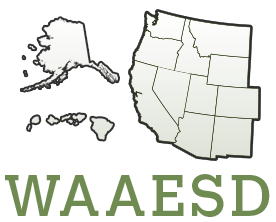
W3128: Scaling Microirrigation Technologies to Address the Global Water Challenge
(Multistate Research Project)
Status: Inactive/Terminating
W3128: Scaling Microirrigation Technologies to Address the Global Water Challenge
Duration: 10/01/2014 to 09/30/2019
Administrative Advisor(s):
NIFA Reps:
Non-Technical Summary
Statement of Issues and Justification
The water crisis and how appropriately-scaled microirrigation can address it
The world population is expected to increase to 9.6 billion people by 2050 according to a recent UN Report. The world supply of food will need to increase by 60% from the 2005 level to meet the demands of this population increase. There is a need for a sustained and diligent effort from all agricultural technology segments to meet food and freshwater demands. Irrigated agriculture provides about 40% of the worlds food supply utilizing about 25% of the land resource. Although there was a great expansion of irrigated lands in the 20th century, most experts agree that such additional great expansion in the 21st century will not be possible. The problem could be exacerbated by the largely known effects of climate change on quantity and quality of water available for irrigation and the concurrent increases in water demand to meet drinking water, energy production, and other needs. The challenge is great to the agricultural sector. The agricultural community must not only increase the food supply, but it must also conserve water and protect water quality. Additionally, it must do this at multiple scales from the largest, most technologically advanced producers to small holder units with minimal technology input. Irrigation technologies will definitely play a large role in meeting these challenges and microirrigation is a specific method of irrigation that has some inherent advantages in addressing some of these challenges. Although it has long been recognized that microirrigation has great potential for efficiently using water resources and also protecting water quality, there is only an emerging realization that microirrigation can help to address the many scale issues affecting agriculture. The scaling of microirrigation and its associated technologies include: system design, scope and costs; cropping system economics and associated risks; availability and quality of land, soil, and water resources; international and intranational guidelines and focal points; manufacturers product lines; irrigation support systems, and end-user technology skills and financial status. This proposed multistate project concerning microirrigation does not have the expertise or resources to address all of the above issues, but it can address some of the tasks.
Unless timely action is taken, it is anticipated that water supply- and water quality related crises will affect economies and resources of national and international importance. Microirrigation can reduce the waste of water to a negligible amount and reduce the transport of contaminants to surface water and groundwater. Irrigation events can be fine-tuned to spoon feed water and nutrients just in time to minimize plant water stress. It can optimize crop production (more crop per drop) and in many cases increase the quality of agricultural products.
Issues and Justification for Objective 1
The most common definition of irrigation scheduling is simply the determination of when and how much water to apply. Looking to the future, a more conservation-oriented and economically profitable definition can be stated as delaying any unnecessary irrigation with the hope that the cropping season ends before the next irrigation is needed. The crucial meaning of these two alternatives is not fundamentally different, but the complexity of perfect irrigation scheduling is best illustrated by the second definition. Many producers have uncertainty about whether their existing irrigation scheduling is correct, so they err on the side of applying extra water. This is not only an inefficient use of water, but may also cause water quality problems from runoff or leaching or may reduce crop quality. Development of more robust irrigation scheduling products that might address the various economic and technological skills of the end-users might reduce producer uncertainty and thus increase use of sound science-based irrigation scheduling. By combining more than one of the primary plant-, soil-, or ET-based irrigation scheduling methodologies, the producer might be persuaded through the mounting of evidence that the techniques are correct for sustainable crop production. Additionally, producers can easily make great strides at improving irrigation management while on that difficult journey towards that perfection of resource management. However, data are needed from a range of crops and environments to quantify the effects of different irrigation scheduling approaches on the relation of yield or product quality to applied/available water.
Issues and Justification for Objective 2
According to the 2000 survey from the International Committee on Irrigation and Drainage (ICID), microirrigation comprised less than 2% of the worlds irrigated land area. Early and growing adoption of microirrigation usually begins with comparison of microirrigation to more traditional irrigation methods for the region. Although the results of system comparisons may differ with region, crop, soil, and climate constraints, the primary goal remains to optimize production for the various irrigation methods, particularly for those methods such as microirrigation which may be less familiar to producers.
There is a gross misconception about microirrigation that it is only suitable for high value crops. Advances in the available products and increased knowledge about microirrigation maintenance have allowed the technology to be used by some end-users of all technological skills and economic levels. Additionally, it should be noted that some of the greatest land area expansion opportunities lie in increased microirrigation of the broad-based commodity crops (grains, forages and fibers). Through broader dialog between developers and end-users and improved education, new design and management schemes should help to greatly increase the use of microirrigation worldwide.
Water use through microirrigation can be approached through the concept of more crop per drop. Thus, advanced and refined management techniques will be the key in further efficiency gains in water conservation. In some cases, these advanced techniques will be economical but technologically impractical for some end-users. In those cases, it will be necessary for participants in this project to work cooperatively to develop robust science-based surrogate management strategies that are more suitable to the end-user. Fortunately, microirrigation has some advantages that can be combined to achieve this goal, such as reducing the waste of water to a negligible amount and reducing the transport of contaminants to surface water and groundwater. Irrigation events can be fine-tuned to spoon feed water and nutrients just in time to avoid plant stress.
Issues and Justification for Objective 3
Traditionally, this multistate project has been very active in technology transfer with outreach activities averaging approximately 145 per year since 2009. Although individual participants engage in technology transfer to various levels (technical skill and economic status) of end-users, there has not been a committee-wide technology transfer effort in this regard. Scientists and engineers have a responsibility to conceptualize technologies to the greatest extent possible and also to help less technology savvy end-users reach an increased knowledge and comfort with a conceptual understanding rather than an easier but less universal general understanding. The new project proposes to step up efforts in technology transfer using a broad spectrum of paper and electronic technologies as well as to propose and sponsor technical sessions at national/international conferences during the last two years of the project. If funding can be secured, the project will propose a stand-alone conference or symposium. The goals of the technical sessions/conference will be to increase end-users comfort level with microirrigation technologies and to extend information resources to a greater audience through educators and technical service providers.
Related, Current and Previous Work
Results of CRIS Search and related NIFA multistate research projects
A recent CRIS search indicated 676 records related to microirrigation (83), micro-irrigation (187), micro irrigation (187), drip irrigation (551) or trickle irrigation (38) although the ASABE has defined the preferred spelling as microirrigation (ASABE Soil and Water Division Terminology, ASAE S526.3). There were 223 active records not including current W2128 activities. Most of these active records can be categorized into 3 major categories that are not strongly related to the proposed project. These categories include a large number of projects that are primarily using microirrigation as a highly uniform irrigation or water application method to minimize experimental variability, a moderate number of studies where microirrigation is desirable for minimizing pest and disease control within the research, and a lesser number of projects using microirrigation to find a suitable alternative to fumigation with methyl bromide. The search found 33 records that could be classified as at least somewhat related to the efforts of the proposed project. Sixteen of these 33 records were either the current umbrella projects of the projected W3128 participants or were being conducted by colleagues at the same institution location.
A project at the USDA-ARS Arid-Land Center in Arizona is working to improve crop coefficients for irrigation scheduling. The project revision W3128 will use its current University of Arizona members and USDA-ARS-TX members to keep the project informed of this other projects results and to help reduce unnecessary duplication. Several other projects revealed in the CRIS search were related to efforts being conducted at the USDA-ARS WMRL at Parlier, CA. Although, the WMRL chose not to rejoin in the project revision to W3128 at this time, they will be following and monitoring the proposed W3128 efforts, and W3128 communication is excellent with the WMRL. It is likely that informal relationships with WMRL will continue for the foreseeable future, so the chance of unnecessary duplication is low and the chance for collaboration remains high. A project at the University of California-Davis that ended in 2012 examined the effect of drip irrigation on yield and fiber quality of cotton which has some similarity to efforts being conducted by W3128 participants in Texas and at USDA-ARS-TX. Because of the differences in climate, soils and varieties of cotton grown, W3128 does not project any unnecessary duplication, but there may be some complement to this previous work. Some soil water modeling work that ended in 2012 at the University of California-Riverside involved numerical modeling of water flow from surface and subsurface sources. As W3128 has soil physicists that also participate in W2188, Characterizing Mass and Energy Transport at Different Vadose Zone Scales, there will be no unnecessary duplication of research.
A search for possibly related NIFA multistate research projects revealed NC1186, Water Management and Quality for Ornamental Crop Production and Health; W2188, Characterizing Mass and Energy Transport at Different Vadose Zone Scales; and W2190, Water Policy and Management Challenges in the West. The first project NC1186 continues through 2015 and has 28 listed objectives which primarily are concerning ornamental crop production using a variety of irrigation technologies and has little or no activity at specifically optimizing or scaling of microirrigation technologies. The second project W2188 does have a few overlapping activities with the proposed W3128 as mentioned in the previous paragraph. These overlaps are in soil water sensor development and in numerical modeling of soil water flow and redistribution. However, the overlaps generally exist only for W3128 participants that are also members of W2188, so the chances for unnecessary duplication are small and the overall efforts of both projects should be complementary. The project W2188 is also being revised at this time. An examination of the proposed objectives on January 10, 2014 did not indicate any new overlaps with the proposed W3128. The last identified, potentially related project, W2190, is primarily investigating the effect of water policies on water availability and the resulting economics for western U.S. agriculture and does not appreciably overlap with activities in the proposed W3128 project.
A NIFA multistate research project, S1018, Irrigation Management for Humid and Sub-Humid Areas, that ended in 2010 had some similar activities to the proposed W3128. Some of that projects participants have migrated to W2128 and will continue with W3128.
Review of Progress in Current W2128 Microirrigation Project
The current project W2128, Microirrigation for Sustainable Water Use, had four objectives: 1) Compare irrigation scheduling technologies and develop grower-appropriate scheduling products; 2) Develop design, management and maintenance recommendations; 3) Develop best management practices for application of agrochemicals; and 4) Evaluate use of non-potable water through microirrigation. Overall accomplishments of the current project have been excellent as evidenced by 583 total publications and presentations summarized for the period 2009 through early 2013. Objectives 1 and 2 had 227 and 241 publications and presentations, respectively. The project participants are approximately 50% agricultural engineers, 34% plant scientists and 16% soil scientists, so it is natural these first two objectives garnered more activity. Although Objective 3 concerning microirrigation of fertilizers and agrochemicals only had about 16% of the total activities, it had an excellent cross section of the participants and professional disciplines contributing to its 93 total activities. Objective 4 concerning microirrigation usage of non-potable water still had 22 activities despite the fact that this remains an emerging technology in many regions and also that key W2128 participants engaged in this objective have retired. The accomplishments of the current project W2128 directly feed into further advances and the scaling of the microirrigation technologies that are envisioned in W3128. In some cases the proposed W3128 effort will be to step forward to the next advance in the technology (e.g., combining of irrigation scheduling approaches to increase robustness) while in other cases the effort will concentrate on packaging and promoting the technologies (e.g., smart phone applications of decision tools) that have already been developed in the history of this project since 1972. Some additional review of W2128 progress will be indicated in various topics discussed later in this section.
Current and Related Research Specific to Obj. 1.
Irrigation scheduling and water management takes on many forms (Hoffman et al., 1990). Some of these techniques are soil-based, some are plant-based, some are weather-based and some are a combination of two or more approaches.
Much of the current research emphasis on irrigation scheduling has focused on models of crop water demand, based on one or more weather-related factors that describe the evaporative demand of the atmosphere (e.g., FAO 56 in Allen et al., 1998). This approach assumes that the water use of any particular crop (the crops evapotranspiration, or ETc) can be calculated as a simple proportion of the calculated reference ET from a reference surface (typically either a short grass surface or a taller alfalfa surface) under the same weather conditions, using the relation ETc = Kc x ETref. The empirical proportionality constant (crop coefficient, Kc), is meant to include all effects specific to the crop in question (stage of canopy and plant development, crop health, degree of water stress, etc.), and many different resources have been developed to help growers adapt this approach for irrigation scheduling (Gowda et al., 2011, Rogers, 2012). This approach is very useful for planning purposes at a regional scale, but it requires a number of assumptions and/or site specific information (e.g., local rainfall, crop rooting depth) in order to be scaled appropriately for on-farm use. It also requires environmental information appropriate to the microclimate that is experienced by the crop, and in some cases this information is not widely available, or only available at a regional scale. One of the significant issues with the FAO Kc x ETref approach is the non-transferable nature of the Kc coefficients from one location to another, which justifies the need to develop alternative and more robust methodology for estimating crop water requirements. Hence, there is a need for continued development of this approach, in order to supply growers with more accurate and site-specific (e.g., growing degree day-based) or microirrigation system-specific (e.g., DI vs. SDI) estimates of Kc, forecast ET (reference) information for on-farm planning purposes, and web-based access to models that either measure or calculate local weather conditions (e.g., rainfall). Such improvements will be useful to growers as well as water planning authorities (e.g., Fares, 2013).
Soil-based approaches to irrigation scheduling (e.g., Casanova et al., 2012) are an alternative that may be more directly scalable to the needs of the grower than weather-based approaches, since they are based on local soil measurements. Since both soil- and weather-based approaches are founded on the same principles of crop water balance, they should give comparable results, and hence combining these approaches may provide mutual support for growers irrigation decisions. Soil water content is an important metric for managing timing and amounts of irrigation (Merriam, 1966; Evett et al., 2009). Due to the large rooting depth of some crops, measurements of the soil water profile down to >2 m are necessary in some cases to accurately capture the root zone soil water dynamics, particularly for soil water balance studies of crop water use. Passive and active microwave remote sensing are capable of sensing soil water, but only to depths of approximately 5 cm (Jackson and Schmugge, 1989). Neutron probes are accurate and can be used to appropriate depths, but are impractical for frequent measurements (less than a few days) and come with regulatory burdens due to the radiation source. Capacitance probes are often inaccurate due to heterogeneity in soil electrical conductivity and structure (Mazahrih et al., 2008; Evett and Steiner, 1995, Evett et al., 2009). Time-domain reflectometry (TDR) uses the travel time of an electric pulse sent down a waveguide in the medium to be measured (Topp et al., 1980). Travel time is related to the soil complex dielectric permittivity and is affected by pulse bandwidth and soil bulk electrical conductivity, with temperature effects on bound water and conductivity, as well as soil water content effects on conductivity. For TDR, these complicated interferences can be accurately calibrated by accounting for bound water and temperature effects (Schwartz et al., 2009). However, these calibrations are tedious, and conventional TDR probes are not easily installable to the depths required, so additional work in this area is needed.
Since the final objective of irrigation is to economically improve plant productivity, plant-based irrigation scheduling should in theory be scale independent, but plant responses to water limited conditions are complex (Jones, 2004), and the availability of reliable and relatively low-cost approaches to the grower are currently limited. Infrared thermometers (IRTs) have been widely used in agricultural research to remotely measure surface temperatures and develop vegetation stress indexes (Jackson et al., 1981; Colaizzi et al., 2003), monitor crop canopy temperatures and schedule irrigations based on plant feedback (Jones, 2004; Peters and Evett, 2007), predict crop yields (Irmak et al., 2000; Ajayi and Olufayo, 2004), or estimate sensible and latent heat fluxes (e.g. Kustas and Norman, 1997; Kalma and Jupp, 1990). Historically, these applications employed the use of stationary industrial IRTs wired to data loggers. These sensors can be expensive, cumbersome, and impractical for commercial on-farm applications. Recent wireless technology (OShaughnessy and Evett, 2010a; OShaughnessy et al., 2011) and internet access have improved the prospects for on-farm use of these devices, but further research is needed to evaluate these devices and to develop user-friendly systems and scheduling criteria that are appropriate to the crop of interest and the device used. The most direct, but also labor and/or technology intensive plant-based irrigation scheduling method is the measurement of plant water status, typically as measured by plant water potential. While the relevance of this measure to plant function has been questioned (Sinclair and Ludlow, 1985) a few early studies in woody perennial crop species (Garnier and Berger, 1985, McCutchan and Shackel, 1992) reported a close relation of midday stem water potential to both irrigation regime and plant physiological activity. This approach has been successfully used for deficit irrigation strategies in high value woody perennial crops (Shackel, 2011), but its application to herbaceous crops, while successful, has been rare (Campillo et al, 2008). The applicability of this approach to a wide range of crop species is warranted, as it can either serve as direct feedback to irrigation scheduling (e.g., Girona et al, 2006), or as a research tool to evaluate other indirect plant-based measures that can be automated, such as canopy temperature (Peters and Evett, 2008; OShaughnessy and Evett, 2010b) or stem diameter fluctuations (Conejero et al, 2011).
Current and Related Research Specific to Obj. 2.
When considering agrochemical application in conjunction with microirrigation, water quality protection must be considered in addition to improving crop production and improving agrochemical efficacy. Modeling approaches such as HYDRUS 2D can be used to identify both design and operational procedures for using microirrigation systems that can minimize leaching and runoff. For almost 90 years, it has been recognized that the movement of water and energy in the soil are coupled (Boucoyous, 1915). However, soil water movement in unsaturated soils is mostly studied under isothermal conditions based on the Richards equation (Richards, 1931). Early studies (Taylor and Cavazza, 1954) demonstrated that moisture movement in response to thermal gradients through an unsaturated soil occurs mainly in the vapor phase. The complexity of the coupled liquid water, water vapor and heat transport in the unsaturated zone and the difficulties associated with field measurements especially near the soil surface necessitate the application of numerical models to analyze these processes (Deb et al., 2011). Water transport under both liquid and vapor phases were studied involving laboratory (Hanks et al., 1967; Westcot and Wierenga, 1974; Nassar and Horton, 1992) and field (Ross et al., 1985; Scanlon and Milly, 1994; Saito et al., 2006; Deb et al., 2013) conditions. There have been limited modeling studies that evaluated coupled fluxes and their temporal variations in field soils where irrigations are commonly applied after short intervals (Deb et al., 2013b). The growing computational capacity has enhanced the use of more accurate simulation models coupled for water and energy transport in unsaturated soils (Saito et al., 2006). HYDRUS-1D is software capable of simulating water, heat and solute transport through variably saturated porous media (Simunek et al., 2008; Shukla, 2014).
Groundwater quality can greatly affect the sustainability of agriculture production because salinity produces an important abiotic stress and exacerbates the plant water stress further (Miyamoto and Storey, 1995; Deb et al., 2013a). Salinity stress is an ever-present environmental constraint to crop productivity in arid and semiarid regions and thus can affect the scaling and selection of irrigation systems. Salts present in the soil and irrigation water are expected to increase as the competition for freshwater uses among domestic, agriculture, and industrial sectors intensifies.
Among all the elements needed for plant growth, nitrogen (N) is considered the most important fertilizer element applied to soils because crop requirements for N are high compared with requirements for phosphorous (P), potassium (K), and other essential plant nutrients (Allison, 1996). However, solubility of nitrate (NO3) sources in water can cause rapid movement through soils, and among the various sources of N loss in agricultural fields, leaching is considered a major source of NO3-N loss under normal agricultural practices (Allison, 1996). Crops differ in rooting depths, rooting densities, N and water requirements, and plant uptake efficiencies (Peterson and Power, 1991), and the percolation of NO3-N to deeper soil layers depends on the cropping systems. In addition to N fertilizers and water applied by irrigation or received through precipitation, type of irrigation system and soil physical properties also play important roles in NO3-N leaching to groundwater (Al-Jamal et al., 1997; Cepuder and Shukla, 2002). In arid regions excess irrigation is also applied to flush salts out of the rooting zone to control soil salinization, leading to high N leaching. Microirrigation has the potential to supply water and N directly to crop roots and reduce water and NO3-N leaching to the deeper soil layers. Drip irrigation systems have been reported to apply 22% (Bucks et al., 1974) to 30% (Gustafson, 1975) less water than furrow irrigation systems. Higher onion yields, larger bulb sizes, less NO3-N leaching, higher water use efficiency, and higher N fertilizer use efficiency were reported under drip irrigation systems compared to furrow irrigation systems (Halvorson et al., 2008).
Universities in TX and KS and the USDA-ARS sites in TX (which are also participants in this proposed project) have considerable joint research activity concerning development of SDI as part of the USDA-ARS Ogallala Aquifer Program. KS has maintained a website devoted to SDI topics (SDI in the Great Plains, http://www.ksre.ksu.edu/sdi/) since 1998. Although there have been some very good summaries and discussion of SDI research efforts (Camp, 1998; Camp et al., 2000; Ayars, et al., 1999; Lamm and Trooien, 2003, Lamm and Camp, 2007) which have outlined many of the design considerations for SDI from a conceptual standpoint, there still remains a need to develop management procedures and design adaptations as SDI expands into new areas with different climates, soils and cropping systems. The proposed project can help to develop new and broaden existing guidelines, and to package the recommendations into more easily accessible platforms.
Current and Related Research Specific to Obj. 3.
This multistate project has been working since its initiation in 1972 to address practical issues related to application of microirrigation technology. Originally formulated as a western U.S. regional project concerning drip and trickle irrigation (W128), over the years the group has included multiple disciplines, such as agricultural engineers, plant and soil scientists, and agricultural economists from diverse regions (Caribbean to the Pacific U.S. Territories). The project was renumbered W1128 in 2004 and became W2128 in 2009. A major project accomplishment during the early years of the microirrigation project W128 was publication of the original reference book, Trickle Irrigation for Crop Production (Nakayama and Bucks, Eds., 1986). The author group of that books successor, Microirrigation for Crop Production (Lamm, Ayars, and Nakayama, Eds., 2007) involved 18 past or present project members. In a plenary paper at the 5th International Microirrigation Congress, Phene (1995) attributed the bulk of coordinated microirrigation research to the regional projects W128 and the now defunct S-247. The project has offered project-related technical sessions at the Irrigation Associations (IA) conferences in 2001, 2002, 2004, 2005, 2009, and 2012. The project has a long history of productivity through publications with 178 publications occurring under the previous project number, W1128 and over 600 publications and presentations to date in the current project W2128. Field Days and tours conducted by the W2128 participants help to achieve the second overarching outcome of the project, clientele interaction, and have been well attended and well received according to participant surveys. A major technology transfer effort of the current project W2128 was a major SDI technology transfer effort that began in 2009. This effort involving W2128 participants KS, TX, and USDA-ARS-TX had 216 activities (publications, presentations and/or tours) during the first two years since its inception. Effectiveness of some of these activities was documented in Porter, et.al., (2010). Another significant product of W2128 was the extensive microirrigation maintenance website http://micromaintain.ucanr.edu/ with joint collaboration of CA, TX, and KS. The W2128 project maintains its own website, http://www.cropinfo.net/MI/, and has begun to brand its creations with the recently adopted microirrigation logo. Based on the projects long history of technology transfer and the willingness to embrace a wide range of media, it is anticipated that W3128 will adequately address technology transfer.
Objectives
-
Develop robust and appropriately-scaled methods of irrigation scheduling using one or more soil-, plant- or weather-based approaches.
-
Develop microirrigation designs and management practices that can be appropriately scaled to site-specific characteristics and end-user capabilities.
-
Develop technology transfer products for a diversity of stakeholders to promote adoption of microirrigation.
Methods
Methods for Obj 1. Microirrigation can be scheduled using soil-, weather-, plant-based methods, or less frequently with some combination of these three methods. All of these methods can be appropriate and sufficiently robust under specific circumstances. Project participants will discuss planned protocols at the annual meetings to look for commonalities and ways to enlarge the common databases. Methods for Subobjective 1a (Development and Evaluation of Soil-Based Irrigation Scheduling) Technical advances in soil water sensors and their increasing commercial viability help focus the need for more research and extension of soil-based scheduling. Confirming the reliability and improving the convenience and accessibility to growers of soil water sensors will be addressed in a number of studies (ID, NM, OR, TX, USDA-ARS-GA, USDA-ARS-TX, WA). Wireless sensors will be tested in laboratory and field settings with comparisons against NIST standards (USDA-ARS-TX). Wireless soil water sensor development will also continue, as will investigations into COSMOS wide-area surface soil water content measurement as influenced by subsurface drip irrigation (SDI) and by green biomass (USDA-ARS-TX). Following calibration, a range of soil water sensors (e.g., neutron probe, capacitance probe, TDR) will be used for scheduling microirrigation in greenhouse experiments (NM), and soil water sensor data will be used to both augment and confirm ET-based irrigation scheduling in the greenhouse (NM) and field (TX). The NM scheduling results will be correlated with soil, meteorological, root, and plant physiological measurements and will be evaluated in terms of crop yield, water use and salt balance. Soil sensor trigger points to increase water productivity without reducing crop yield for shallow SDI will be established for peanut and its associated crop rotations (USDA-ARS-GA). Remote grower access to soil water data by computer or smart phone will be evaluated for irrigation scheduling in WA, OR, and ID. Methods for Subobjective 1b (Development and Evaluation of Weather-Based Irrigation Scheduling) Weather-based irrigation scheduling approaches over a range of scales in space (regional and local) and time (historical, current, and forecast conditions) will be developed and/or evaluated by several participants (AZ, FL, KS, NY, TX). While the number of public and private automated weather networks is increasing, there is still a lack of quality networks and adequate station density in some locales. TX will lead an effort investigating the use of alternative weather data and/or surrogate parameters to estimate ET for these locations. In FL high resolution, site-specific rainfall and other weather data will be obtained from commercial providers, compared to regional weather station data, and incorporated into an existing PC-based water balance model for irrigation of citrus and other crops. A web-based, orchard-specific irrigation scheduling system for apples based on micro-meteorological data collected by on-farm weather stations will be developed by NY. This product will improve time scaling with forecasted predictions of water use for as much as 5 days into the future. Crop coefficient values (Kc) are often considered to be a weak link in generalizing or transferring ET-based irrigation scheduling. More robust and generic Kc will be developed based on heat units for corn (KS), and multiple other crops (AZ, USDA-ARS-TX). IRT canopy temperatures will be correlated to daily calculated ET and also to the measured crop ET determined from a soil water balance (neutron probe), in order to develop a more robust and transferrable means of scheduling irrigation for SDI corn (KS, USDA-ARS-TX). Methods for Subobjective 1c (Development and Evaluation of Plant-Based Irrigation Scheduling) Direct and indirect measures of plant water status will be used to schedule, guide, and/or evaluate irrigation for several project participants (CA, KS, NM, TX, USDA-ARS-TX, WY). Thermal infrared thermometry (IRT) will be used to determine canopy temperature in corn and/or cotton (KS, TX, USDA-ARS-TX, WY) and almond (CA). A wireless computer vision system to determine both canopy temperature and physiological status will be investigated by USDA-ARS-TX. IRT and soil water content will be used to determine the timing of the onset of water and heat stress in corn and other crops (KS, WY). A more direct, but labor intensive plant-based method, midday stem water potential (mSWP), will be used in almond (CA) and pecan (NM). In both locations, mSWP will be correlated with additional leaf level physiological parameters such as stomatal conductance, photosynthesis and leaf temperature. Methods for Subobjective 1d (Software Development and Comparison of Multiple or Combined Irrigation Scheduling Methods) A large number of project participants plan to develop, modify, adapt, or expand their software tools and smart phone applications for irrigation scheduling (FL, ID, KS, TX, TX-PVAMU, WA, WY). As some of these activities interact with Objective 3 of this project, further discussion of this topic can be found there. Nearly all participants will impose irrigation treatments and will compare alternative methods, the most common comparison being soil- to weather-based methods (AL, CA, ID, KS, NE, OR) CA,), but also soil- to plant-based (CA, KS, PR, USDA-ARS-TX, WY), and in some cases all three scheduling methods (AL, CA, KS). USDA-NRCS will work with all participants to promote and extend these methodologies. This will help to improve conceptualization of these scheduling methods and scaling each to the appropriate need. In OR, soil-based scheduling for onion will be compared to weather-based scheduling, and a fixed irrigation schedule compared to soil- and weather-based scheduling for seed production from native plants. In KS and USDA-ARS-TX, corn canopy temperatures (IRT) will be correlated with crop growth stage and ET determined by neutron attenuation. In PR, typical growers’ irrigation of Meyer lemons will be compared to a 100% ET treatment with and without the use of reflective plastic soil covers. In another PR study two soil-based thresholds will be compared to rainfed production of Valencia oranges. Remotely sensed solar radiation, real-time Epan, historical Epan, and Penman-Monteith methods will also be compared under DI and SDI in vegetables, root crops, grains and forage legumes in PR. In CA, 70% – 110% ET irrigation will be imposed on three commercial almond orchards to determine a water production function. Soil water measurements and mSWP will be compared at all locations and two independent plant-based methods (IRT and mSWP) as well as a soil-based method will be compared at one location. In NE, soil water extraction, crop ET, crop growth and yield under SDI will be determined in maize, soybean, and winter wheat. Field experiments will also compare SDI, center pivot, and gravity irrigation methods for the same crops. In AL, yield for continuous corn under five irrigation levels based on pan evaporation will be measured, and continuous cotton will be compared to a corn-wheat-soybean rotation under SDI. In ID, 40% - 140% ET will be imposed on sprinkler and drip-irrigated sugar beets to better define the water production function as affected by irrigation method. Methods for Obj 2. Project participants will discuss planned protocols at the annual meetings to look for commonalities and ways to enlarge the common databases. Methods for Subobjective 2a (Improved Management for Soil Salinity and Source Water Quality Concerns) Soil salinity and the use of poor quality surface and ground waters are of growing concern in the arid and semi-arid regions of the world. A combination of modeling, field and educational activities will be used to address this issue involving participants from AZ, CA, CO, TX and USDA-ARS-TX. AZ will use numerical and analytical models for developing design and management practices for different crops, water quality, and soil characteristics. CA will conduct field studies with tree crops to assess field-scale leaching of water and nitrate rates when using microirrigation for various soils. Efforts will be made to couple and integrate HYDRUS 2D with the experimental data to estimate nitrate leaching rates. CO will compare source water quality (high quality surface water vs. saline shallow alluvial groundwater) for microirrigation of high-value horticultural crops (cantaloupe, watermelon, and chile peppers). Soil water movement, soil salinity distribution, crop yield, and fruit quality will be assessed. USDA-ARS-TX will investigate the use of treated wastewaters with tree (almond, olive and pomegranate) and vegetable crops, focused on nutrient loads and on using wireless IRT and soil water sensors for irrigation scheduling. TX will conduct educational activities to help producers address saline ground water concerns. Methods for Subobjective 2b (Improved Efficiency of Water and Nutrients) The use of SDI is growing in the US Great Plains. Water and nutrient use efficiency are both important factors in decision making of SDI use in the region. USDA-ARS-TX and KS will be conducting research to compare sprinkler and SDI irrigation methods for crop production. These two locations have differing soils and seasonal ETc, but have similar seasonal precipitation and these factors may influence the relative outcomes for the two methods. USDA-ARS-TX will conduct water and energy balance studies comparing sprinkler irrigation and SDI for cotton and corn production on a heavy silty clay loam soil. Weighing lysimeters will be used to measure evaporative losses and soil temperature profiles. Canopy temperature measured with IRTs will be related to soil water content. Crop yield, crop quality parameters, water use and water productivity will also be compared across the two irrigation methods. KS will compare corn grain yield, water use and water productivity for SDI and LEPA sprinkler irrigation on a well-drained silt loam soil. NM will conduct water and energy balance studies with SDI for chile production in greenhouse. Deep percolation, soil water content, leaf temperature, stomatal conductance, transpiration and photosynthetic rates will be measured under three different rates and methods of water application. Crop yield, water productivity, chemistry of irrigation, and drainage water, soil and leaf will be periodically determined for nutrient balance and plant nutrient uptake. Environmental conditions will be simultaneously measured to determine water and energy transport through soil using HYDRUS model. Several project participants (AL, KS, OR, PR, USDA-ARS-GA, WY) will work on conjunctive use of irrigation and nutrients for microirrigated crop production. USDA-ARS-GA will study chemical injection for fertility and pest control in cotton, corn and peanut cropping systems grown using strip tillage for shallow SDI systems in the US Southeastern Coastal Plain region. Peanut, the crop with the most economical profitability, must be grown in rotation with corn and cotton. This research will investigate dripline placement and timing of nutrient and herbicide applications for the three crops in terms of crop yields, chemical efficacy, soil chemical distribution and leaching. WY will develop DI and SDI water and fertigation management practices for corn for both grain and forage production. Timing of nutrient application will be controlled by general crop nutrient uptake curves and the results will be evaluated in terms of grain and biomass yields, water use and water productivity. Similar work will be conducted for horticultural crops grown in high tunnels. KS will investigate the application of micronutrients through fertigation for SDI corn with deep dripline placement (0.3 to 0.4 m). Soil and plant assays will be used to identify likely micronutrient candidates for inclusion in attempts to raise corn yield goals in long-term continuous corn. Results will be evaluated in terms of grain yields, water use, water productivity and nutrient uptake. AL will work on determining the appropriate fertigation timing for corn and cotton grown with SDI. Treatments will include surface and subsurface application of fertilizer as compared to a non-fertigated control treatment. BMPs for nutrient injection will be based on crop yield, water and nutrient use efficiencies, and nutrient leaching. OR will study the efficiency of phosphorous fertigation for drip-irrigated onion as early season growth of onion is often limited by P availability. Several strategies of P application will be compared along with several methods to test the P concentration in plants and in the soil. PR will investigate using microirrigation to help control HLB (citrus greening) disease. Different irrigation regimes and different fertilization strategies (fertigation, foliar sprays and combination) will be imposed to the covered (reflective plastic) and non-covered areas. The lemon trees’ leaf water potential will be determined using a pressure chamber to characterize obstruction in the vascular bundles caused by HLB. Trunk diameter, canopy volume, production, and soil water (TDR) will also be determined. Also, PR will continue studying the efficacy of slow release fertilizers and fertigation on mature avocado trees for another year. Nitrate and ammonium concentration in soil water below the root zone will be determined. TX-PVAMU will conduct experimental and modeling work (Hydrus 2d/3D) on conjunctive use of irrigation and nutrients formicroirrigated crop production. W3128 participants will compare the results of these studies to develop more generic procedures for water and nutrient applications with microirrigation and also to be able to rapidly extend (scale) these findings to other crops, soils, and regions. Methods for Subobjective 2c (Improved Designs and Performance of Microirrigation Systems) The scaling of microirrigation system size and the required components are typically considered to be engineering–related problems. Design, installation, and the resulting performance are key activities of a number of W3128 participants including AZ, ID, KS, OR, TX, USDA-ARS-TX and USDA-NRCS. ID and OR will evaluate SDI dripline placement (depth and spacing) and its effect on corn and sugarbeet water management. Similarly, TX, USDA-ARS-TX and KS will conduct research with SDI dripline placement and crop placement/tillage management aimed at improving seed germination and crop establishment under droughty conditions. TX and USDA-ARS-TX will be working with cotton while KS will be working with corn. TX will focus efforts on how SDI lateral/crop row position and the shifting of planting dates might affect cotton establishment and subsequently lint yield and quality and water productivity. Irrigation of all treatments will be at approximately 70% ETc, limited by an irrigation capacity of 5 mm/d. Preseason irrigations of up to 125 mm will be initiated 25 days prior to each planting date and will continue for up to 10 days following planting or until crop emergence has occurred. USDA-ARS-TX will focus efforts on alternative SDI bed and cropping designs (alternate furrow, wide-beds with twin crop rows, and one dripline/crop row) at various installation depths (15 to 30 cm) and irrigation regimes for cotton production with similar evaluation criteria to the TX study. KS will concentrate its efforts on evaluating corn establishment with two tillage management treatments (bed and flat planting) as affected by 3 emitter discharge rates (0.7 to 1.4 L/hr). Soil water will be measured at various depths and for various horizontal distances from the dripline. Crop emergence will be correlated with measured seed-zone soil water. AZ, KS, TX, and USDA-NRCS will work to scale system designs and technologies for small to larger commercial sized operations. Initial efforts in this regard will be to develop flow-charts to assess microirrigation suitability and design requirements building upon efforts of Clark et al. (2007). The results of these efforts will be useful in enhancing microirrigation design tools that are under development at the USDA-NRCS and also be feeding into products that are a part of Objective 3 of this project. Further efforts will then be conducted to generalize microirrigation design procedures by developing spreadsheets and software that can be scaled for system size and crop economic potential, recognizing that sometimes irrigation design is a mixture of science and art (i.e., more than one suitable design may exist). These participants will also collaborate on development of simplified, yet reliable methods of evaluating microirrigation performance for various size systems. Methods for Obj 3. Scaling of microirrigation includes development and adaptation of technology transfer products to a diversity of stakeholders. Efforts will focus on four primary activities: development and expansion of internet-based resources, decision tools and applications; development of print and multimedia content; coordination of educational events, and advancement and promotion of microirrigation through public-private partnerships. Methods for Subobjective 3a (Development and expansion of internet-based resources, decision tools and applications) Internet-based delivery of information has become a mainstay for technology transfer, and well-established websites will continue to serve as primary sources of information for many stakeholders. Although the publications and products of the current W-2128 project are thoroughly listed on the project website http://www.cropinfo.net/MI/ many of these documents cannot be easily obtained through active Internet links. In the proposed project, all participants will work to improve free and open access to publications and products for all our global stakeholders. Since much information remains underutilized, project participants will work together to increase availability of information in audience-appropriate formats. Participating programs in KS, NE, OR, TX, and USDA-ARS-TX will leverage existing Internet resources to deliver new and expanded tools and information resources, including adapting existing decision support tools or new applications (apps) for smartphones, tablets and other mobile devices, and provide training for end-users. Irrigation scheduling tools and apps are available or under development by participants in AZ, KS, TX, USDA-ARS-TX, WA, and WY; and new and expanded tools are planned by these participants and others (FL, ID, TX-PVAMU, WA). For instance, ID will work with WA to adapt Washington State University’s Mobile Irrigation Scheduler app for Idaho crops and conditions; TX will work with USDA-ARS-TX to adapt and promote a mobile app of the Bushland Reference ET Calculator; and FL will lead an effort developing irrigation scheduling mobile app(s) for specialty crops and low volume turf applications in the southeastern U.S. New apps to support design and management decisions for microirrigation systems are proposed by teams in KS and TX-PVAMU. CO aims to use web-based resources as a platform to increase grower-to-grower interaction. Higher level integration of information from multiple sources for use in decision support will be made possible through development of new platforms. TX plans to integrate multiple data streams and models through an Internet-based “dashboard” package. KS will initiate the MIChoice suite of decision tools for microirrigation, with goals to help generalize and standardize the procedural order of questions and the format of input data to successfully use microirrigation. Initial proof-of-concept design and decision tools will be MIWaterQuality and MIFilter, expected to be easiest to develop and implement. These will be implemented as smart phone apps. Long term goals are to develop flowchart-driven decision tools to help potential end users or system providers decide if microirrigation is the right choice and to help lead them through the design process. Methods for Subobjective 3b (Development of print and multimedia content) Building upon existing conventional libraries of content, participants in ID, KS, NE, NY, OR, TX, USDA-ARS-TX, USDA-NRCS, WA, WY will expand subject matter and reach a greater diversity of stakeholders by emphasizing audience appropriate presentation (user-friendly language and formats; appropriate technical level) and greater accessibility. TX, KS, and USDA-NRCS will collaborate on technology transfer products and programs to promote efficient irrigation methods (including microirrigation) and management practices, providing outreach for expanded and emerging audiences, (small acreage landowners, off-site landlords, policy makers), in addition to traditional audiences (agricultural producers, irrigation professionals, and crop consultants). KS, NE, TX, and USDA-ARS-TX will develop publications on design, operation, management and maintenance of SDI for field crops in the Great Plains. ID, OR, WA will develop a “buyer’s guide” for agricultural microirrigation addressing crop-specific benefits; suitability (crops, soils, water quality and supply, and other site conditions); and factors to consider in evaluating contractor bids. NY will develop an extension bulletin (print and web) on irrigation methods and scheduling for high density apple orchards. WY will develop publications to help growers improve irrigation for field and horticultural crops. These products, primarily distributed through websites, will be promoted through educational events, mass media (print, radio), and other targeted means (listservs and newsletters). Methods for Subobjective 3c (Coordination of Educational Events) Educational events remain key to technology transfer. Workshops, field days, technical sessions, webinars, and conferences will be achieved through venue-appropriate and audience-appropriate activities. Stakeholder targeted field days, workshops and conferences will be conducted (AL, CA, FL, ID, OR, KS, NE, TX, USDA-ARS-TX, WA, WY). These activities may include events sponsored by industry, commodity groups and trade associations. Some activities will build upon foundations of traditional (often perennial) events; others will require coordinating and marketing of new events (additional venues, audiences). Technical sessions or conferences will be organized and supported by all Project Participants, and may be in conjunction with regional, national and international organizations (e.g., The Irrigation Association, American Society of Agricultural and Biological Engineers, Central Plains Irrigation Association). Where applicable, Continuing Education Units (CEU) or Professional Development Hours (PDH) credits will be offered. The project has had success with these outreach outlets in the past. If funding can be secured, the project proposes to conduct a stand-alone technical conference or symposium to be held in the United States during the latter two years of the project. Methods for Subobjective 3d (Advancement and Promotion of Microirrigation through Public-Private Partnerships) Public-private partnerships, including cooperative research and development agreements (CRADA) can be highly effective technology transfer strategies. USDA-ARS-TX will continue technology transfer of wireless sensors and SCADA systems for irrigation management through CRADAs with private firms. USDA-NRCS will leverage technology transfer through financial assistance programs (cost sharing) to promote promising technologies and tools. CA will cooperate with companies in Australia/Canada (ICT), Israel (Phytech), and the US (PMS) to improve both direct and indirect plant-based sensors of water stress and their use in irrigation scheduling. Project participants will initiate more formal advancement and promotional activities with industry. Based on preliminary informal discussions held at the last annual meeting of W-2128 (November 2013), there is general agreement between interested parties (i.e., industry and W2128 participants) that closer cooperation can be achieved. Anticipated benefits of these alliances will be greater access to microirrigation information and improved conceptualization and generalization of design, installation and maintenance processes, leading to increased adoption of microirrigation.Measurement of Progress and Results
Outputs
- Under Obj 1a) Soil-based microirrigation scheduling procedures and technologies (ID, KS, NM, OR, TX, USDA-ARS-GA, USDA-ARS-TX, USDA-NRCS, WA)
- (Under Obj 1b) Weather-based microirrigation scheduling procedures and technologies (AZ, FL, KS, NY, USDA-ARS-TX, USDA-NRCS)
- (Under Obj 1c) Plant-based microirrigation scheduling procedures and technologies (CA, KS, NM, TX, USDA-ARS-TX, USDA-NRCS, WY)
- (Under Obj 1d) Software and phone applications for microirrigation scheduling (FL, ID, KS, TX, TX-PVAMU, WA, WY)
- (Under Obj 1d) Comparison or implementation of multiple or combined microirrigation scheduling methods (AL, CA, ID, KS, NE, OR, PR, USDA-ARS-TX, USDA-NRCS, WY)
- (Under Obj 2a) Knowledge base for handling soil salinity and source water quality concerns (AZ, CA, CO, TX, USDA-ARS TX)
- (Under Obj 2b) BMPs for water and/or nutrients when using microirrigation (AL, KS, NM, OR, PR, USDA-ARS-TX, USDA-ARS-GA, WY)
- (Under Obj 2c) Adaptation and scaling of microirrigation system designs and practices (AZ, ID, KS, OR, TX, USDA-ARS-TX, USDA-NRCS)
- (Under Obj 3a) Expansion of existing and development of new internet resource knowledge bases, software decision tools and smartphone applications (AZ, CO, FL, KS, NE, OR, TX, TX-PVAMU,USDA-ARS-TX, WA, WY)
- (Under Obj 3b) Expansion of existing libraries of publications (print and/or electronic formats) to reach diverse audiences (ID, KS, NE, NY, OR, TX, USDA-ARS-TX, USDA-NRCS, WA, WY)
- Under Obj 3c) Educational events for diverse audiences (AL, CA, FL, ID, OR, KS, NE, TX, USDA-ARS-TX, WA, WY)
- Under Obj 3d) Advancement and promotion of microirrigation through public-private partnerships (All project participants)
Outcomes or Projected Impacts
- Soil-, weather-, and plant-based or combined microirrigation scheduling approaches will provide valuable choices for a diverse clientele of growers to improve their crop production and profitability while reducing irrigation withdrawals.
- Increased adoption and proficient management of microirrigation scheduling by growers will improve water productivity and promote improved water quality over a wider range of end-user characteristics and constraints.
- Risks of negative impacts to environment, soil, and water quality that affect all of society will be minimized through reduced leaching or other off-site /non-target chemical movement possibly opening up previously non-productive lands to microirrigation.
- Benefits of microirrigation to growers will be more fully realized through application of improved fertigation practices that will better match fertilizer applications (rates, timing, placement and formulations) to crop requirements.
- Conceptualization and generalization of microirrigation design and management procedures and tools will allow for growers, technical service providers, dealers, and industry to communicate more easily the requirements and preferences for new system installations.
- Improved design and management procedures for SDI will allow broader penetration of microirrigation into regions of the country where adoption has been limited.
- Comparisons of alternative irrigation systems will allow growers to make the best system choice for their operations and will allow them to optimize performance of existing systems.
- Internet-based decision tools and apps (for smartphones, tablets, etc.) will provide convenient, easily updated, and audience-tailored content to help end-users improve irrigation scheduling, water and nutrient management, and system design.
- Expansion of existing websites and accessibility of publications will allow for increased regional, national and international adoption of microirrigation technology.
- Educational events tailored to the audience abilities and needs will increase adoption and correct usage of microirrigation.
- Technical sessions and/or conferences will bring together experts representing different approaches to microirrigation; expand the knowledge base; improve networking of scientists and contribute to a more integrated approach to this field of research and education.
- Increased collaboration between public and private entities will help to increase adoption of microirrigation on a much broader scale and will improve the correct implementation of this technology.
Milestones
(2015): Laboratory and field studies to select, develop, calibrate, adapt, and evaluate soil water sensors begin. Software development/adaptation/modification and field studies involving weather-based irrigation scheduling begin. Plant-based sensor development/modification and their evaluation in field studies begin. Field studies to evaluate single, multiple, or combined irrigation scheduling techniques begins. Modeling, field, and educational efforts to address soil salinity and poor source water quality begin. Field studies will be initiated to determine optimal DI and SDI water and nutrient management for various crops. Initial discussions and outlines will be developed for both publications and software being jointly developed or adapted to a new locale. Tours, field days and educational events will be held at the local and regional level to promote microirrigation. Outline of needs for small holder systems will be developed in cooperation with USDA-NRCS. Conduct roundtable discussions with industry partners to identify needs and possible areas of cooperation. Initiate a pilot joint activity with industry to gage opportunities and challenges of such activities.(2016): All laboratory, modeling and field studies initiated in 2015 for Objectives 1 and 2 will continue using any strategy adjustments determined useful from first year results. Map out any new or adjust any existing website decision trees and identify major information and subject matter gaps. Test software and smartphone applications at their current stage of development (i.e., stage varies between participants and products) and identify gaps. Continue educational events at local and regional level and evaluate additional needs of those audiences. Draft extensive publication for SDI design and management for commodity crops. Evaluate success of pilot joint activity with industry and adjust strategy for future activity. Identify additional joint activities with industry and initiate those that can be accomplished within constraints. Identify potential funding sources for national or international conference on microirrigation.
(2017): Overall structures of websites will be completed and easily-accessible and audience-tailored content be increased. Initial laboratory, modeling, and field studies will be completed and analysis will be underway. Identify and initiate any follow-on laboratory, modeling, and field studies needed for project completion. Release extensive publication for SDI design and management for commodity crops. Evaluate progress with written publications in terms of accessibility and meeting audience needs and begin effort to fill gaps. Continue educational events at local and regional level and offer technical session at national conference. Make decision on whether to conduct national or international microirrigation conference and appoint planning team if appropriate. Continue joint activities with industry and identify new opportunities.
(2018): Finish analysis of all initial laboratory, modeling and field studies that began in 2015 and publish results. Finish any follow-on laboratory, modeling, and field studies that began in 2017 and begin analysis of results. Finish all software and smart phone application developments and promote products through appropriate venues. Finish drafts for any publication gaps identified in 2017. Plan and prepare for national or international microirrigation conference. Reevaluate collaborations with industry and make adjustments. Plan for revision of W3128 microirrigation project.
(2019): Release any remaining software and smart phone applications to public domain. Finalize all reports and make presentations at local and regional meetings. Conduct national or international microirrigation conference. Summarize project-wide accomplishments and present at national or international meetings. Finis
Projected Participation
View Appendix E: ParticipationOutreach Plan
Objective 3 of this project primarily focuses on the outreach requirements necessary to appropriately scale microirrigation and thus promote its use to a broader group of end-users. The reader is referred to the Objective 3 section of the Methods for additional details. Overall, a broad mix of traditional and non-traditional educational mediums will be used in outreach. This will include but will not be limited to field days, tours, demonstration sites, college class seminars, targeted training sessions (e.g. NRCS staff, Consultants), regional, national, and international conferences, newsletters, newspaper and popular press articles, audio-visual CDs and DVDs, Powerpoint presentations, factsheets, extension bulletins, research publications, refereed journal articles and Internet-based educational material.
A necessary outreach requirement in the proposed project will be to assess the appropriateness and accessibility of currently existing microirrigation technology transfer products within the participants own institutions. This assessment will identify which products might need adaptation/modification to reach a diverse clientele and will also determine what steps are necessary for greater accessibility. New publications and products developed during the course of the project will need to meet the criteria of having an appropriate format (i.e., meets scope and technical needs of potential end-user groups) and be easily accessible.
The internet has become the method of choice for information retrieval for much of the global group of end-users. The use of centralized website repositories that are easily revealed by search engines will be encouraged. Many of the project participants already have experience with these centralized repositories such as the project own website http://www.cropinfo.net/MI/index.php, SDI in the Great Plains website, http://www.ksre.ksu.edu/sdi/, and the Advanced Sensing and management Technologies to Optimize Resource Use in Deciduous Tree Crops website, http://ucanr.edu/sites/scri/.
Educating agricultural producers is a critical part of the successful implementation of microirrigation technology. A number of the participants have full or partial Cooperative Extension appointments, but all participants have an expectation of outreach. However, educating technical service providers (TSP) and consultants who are often largely responsible for microirrigation information interpretation is of equal importance. The Project will conduct targeted training sessions for TSPs, consultants and staff from Cooperative Extension, USDA-NRCS, other state and local agencies. Project participants will also improve communication and networking with microirrigation industry partners to identify informational needs and will work cooperatively with industry to fill those needs. The project anticipates that these efforts will rapidly identify gaps in current technology transfer and will accelerate our ability to appropriately adapt material (i.e. a necessary scaling aspect) to new and emerging audiences.
Project participants will conduct technical sessions at national or international conferences during the latter two years of the project to reach their technical peers and to broaden the networking of microirrigation scientists and engineers. If funding can be secured, the project will propose an international conference on microirrigation to be held in the U.S. during the final two years of the project. Potential co-sponsors/financial backers would include, but not be limited to the Irrigation Association (IA), the American Society of Agricultural and Biological Engineers (ASABE), and the American Society of Agronomy, Crop Science Society of America and Soil Science Society of America (ASA-CSSA-SSSA).
Organization/Governance
The organization and implementation of the project will be in accordance with the Manual for Cooperative Regional Research.
The Regional Technical Committee will consist of representatives from each cooperating Agricultural Experiment Station and federal agency cooperating in this project. The representative(s) will be appointed by their respective Experiment Station or Research Director. The above will constitute the voting membership of the technical committee.
The Regional Technical Committee will be responsible for the planning and execution of the research project. It will be responsible for coordinating research activities of each cooperating Experiment Station and federal agency and for the developing of appropriate research methods and procedures.
A Director from the Agricultural Experiment Stations of the Western Region appointed by the Agricultural Experiment Station Directors of the Western Region will serve as Administrative Advisor and an ex-officio (non-voting) member of the technical committee. A representative of the USDA National Institute of Food and Agriculture (NIFA) will serve as an ex-officio (non-voting) member of the technical committee.
An executive committee, consisting of a chair, vice-chair, and secretary will be elected from the voting members of the technical committee. The executive committee will serve one year in each elected office with the provision that the vice-chair will ascend to chair, and the secretary to vice-chair. A secretary will be elected each year. The executive committee will have the authority to act on behalf of the technical committee.
The chair, with the approval of the Administrative Advisor, will notify technical committee members of the time and place of meetings, prepare the agenda, and preside at meetings of the technical committee and executive committee. The chair will also be responsible for naming appointments to subcommittees for specific assignments. The chair will be responsible for annual and final reports. In the absence of the chair, the vice-chair will perform these duties. The secretary will record and distribute the minutes of the meetings.
Literature Cited
Ajayi, A. E. and A. A. Olufayo. 2004. Evaluation of two temperature stress indices to estimate grain sorghum yield and evapotranspiration. Agron. J. 96, 12821287.
Al-Jamal, M. S., T. W. Sammis, and T. Jones. 1997. Nitrogen and chloride concentration in deep soil cores related to fertilization. Agricultural Water Management. 34: 1-16.
Allen, R. G., L. S. Pereira, D. Raes, and M. Smith. 1998. Crop evapotranspiration, guidelines for computing crop water requirements. FAO Irrig. and Drain. Paper 56. Food and Agric. Organ. United Nations, Rome, Italy. 300 pp.
Allison, F. E. 1996. The fate of nitrogen applied to soils. Advances in Agronomy. 18, 219-258.
Ayars, J. E., C. J. Phene, R. B. Hutmacher, K. R. Davis, R. A. Shoneman, S. S. Vail, R. M. Mead. 1999. Subsurface drip irrigation of row crops: a review of 15 years of research at the Water Management Research Laboratory. Agric. Water Mgt. 42:1-27.
Bouyoucos, G. T. 1915. Effect of temperature on the movement of water vapor and capillary moisture in soils. J. Agric. Res. 5:141172.
Bucks, D. A., L. J. Drie, and O. F. French. 1974. Quality and frequency of trickle and furrow irrigation for efficient cabbage production. Agronomy Journal. 66: 53-56.
Camp, C. R. 1998. Subsurface drip irrigation: A review. Trans. ASAE. 41(5)1353-1367.
Camp, C. R., F. R. Lamm, R. G. Evans, and C. J. Phene. 2000. Subsurface drip irrigation: Past, present and future. In proc. of the 4th Decennial Natl Irrigation Symp., Phoenix, AZ, Nov. 14-16. pp. 363-372.
Campillo C, M. H. Prieto, C. Daza, M. J. Monino, M. Garcia, I. Goodwin, and M. G. OConnel. 2008. Use of stem water potential measurements to correct crop water needs estimations in a rotation of two horticultural crops. Acta Horticulturae 72:147-154.
Casanova, J. J., S. R. Evett, R. C. Schwartz. 2012. Design and field tests of an access-tube soil water sensor. Appl. Eng. Agric. 28(4):603-610).
Cepuder, P. and M. K. Shukla. 2002. Groundwater nitrate in Austria: A case study in Tullnerfeld. Nutrient Cycling in Agro ecosystems. 64: 301-315.
Clark, G. A., D. Z. Haman, J. F. Prochaska, and M. Yitayew. 2007. General system design principals. Ch. 5 in Microirrigation for Crop Production - Design, Operation and Management. F.R. Lamm, J.E. Ayars, and F.S. Nakayama (Eds.), Elsevier Publications. pp. 161-220.
Colaizzi, P. D., E. M. Barnes, T. R. Clarke, C. Y. Choi, and P. M. Waller. 2003. Estimating soil moisture under low-frequency surface irrigation using Crop Water Stress Index. J. Irrig. Drain. Eng. 129, 2735.
Conejero W., C. D. Mellisho, M. F. Ortuno, A. Galindo, F. Perez-Sarmiento and A. Torrecillas. 2011. Establishing maximum daily trunk shrinkage and midday stem water potential reference equations for irrigation scheduling of early maturing peach trees. Irrigation Science 29(4): 299-309.
Deb S. K., M. K. Shukla, P. Sharma, and J. Mexal. 2011. Coupled liquid water, water vapor, and heat transport simulations in an unsaturated zone of a sandy loam field. Soil Science. 176(8):387-398.
Deb S. K, P. Sharma, M. K. Shukla and T. W. Sammis. 2013a. Drip-irrigated pecan seedling response to irrigation water salinity. Hort. Science. 48:1-8.
Deb, S. K., M. K. Shukla, J. Simunek, and J. G. Mexal. 2013b. Evaluation of spatial and temporal root water uptake patterns of a flood-irrigated pecan tree using the HYDRUS (2D/3D) model. ASCE, Irrigation and Drainage Engineering. 139: 599-611.
Evett, S., and J. Steiner. 1995. Precision of neutron scattering and capacitance type soil water content gauges from field calibration. Soil Sci. Soc. America J. 59(4): 961-968.
Evett, S. R., R. C. Schwartz, J. A. Tolk, and T. A. Howell. 2009. Soil profile water content determination: Spatiotemporal variability of electromagnetic and neutron probe sensors in access tubes. Vadose Zone J. 8(4): 926-941.
Fares, A. 2013. Water Management Software to Estimate Crop Irrigation Requirements for Consumptive Use Permitting In Hawaii: Version 2.0. Final Report for the Commission on Water Resource Management, Hawaii Department of Land and Natural Resources. Final Report.
Garnier E and A. Berger. 1985. Testing water potential in peach trees as an indicator of water stress. J. Horticultural Science, 60, 47-56
Girona J, M. Mata, J. del Campo., A. Arbones, E. Bartra, and J. Marsal. 2006. The use of midday leaf water potential for scheduling deficit irrigation in vineyards. Irrigation Science 24(2): 115-127.
Gowda, P., T. A. Howell, J. R. Ennis, T. H. Marek, and D. O. Porter. 2011. Bushland, Texas Reference ET Calculator [abstract]. ASA-CSSA-SSSA Annual Meeting Abstracts. Paper No. 68-7.
Gustafson, C. D. 1975. Drip Irrigation-Worldwide 1975, Present Status and Outlook for Drip Irrigation. Survey Report of University of California, San Diego, CA, pp. 1-5.
Halvorson A. D., F. C. Schweissing, M. E. Bartolo, C. A. Reule, and A. Berrada. 2008. Nitrogen effects on onion yield under drip and furrow Irrigation. Agronomy Journal.. 100: 1062-1069.
Hanks, R. J., H. R. Gardner, and M. L. Fairbourn. 1967. Evaporation of water from soils as influenced by drying with wind and radiation. Proc. Soil Sci. Soc. Am. 31:593598.
Hoffman, G. J., T. A. Howell, and K. H. Solomon. 1990. Management of farm irrigation systems. ASAE Monograph. ASAE, St. Joseph MI. 1015 pp.
Irmak, S., D. Z. Haman, and R. Bastug. 2000. Determination of crop water stress index for irrigation timing and yield estimation of corn. Agron. J. 92, 12211227.
Jackson, R. D., S. B. Idso, R. J. Reginato, and P. J. Pinter. 1981. Crop canopy temperature as a crop water stress indicator. Water Resour. Res. 17, 11331138.
Jackson, T. and T. Schmugge. 1989. Passive microwave remote sensing system for soil moisture: Some supporting research. IEEE Trans. on Geoscience and Remote Sensing 27(2): 225-235.
Jones, H. 2004. Irrigation scheduling: advantages and pitfalls of plant-based methods. J. Exp. Bot. 55, 24272436.
Kalma, J. D and D .L. B. Jupp. 1990. Estimating evaporation from pasture using infrared thermometry: evaluation of a one-layer resistance model. Agric. Forest Metero. 51, 223246.
Kustas, W. P. and J. M. Norman. 1997. A two-source approach for estimating turbulent fluxes using multiple angle thermal infrared observations. Water Resour. Res. 33, 14951508.
Lamm, F. R., J. E. Ayars, and F. S. Nakayama (Eds.). 2007. Microirrigation for Crop Production - Design, Operation and Management. Elsevier Publications. 608 pp.
Lamm, F. R. and C. R. Camp. 2007. Subsurface drip irrigation. Chapter 13 in Microirrigation for Crop Production - Design, Operation and Management. F.R. Lamm, J.E. Ayars, and F.S. Nakayama (Eds.), Elsevier Publications. pp. 473-551.
Lamm, F. R. and T. P. Trooien. 2003. Subsurface drip irrigation for corn production: A review of 10 years of research in Kansas. Irrig. Sci. 22(3-4):195-200.
Mazahrih, N., N. Katbeh-Bader, S. Evett, J. Ayars, and T. Trout. 2008. Field calibration accuracy and utility of four down-hole water content sensors. Vadose Zone J. 7(3): 992-1000.
McCutchan, H and K. A. Shackel. 1992. Stem-water potential as a sensitive indicator of water stress in prune trees (Prunus domestica L. cv. French). J. American Soc. Horticultural Science, 117, 607-611.
Merriam, J. 1966. A management control concept for determining the economical depth and frequency of irrigation. Trans. ASAE 9(4): 492-498.
Miyamoto, S., and J. B. Storey. 1995. Soil management in irrigated pecan orchards in the southwestern United States. HortTechnology 5:219222.
Nakayama, F. S. and D. A. Bucks, Eds. 1986. Trickle Irrigation for Crop Production - Design, Operation and Management. Elsevier Publications. 383 pp.
Nassar, I. N. and R. Horton. 1992. Simultaneous transfer of heat, water, and solute in porous media: I. Theoretical development. Soil Sci. Soc. Am. J. 56:13501356.
OShaughnessy, S. A., and S. R. Evett. 2010a. Developing wireless sensor networks for monitoring crop canopy temperature using a moving sprinkler system as a platform. Applied Eng. in Agric. 26(2), 331-341.
OShaughnessy, S. A., and S. R. Evett. 2010b. Canopy temperature based system effectively schedules and controls center pivot irrigation for cotton. Agric. Water Mgmt. 97(9), 1310-1316.
OShaughnessy, S. A., M. A. Hebel, S. R. Evett, and P. D. Colaizzi. 2011. Evaluation of a wireless infrared thermometer with a narrow field of view. Comput. Electron. Agric. 76(1), 59-68.
Peters, R. T. and S. R. Evett. 2007. Spatial and temporal analysis of crop stress using multiple canopy temperature maps created with an array of center-pivot-mounted infrared thermometers. Trans. ASABE 50, 919927.
Peters, R. T., and S. R. Evett. 2008. Automation of a center pivot using the temperature-time-threshold method of irrigation scheduling. J. Irrig. Drainage Engr. 134 (3), 286-290.
Peterson, G. A. and J. F. Power. 1991. Soil, crop, and water management. In: Managing nitrogen for groundwater quality and farm profitability, R. F. Follett et al., Eds., pp. 189198, SSSA, Madison, WI.
Phene, C. J. 1995. Research trends in microirrigation. In: Microirrigation for a changing world. Proc 5th Intl. Microirrigation Congress. F. R. Lamm, (Ed.), St. Joseph, Michigan. ASAE. pp. 6-24.
Porter, D. O., D. Rogers, T. Marek, F. Lamm, N. Klocke, M. Alam, and T. Howell. 2010. Technology transfer: Promoting irrigation progress and best management practices. In: 5th National Decennial Irrigation Symposium, December 5-8, 2010, Phoenix, AZ. CD-ROM Proceedings, M. Dukes ed. St Joseph Mich: ASABE. ASABE Publication Number 711P0810cd
Richards, L. A. 1931. Capillary conduction of liquids through porous media. Physics 1:318333.
Rogers, D. H. 2012. Introducing the Web-based Version of KanSched: An ET-based Irrigation Scheduling Tool. In: Proc. 24th annual Central Plains Irrigation Conference, Colby, Kansas, Feb. 21-22, 2012. Available from CPIA, 760 N.Thompson, Colby, KS. pp. 230-236.
Ross, P. J., J. Williams, and R. L. McCown. 1985. Soil temperature and the energy balance of vegetative mulch in the semiarid tropics: II. Dynamic analysis of the total energy balance. Aust. J. Soil Res. 23:515532.
Saito, H., J. Simunek, and B. P. Mohanty. 2006. Numerical analysis of coupled water, vapor, and heat transport in the vadose zone. Vadose Zone J. 5:784800.
Schwartz, R. C., S. R. Evett, and J. M. Bell. 2009. Complex permittivity model for time domain reflectometry soil water content sensing: II. Calibration. Soil Sci. Soc. America J.73(3): 898-909.
Shackel, K. A. 2011. A plant-based approach to deficit irrigation in trees and vines. Hort.Sci. 46:173-177.
Sinclair, T. R. and M. M. Ludlow. 1985. Who taught plants thermodynamics? The unfulfilled potential of plant water potential. Aust. J. Plant Physiol. 12:213-217.
Topp, G., J. Davis, and A. Annan. 1980. Electromagnetic determination of soil water content: Measurements in coaxial transmission lines. Water Resources Res. 16(3): 574-582.