed-loop supply chains. MO station will investigate super-intensive aquaculture systems capable of harvesting and converting microbial biomass used to maintain water quality into valuable by-products, while simultaneously reducing, or eliminating, water or waste discharge to the environment. CA and MN stations will investigate anerobic digestion (AD) systems, both at bench and pilot scale (<5 tons/day), that are fed a range of urban food waste and agricultural liquid waste. MA station will explore the role of the extracellular sheath of microorganisms in the nano-scale delivery of metabolites involved in the digestion of woody substrates for bioconversion. Further, MA station efforts will focus on thermophilic waste-to-hydrogen conversion, determining how best to optimize the process and avoid hydrogen-to-formate conversion using bioreactor parameters and HPLC and enzyme activity analyses. ND will be developing bioprocessing of abundant soymeal to biochemicals using hydrolytic enzymes and microbes in separate (step-by-step) or simultaneous (one-pot) methods. The approaches include substrate formulation, process optimization, and synthetic biology. OK station will develop biological conversion technologies for the conversion of lignocellulosic derived sugars and CO2 into biofuels and value-added chemicals. This include improve microorganisms’ capacity to co-utilize sugar and gases to make various products.
Task 2: Develop and assess thermochemical conversion technologies (AL, KY, MN, OH, OK, SD, TN, TX, WV, WI)
This task will remain vital in the next five years as participants from at least ten states will continue to work on these conversion technologies. This will be done by either developing new efficient technologies or optimizing existing technologies using wide varieties of feedstocks. Researchers in LA will perform research investigating electrically driven thermochemical conversion of dedicated energy crops and residual biomass into fuels (e.g., sustainable aviation fuels) and platform molecules for further downstream conversion into materials for biomedical, environmental, and agricultural applications. SD station will investigate cost-effective thermal chemical conversion technologies, including gasification, pyrolysis, torrefaction, and hydrothermal liquefaction (HTL), to produce green hydrogen, aviation fuels, and other valued-add bioproducts and/or biomaterials for biomedical, environmental, food, and agricultural applications. OH will evaluate hydrothermal carbonization of different high-moisture feedstocks, including organic wastes, to produce hydrochar. PA will work on optimization of catalytic hydrothermal gasification process for conversion of low-grade biomass to gaseous fuel, hydrogen. AL will investigate feedstock-process relationship with focus on biofuels and bioproducts such as fuel additives, bio-lubricants, lubricant additives, and biopesticides. Meanwhile TN will continue to develop an improved understanding of the thermochemical relations for various feedstocks and the specific thermal conversion details. MN will develop and investigate catalytic microwave assisted gasification and pyrolysis of solid wastes, including microalgae, crop residues, and waste plastics, to produce high value chemicals, materials, and energy as part of our overall approach to circular economy development. OK station will investigate gasification technologies for production bioenergy from biobased materials and wastes. VA station will work on the development of thermochemical technologies to convert biomass (e.g., energy crops) into hard carbon materials as anodes for sodium-ion and lithium-ion batteries. ND will perform thermochemical processing of soybean meals to produce fermentable liquified fractions for biochemical production.
Task 3: Develop and assess chemical, catalytic, electrocatalytic, and other conversion technologies (AL, MI, MN, PA, SD, WA).
Utilization of biobased resources for production of renewable energy and materials is key to enable circular bioeconomy. Biobased resources are highly variable and inhomogeneous. Research and Development efforts are needed to develop robust technologies for different bioresources, to handle their inhomogeneity, and to achieve zero or near-zero emission conversion. Chemical, catalytic, electrocatalytic, and other conversion technologies serve as powerful tools to broaden the product portfolio, improve process efficiency, reduce processing costs, and mitigate environmental impacts. In the next five years, PA station will develop a scalable lignin-first fractionation process based on the reverse catalytic fractionation. This research will valorize lignin and make the cost-intensive pretreatment of biomass a cost-efficient process. PA will also develop continuous catalytic fractionation technologies using catalytic transfer hydrogenolysis to isolate lignin as a suite of monomeric products and convert these monomeric products into new polymeric resins for commercial applications in agriculture and elsewhere. AL will develop advanced C1 chemistry (one-carbon molecules) through catalytic and electrocatalytic conversion technologies for carbon capture, recycling, and conversion into liquid chemical platforms. SD station will explore effective applications of various catalyst systems in thermochemical processes for catalytic conversion and upgrading of agricultural and forest wastes to sustainable biofuels, chemicals, and new materials. WA will work on new biomass pretreatment technology and manufacturing technologies to process biomass into jet fuel, carbon fiber, supercapacitor, and other bio-products. MN will develop and investigate catalytic conversion and upgrading of solid wastes to sustainable fuels, chemicals and materials and a biological approach to complete utilization of liquid wastes. MI will continue developing fast pyrolysis bio-oil stabilization through electrocatalytic hydrogenation; where this approach couples renewable electricity with the energy inherent in bio-oil to create a relatively non-reactive intermediate suitable for upgrading in existing refineries.
Task 4: Develop hybrid conversion technologies (AL, HI, OK, PA, SD)
There is a need for increased attention on bioprocesses optimized for carbon removal, or permanent removal of carbon dioxide from the atmosphere. Specifically, biofuels, biochemicals, and biomaterials that have net negative carbon footprints over their entire lifecycle. Biological process is particularly useful in selectively transforming low-value carbon sources into small molecules under mild conditions. However, biological process alone often cannot produce long-chain petroleum-equivalent chemicals efficiently. While catalytic processes are well-suited for upgrading small biogenic molecules into petroleum-like compounds. Thus, integrating biological process with catalytic processes, and other emerging technologies could leverage the strengths of both, enabling more efficient processes for biochemical production. Scale-up and commercialization of the developed technologies is much needed. Transitioning research into the marketplace requires close collaborations between LGU, National Laboratories and industrial partners. Only then can successfully commercialize the research.
S-1075 participants will investigate combined thermochemical and biological conversion technologies for converting biomass feedstocks into biofuels and biomaterials. The feedstocks that will be examined include agricultural residues and candidate energy crops (HI and PA) and waste generated in the poultry industry (AL) into target products, including advanced biofuels and biomaterials. Furthermore, SD station will investigate the combination of thermochemical conversion, nanotechnologies, and other emerging engineering approaches to produce biopolymer-based nanocomposites for applications in smart food packaging and precision agriculture to reduce environmental impacts and carbon footprint. OK station will develop hybrid thermochemical-biochemical conversion technologies for the conversion of lignocellulosic biomass, wastes materials and CO2 into biofuels and value-added chemicals.
Objective C: Use advanced models to provide system level analytics to enable sustainable feedstock supply and conversion technologies.
Development of environmentally sustainable and economically viable sources of bioenergy and bioproducts is driven by the need to reduce the environmental impact of the energy industry. For this, supply chains capable of providing ample biomass for production of advanced biofuels will be necessary. These supply chains will need to be integrated with commercial scale conversion facilities and connected to viable distribution systems. This objective includes utilization of existing system analysis technology to assist in the development of usable frameworks to facilitate the biorefinery development.
Task 1. Develop system models and data tools to assess sustainability (KY, MI, MO, NE, OK, ND, NJ, OH, PA, VA).
Agricultural, forestry, and aquatic feedstocks will be included in various system analyses. For agricultural systems, changes to land-application of nutrients by shifting from manure and/or synthetic fertilizer application to digestate application and feedstocks such as duckweed, will be investigated to observe changes in carbon and nitrogen balances (PA). This work will contribute to understanding how these novel bioenergy crop systems may alter both of these important biogeochemical flows in relation to agriculture and environmental impacts of agriculture and bioproducts. New models will be developed to predict the correlation between biomass moisture content with loss of cannabinoids during drying and storage of medicinal hemp (ND). Regarding forest feedstocks, an automatically logging/updating regional inventory of biomass/byproducts to aid industrial partners in locating and utilizing feedstock will be developed (KY); broad applicability of the model to other feedstocks will be sought. Models that incorporate variability and uncertainty associated with production of bio-based products will be developed; robust modeling frameworks that reduce or mitigate variability and uncertainty will be sought (NE).
Techno-economic analyses (TEA) and environmental life cycle assessments (LCA) will be performed for numerous bio-products and bio-processes. Projects include preliminary LCA for emerging materials, such as biocomposites and advanced adhesives. TEA and LCA are also underway and will be continued for hops and dairy products, bioenergy crops, and anaerobic digestion systems for the enhancement of ecosystem services and bioenergy production (PA). ND will optimize the process of using corn starch as an alternative to cement in fabricating non-load bearing construction materials. VA will perform TEA to assess the economic feasibility of converting biomass into value-added liquid fuels, renewable natural gas, and food-grade peptides. Renewable electricity generation systems (i.e., bioenergy carbon capture and storage) will be assessed to quantify their impact on agriculture and rural communities. The net benefits of introducing load-shifting technologies (i.e., alternative fertilizer production systems) and their implications for the electric grid's reliability will also be determined (NJ). NJ station also aims to model bioenergy carbon capture and storage technologies within a computable general equilibrium model of the US to incorporate the cost of carbon, disruptive technologies, the impact of policy, and new guidelines intended to affect the economic and behavioral aspects of agricultural production and technology adoption . OK station will develop models using state-of-the-art machine learning and AI tools to reduce uncertainty and risks in commercialization of bioenergy technologies. Tropical resources (e.g., algae, seaweed, and oleaginous crops) for bioenergy products and other valuable biomaterials will be explored (HI). Carbon negative bioenergy and bioproduct systems will be especially considered, owing to the net sequestration of atmospheric CO2 equivalents (MI). These methods are used to make informed decisions in the bioeconomy and to comply with carbon markets.
Task 2. Use system models to configure, analyze and optimize bioenergy, biological materials, and bioproduct systems (OH, MI, SD, WA, WI)
Several bioenergy and bio-product systems will be configured and optimized using the tools currently available to and developed by our participating stations. Alcohol-based fuels, sustainable aviation fuel, diesel fuel, green gasoline, torrefied solid fuels, and the use of renewable electricity will be included in analyses conducted by several states (MI, OH, WA, WI). Valuable bioproduct platforms (WA), such as nanocellulose (WI), lignin (MI, WI), bio-oil, biochar (MI), biogas (MI), and syngas, will continue to be explored. The SD station will integrate data sciences and AI in development and application of advanced system models to analyze and optimize biomass catalytic conversion and upgrading systems for producing sustainable biofuels, chemicals, and new materials from agricultural and forest wastes. System models will be optimized across multiple objective functions to configure and guide the implementation of biomass supply chains, regional biomass depots, and biorefineries that make a suite of valuable products.
Measurement of Progress and Results
Outputs
- Annual report will be submitted to NIMSS website.
- Poster presentation abstracts will be made public after each S-1075 annual meeting and the symposium. Comments: During the current project, participants assemble each year in a selected location to report on their station’s progress by: 1) individual station reports; 2) a scientific symposium where research posters are presented, 3) assemble an annual report and proceedings of poster abstracts. In the next 5 years, the team will emphasize on multi-state collaborations not only in research projects but also on proposal development and student training. At each annual meeting, participants will define each year’s most pressing knowledge gaps and identify funding opportunities towards the establishments of synergistic activities among stations in a dedicated “igniting research collaboration” section. Definable outputs for the year will be decided among the group, and could include a white paper, a collective review, or a scientific article. In the following year, a research symposium will be held to showcase the work that was done to answer the specific questions. Both S-1075 members and outside stakeholders will be invited to address the committee during the symposium. Stakeholders, economists, and social scientists interested in a particular research question will be invited to the annual meeting and scientific symposium to interact with the group. This “self-management” plan will provide an integrated technical focus to annual symposia while ensuring engagement of the wide range of experts available in the S-1075 multistate team to problems of current importance.
- A large portion of the efforts outlined in Objectives A through C are application-oriented and will be useful to develop pilot projects, demonstrations, and commercialization of biomass conversion processes or novel biobased products.
- Publications in peer reviewed journals, trade journals and popular magazines.
- Patents and patent applications.
- Presentations to economic development groups, legislative groups, and to the general public.
- Posters and oral presentations at scientific and engineering conferences.
Outcomes or Projected Impacts
- The committee will continue to serve as a resource for the Bioenergy Program for Advanced Biofuels (or similar program included in the next Farm Bill), Sustainable Aviation Fuel Grand Challenge, USDA and NSF SBIR, NSF, NIFA and DOE panels.
- Multi-state membership will contribute to identification of funding priorities and shaping policy of federal agencies through participation in working groups, advisory groups, and workshops.
- Multi-state membership will play a pivotal role in the Circular Bioeconomy Systems initiative under the American Society of Agricultural and Biological Engineers (ASABE).
- The proposed research will enable reduced dependency on foreign petroleum.
- The proposed research will encourage investment in the agricultural sector and in rural communities where the feedstocks required for biobased industry are located.
Milestones
(2024):Identify 1-2 “igniting research collaboration” projects by August 2024 prior to initiating a white/review paper.(2026):Collect inputs of all participating stations by August 2026 to write white/review papers.
(2027):Publish the white/review papers by August 2027.
Projected Participation
View Appendix E: ParticipationOutreach Plan
In the next phase, we will disseminate the results and information that the group has generated in the form of a yearly Newsletter. This Newsletter will be disseminated through Extension networks; participants being from LGUs will be able to send the Newsletter to interested Extension Specialists at their respective LGUs. This Newsletter will be essential to synthesize and disseminate the group’s progress. Tackling a yearly problem and inviting specific Industry Stakeholders, Economists, Extension Specialists and Social Scientists to present and attend the Symposium will be essential to build important leverages with industrial partners. We will seek support from NSF and/or USDA to offset student travel expenses to attend the annual symposiums.
Research results will be made available through peer-reviewed journal articles. Participants in the previous Multi-State Project S-1075 generated over 100 peer reviewed and other publications annually, either independently or in collaboration with other experiment stations represented by S-1075. It is expected that similar productivity will be seen in this Multi-State Project. Many articles can be accessed through individual participant web sites. Workshops and demonstrations will be organized by participating stations for feedstock production, harvesting, storage and conversion. In addition to the Newsletter, we plan to use social media platforms (Facebook, LinkedIn, etc.) to disseminate the research outcomes from S-1075 members.
It is expected that this Multi-State Project will continue to foster scientific collaboration for collaborative proposals. Participants will meet once per year to review progress made towards attaining Objectives A, B and C. Because of the multi-disciplinary nature of this Multi-State Project, participants also often meet in scientific conference settings, planning committees, and in scientific review panels.
Organization/Governance
The Development Committee will use a standard form of governance using a chair, vice-chair and secretary as executive officers. During annual meetings, a secretary will be elected that ascends to vice-chair, then chair. Terms are for one year. Subcommittees will be formed as needed during annual meetings.
Literature Cited
[1] G.T. Fasick, J. Liu, Lab scale studies of miscanthus mechanical conditioning and bale compression, Biosystems Engineering 200 (2020) 366-376.
[2] A.R. Womac, V.S.P. Bitra, Assessment of First-Generation Cotton Module Technology for Chopped Switchgrass, Journal of the ASABE 65 (2022) 367-377.
[3] A. Chahal, D. Ciolkosz, V. Puri, J. Liu, M. Jacobson, Factors affecting wood-bark adhesion for debarking of shrub willow, Biosystems Engineering 196 (2020) 202-209.
[4] P. Yang, X. Cai, C. Leibensperger, M. Khanna, Adoption of perennial energy crops in the US Midwest: Causal and heterogeneous determinants, Biomass and Bioenergy 155 (2021) 106275.
[5] J. Montes, F. Technow, B. Dhillon, F. Mauch, A. Melchinger, High-throughput non-destructive biomass determination during early plant development in maize under field conditions, Field Crops Research 121(2) (2011) 268-273.
[6] A.R. Womac, E.E. Byers, K.G. Thomas, B. Hillenbrand, A. Smith, V.S.P. Bitra, Modeling cotton module builder and loader to enhance biomass testing, Applied Engineering in Agriculture 37(5) (2021) 911-928.
[7] J. Feng, J. Zhang, Y. Ma, Y. Feng, S. Wang, N. Guo, H. Wang, P. Wang, P. Jiménez-Bonilla, Y. Gu, Renewable fatty acid ester production in Clostridium, Nature Communications 12(1) (2021) 1-13.
[8] Y. Wang, Z. Chen, M. Haefner, S. Guo, N. DiReda, Y. Ma, Y. Wang, C.T. Avedisian, combustion of n-butyl acetate synthesized by a new and sustainable biological process and comparisons with an ultrapure commercial n-butyl acetate produced by conventional Fischer esterification, Fuel 304 (2021) 121324.
[9] P. Jiménez-Bonilla, J. Feng, S. Wang, J. Zhang, Y. Wang, D. Blersch, L.E. de-Bashan, P. Gaillard, L. Guo, Y. Wang, Identification and investigation of autolysin genes in Clostridium saccharoperbutylacetonicum strain N1-4 for enhanced biobutanol production, Applied and Environmental Microbiology 87(7) (2021) e02442-20.
[10] A.M. Worland, J.J. Czajka, Y. Li, Y. Wang, Y.J. Tang, W.W. Su, Biosynthesis of terpene compounds using the non-model yeast Yarrowia lipolytica: grand challenges and a few perspectives, Current Opinion in Biotechnology 64 (2020) 134-140.
[11] N. Li, Z. Han, T.J. O’Donnell, R. Kurasaki, L. Kajihara, P.G. Williams, Y. Tang, W.W. Su, Production and excretion of astaxanthin by engineered Yarrowia lipolytica using plant oil as both the carbon source and the biocompatible extractant, Applied Microbiology and Biotechnology 104(16) (2020) 6977-6989.
[12] A.J. Ragauskas, G.T. Beckham, M.J. Biddy, R. Chandra, F. Chen, M.F. Davis, B.H. Davison, R.A. Dixon, P. Gilna, M. Keller, Lignin valorization: improving lignin processing in the biorefinery, Science 344(6185) (2014) 1246843.
[13] R.M. Kalinoski, W. Li, J.K. Mobley, X. Chen, S.E. Nokes, B.C. Lynn, J. Shi, Controlling bacterial contamination during fuel ethanol fermentation using thermochemically depolymerized lignin bio-oils, Green Chemistry 23(17) (2021) 6477-6489.
[14] S. Pradyawong, G. Qi, M. Zhang, X.S. Sun, D. Wang, Effect of pH and pH-shifting on adhesion performance and properties of lignin-protein adhesives, Transactions of the ASABE 64(4) (2021) 1141-1152.
[15] T.A. Adjuik, S.E. Nokes, M.D. Montross, R. Walton, O. Wendroth, Laboratory determination of the impact of incorporated alkali lignin-based hydrogel on soil hydraulic conductivity, Water 14(16) (2022) 2516.
[16] J. Sutton, Evaluation of Lignin-containing hotopolymers for use in Additive Manufacturing, (2019) Master's Thesis, University of Tennessee
[17] L.S. Ebers, A. Arya, C. Bowland, W. Glasser, S. Chmely, A. Naskar, M.P. Laborie, 3D printing of lignin: Challenges, opportunities and roads onward, Biopolymers 112(6) (2021) e23431.
[18] M.C. Vin-Nnajiofor, W. Lino, S. Debolt, Y.-T. Cheng, J. Shi, Fractionation and Upgrade of endocarp lignin to carbon-silicon nanocomposites as an anode material in lithium-ion batteries, Applied Engineering in Agriculture 38 (2022) 509-516.
[19] V. García-Negrón, S.C. Chmely, J. Ilavsky, D.J. Keffer, D.P. Harper, Development of nanocrystalline graphite from lignin sources, ACS Sustainable Chemistry & Engineering 10(5) (2022) 1786-1794.
[20] A.C. McBride, V.H. Dale, L.M. Baskaran, M.E. Downing, L.M. Eaton, R.A. Efroymson, C.T. Garten Jr, K.L. Kline, H.I. Jager, P.J. Mulholland, indicators to support environmental sustainability of bioenergy systems, Ecological Indicators 11(5) (2011) 1277-1289.
[21] B.E. Dale, J.E. Anderson, R.C. Brown, S. Csonka, V.H. Dale, G. Herwick, R.D. Jackson, N. Jordan, S. Kaffka, K.L. Kline, Take a closer look: biofuels can support environmental, economic and social goals, Environmental Science and Technology 48 (2014) 7200–7203.
[22] K.L. Kline, S. Msangi, V.H. Dale, J. Woods, G.M. Souza, P. Osseweijer, J.S. Clancy, J.A. Hilbert, F.X. Johnson, P.C. McDonnell, Reconciling food security and bioenergy, GCB-Bioenergy (2017) https://doi.org/10.1111/gcbb.12366
[23] S.J. McGrane, M. Acuto, F. Artioli, P.Y. Chen, R. Comber, J. Cottee, G. Farr‐Wharton, N. Green, A. Helfgott, S. Larcom, Scaling the nexus: Towards integrated frameworks for analysing water, energy and food, The Geographical Journal 185(4) (2019) 419-431.
[24] G. Finnveden, M.Z. Hauschild, T. Ekvall, J. Guinée, R. Heijungs, S. Hellweg, A. Koehler, D. Pennington, S. Suh, Recent developments in life cycle assessment, Journal of Environmental Management 91(1) (2009) 1-21.