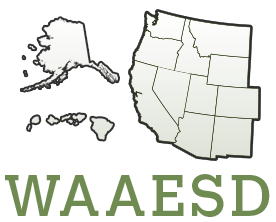
W2171: Germ Cell and Embryo Development and Manipulation for the Improvement of Livestock
(Multistate Research Project)
Status: Inactive/Terminating
W2171: Germ Cell and Embryo Development and Manipulation for the Improvement of Livestock
Duration: 10/01/2009 to 09/30/2014
Administrative Advisor(s):
NIFA Reps:
Non-Technical Summary
Statement of Issues and Justification
Upon establishment of the W-1171 Regional Research Project twenty years ago, the overall goal was the development of a cooperative, multistate research group comprised of basic and applied scientists to investigate the biological mechanisms underlying germ cell and embryonic development so that these processes could be manipulated for the improvement of livestock. Although we have made significant strides in the production of genetically enhanced livestock, there remains a gap in knowledge regarding our understanding of the basic biological mechanisms underlying the techniques used to produce these unique animals. Furthermore, most of these methodologies remain extremely inefficient. The efficiencies of these technologies will have to be substantially improved if we are to benefit from the advantages of genetically enhanced farm animals for human food and fiber production. These advantages include the production of novel, more desirable, health conscious or value-added foodstuffs and more efficient use of natural resources for preservation of the environment. Herein, we request to continue pursuit of our research priorities and renew the W-1171 Regional Research Project with the overall goal of producing genetically enhanced animals to improve the efficiency of livestock production systems.
The major aim of this research falls under Strategic Goal 2 (Enhance the competitiveness and sustainability of rural and farm economies) of the CSREES Strategic Plan (2007-2012). Objective 2.2 under this goal is to provide research, education, and extension to increase the efficiency of agricultural production and marketing systems. The labors of the Project members toward this goal and objective will be evaluated according to the following performance criteria: 2.2.7 - Increase and improve the reproductive performance of animals (CSREES Knowledge Area 301); 2.2.9 - Develop and apply information and technology for genetic improvement of animals (CSREES Knowledge Area 303); and 2.2.11 - Improve understanding of fundamental animal physiological processes (CSREES Knowledge Area 305). In addition, research to increase the practicality of making genetically enhanced livestock animals is directly in line with the Food Animal Integrated Research (FAIR) 2002 Goal 1 (Strengthen global competitiveness: Develop the systems needed to keep American animal agriculture competitive in the global market). Objective l under this goal is to enhance production efficiency and economic strategies at the farm and ranch level. Goal 5 (Ensure environmental quality: Devise animal production and processing systems that sustain or improve the environment) is also relevant to the research proposed under this Project. Objective 2 of this goal is to develop optimal production practices that promote animal health (Anonymous, 2003).
Beneficiaries of this multistate research endeavor include: 1) livestock producers in the Western states, as well as farmers and ranchers across the country; 2) rural communities of the West; 3) consumers of animal products within the Western region, U.S. and the world; and 4) the scientific community worldwide. Livestock producers will benefit from increased profits as a result of reduced input costs linked to efficient production systems, improved performance of animals, and value-added products. The economic stimulus afforded to a rural community that is located near a profitable and sustainable animal industry can be dramatic, providing many opportunities otherwise unavailable to its residents and enhancing the quality of life. Consumers will be impacted by reduced food prices associated with increased efficiency of livestock production, meat products with enhanced health benefits, an improved environment resulting from livestock systems producing less waste and the availability of food sources to meet the demands of an ever increasing population at both the national and international level. Investigators within the scientific community will also benefit from the efforts of the Project members. The use of gene transfer alone or in combination with NT is very useful for obtaining a variety of experimental information. Some examples are insight into the cell cycle, nuclear and cytoplasmic programming or reprogramming, genomic imprinting, gene expression, epigenetics and developmental processes. This information can be used in studies to examine basic biological, biomedical, genetic and evolutionary questions, in addition to agriculture applications.
The economic significance of genetically enhanced animals to U.S. animal agriculture in the future cannot be estimated with any confidence. However, the livestock and dairy industries within the U.S. generated over 87 billion dollars of on-farm receipts in 2008 (Anonymous, 2009). Therefore, even small increases in the efficiency of transgenic animal production would repay research costs several times over. This would be extremely impactful within the Western region as well as nationally, especially given the statistics for livestock production within the states comprising this regional research project (NASS, 2009). A total of 67.9 billion pounds of milk was produced in 2008. As of January 1, 2009, these states had an inventory of 42.93 million hogs, 28.61 million cattle and 2.06 million sheep, which included 1.7 million sows that farrowed, 11.67 million cows that calved and over 1.34 million breeding ewes on hand. In addition, a total of 15.58 million cattle, 91 million hogs and pigs, and 1.93 million sheep were marketed in 2008.
Construction of genetically enhanced animals for the production of food and fiber holds significant promise for consumers, animal producers, their communities and our environment. Conventional and somatic cell nuclear transfer (SCNT), or cloning, has dramatically advanced animal biotechnology, significantly enhancing our ability to produce genetically altered livestock. This technology has three broad applications: 1) applied animal breeding to propagate animals with superior pedigrees (e.g., proven bulls), 2) a tool for basic research, (e.g., mechanisms of differentiation), and 3) a biotechnological tool (e.g., much easier approaches to produce genetically enhanced farm animals). Although the breakthroughs in SCNT are noteworthy, this scientific landmark has been dwarfed by dramatic advancements in the field of genetic modification of animals. This has opened new avenues to produce livestock with improved carcass characteristics, that yield a leaner, more desirable meat, with increased disease resistance, and that are more efficient in growth, reproduction, and wool or milk production (Wheeler et al., 2003). Specific examples of genetically enhanced animals with application to the livestock industry include: 1) swine that produce omega-3 fatty acids in their meat, enhancing its health benefits (Prather, 2006); 2) dairy cows that produce disease resistant (i.e., mastitis) milk (Donovan et al., 2005); 3) sows that lactate milk containing bovine milk proteins, improving piglet growth and survival (Wheeler, 2003); and 4) males of livestock species that produce mono-sex sperm and therefore, sex-specific progeny (Forsberg, 2005). Increased efficiencies in production of animal foodstuffs can be of economic benefit to both consumers and producers. In addition, more efficient production of food and fiber has obvious advantages to the environment in terms of reduced use of natural resources. Consistent with this, investigators have developed swine that produce phytase, an enzyme that breaks down phosphorous, in their saliva, reducing emissions in manure that may be hazardous to the environment (Golovan et al., 2001). Finally, construction of genetically enhanced livestock animals for use in human biomedicine has also developed considerably. In addition to genetically enhanced animals that serve as models for human biology and disease, the potential for harvest of pharmaceutical proteins, antibodies and tissues/organs from these animals for specific medical applications is certainly impactful (Sullivan et al., 2008). Examples of genetically enhanced livestock with significance to human medicine include pigs modified to aid in transplantation of their organs into humans (Dai et al., 2002; Lai et al., 2002) and cattle that produce human antibodies (Keefer et al., 2007; Kuroiwa et al., 2002, 2004).
To offset the costs associated with these technologies, development of a nucleus herd of genetically enhanced livestock for implementation into production systems would need to offer a large potential benefit (Thomson and McWhir, 2004). However, it is not difficult to imagine a cryo-bank of cloned embryos with genetic enhancements available for direct purchase by producers. Even without implementation of genetic modifications, producers could significantly improve the average performance of their animals in a single generation, progress that is unmatched in traditional breeding programs (Thomson and McWhir, 2004). In 2006, the first genetically engineered animal product in the world was approved for commercialization in Europe (Sullivan et al., 2008). This product is utilized for the prophylaxis of venous thromboembolism in surgery of patients with congenital anti-thrombin deficiency. Last year, the Food and Drug Administration approved the release of cloned animals produced by standard NT methods (i.e., no gene targeting) to be marketed. One experiment station (IL) within the W-1171 Regional Research Project determined the absence of a transgene in control animals after co-habitation and post-mating with transgenic animals, providing a critical first step toward rigorous scientific data for risk assessment of transgenic livestock. Consistent with this, the legal and scientific mechanisms have recently been drafted to market a genetically enhanced livestock animal (M.B. Wheeler, personal communication), representing a significant advancement for the field of animal biotechnology. Therefore, translation of science performed by members of the Project to livestock producers is already in progress, marking a major impact of research performed by the W-1171 group.
Current procedures for the production of experimental animals with genetic enhancements can involve the use of in vitro oocyte maturation (IVM), in vitro fertilization (IVF), in vitro culture (IVC), cell culture and NT either before or after gene transfer (Wall, 2002). Combined, these technologies are inefficient, so before genetically enhanced animals can contribute significantly to livestock production systems, construction of these animals will have to be far more efficient. The inefficiencies occur at many levels including in vitro production (IVP) of embryos, nuclear transfer, and establishment of pregnancy. The use of in vitro-derived embryos is much more practical than recovery of in vivo-derived embryos, but IVM, IVF and IVC methodologies remain suboptimal. In the bovine system, blastocyst production by in vitro methods has plateaued at around 40 to 50% despite various attempts to improve culture conditions, falling short of the 85 to 95% development rate that occurs in vivo. Although we continue to make significant advances in NT technology for livestock and laboratory species (Niemann et al., 2003; Wang and Zhou, 2003; Mullins et al., 2004), much is still to be learned regarding the biology and application of these methods to produce genetically enhanced animals. This technology remains relatively inefficient at present (Niemann and Kues, 2000; Niemann and Rath, 2001; Paterson et al., 2003) and needs improvement before it can be widely used for livestock systems. Short and long-term storage of genetically altered embryos is necessary for efficient production of transgenic animals but cryopreservation of manipulated embryos needs to be investigated and improved as well (Hurtt et al., 1999; Dinnyes and Nedambale, 2009). Some inefficiency has been offset by the availability of viral delivery systems (i.e., retroviruses, adenoviruses and lentiviruses) for the production of genetically enhanced animals. However, these delivery systems only improve the efficiency of gene transfer prior to nuclear transplantation and have little impact on the inefficiencies associated with IVM, IVF and IVC procedures. Although, the use of RNA interference (RNAi), or small interfering RNA (siRNA), will likely provide an efficient alternative to determine the function of genes within large animal species, primarily due to the enhanced abilities of viruses to deliver and incorporate DNA into the genome (Thomson and McWhir, 2004). Finally, cloned fetuses often have difficulty developing to term, likely due to abnormal development of organ systems due to incomplete reprogramming of the donor nucleus. In addition to the inefficiencies associated with the production of genetically enhanced animals, the methodologies are highly time consuming and labor intensive. Up to 10 hours of labor may be required to produce a single cloned bovine embryo for transfer into a recipient female. When this is coupled with a 1-5% pregnancy rate, an estimated 1000 hours are required to produce a single transgenic offspring.
Investigation of challenging questions can be achieved very efficiently via a multistate research project of this nature. The combined expertise and resources of member scientists and institutions from both within the Western region as well as stations residing outside of the region can be utilized. Another advantage to the regional research model is that alternative approaches can be examined in multiple laboratories and the effective procedures further tested in the remaining laboratories. Oocyte and embryo procedures appear particularly laboratory dependent; for example, the optimal exposure time for vitrification of mouse oocytes and mouse blastocysts varied significantly among laboratories (Wood et al., 1993; Valdez et al., 1993; Zhu et al., 1993; Shaw et al., 1992). Examination of epigenetic alterations in NT-derived embryos compared to in vivo-derived embryos, improvements in NT methods and the development of embryonic/somatic cell lines to serve as nuclear donors are other areas that would benefit from this multiple laboratory approach. Isolation of pluripotent stem cells for agricultural species has been challenging and as yet, has not been fully successful. Sharing of information and approaches across this multi-state project is critical in advancing stem cell biology and its application to farm animals.
This renewal proposal will evaluate two critically important areas to the future success of animal biotechnology: 1) understand the biology and underlying mechanisms of gamete development, fertilization, and embryogenesis; and 2) refine methods for production of genetically enhanced animals to improve livestock production efficiency.
Related, Current and Previous Work
Support for the collaborative W-1171 Regional Research Project over the past five years has led to a number of accomplishments, significantly impacting the scientific field and ultimately, the livestock industry within the Western states. In point of fact, the Research Project has produced a significant number of publications (687) between Jan. 1, 2005 and December 31, 2008: 260 refereed journal articles; 112 books, book chapters, proceedings, instructional media, and theses/dissertations; 282 abstracts and 33 semi-technical/lay publications (see Publications attachment). Combined with the publications expected in the final year (2009) of the project, it is apparent that the Project has been highly productive and outcomes of these collaborative efforts have been disseminated via a variety of written avenues.
Although the accomplishments of the Project have been extensive (see Additional Accomplishments/Impacts attachment), the major accomplishments and areas of research needing further investigation, organized by objectives, are described below.
1) Understand the biology and underlying mechanisms of gamete development, fertilization and embryogenesis.
Oocyte Maturation - Use of IVM oocytes in combination with IVF for the production of livestock embryos has become commonplace (Nagai, 2001; Lonergan et al., 2003; Miyano, 2003; Nagai et al., 2006). These techniques allow the study of basic biological events that occur during oocyte maturation, fertilization and early embryonic development as well as provide an inexpensive and readily procurable source of preimplantation livestock embryos. Successful maturation, fertilization and subsequent embryonic development depend heavily on initial oocyte quality. Determinants of oocyte viability and its likelihood to fertilize and develop as an embryo following IVM would dramatically improve the efficiency of IVP. Alternatively, reliable methods for assessment of the developmental competence of in vivo- or in vitro-derived embryos would permit screening of embryos prior to manipulation or transfer procedures. Linking these indicators of embryo viability and successful development with the expression and function of specific genes could also afford valuable insights into mechanisms contributing to embryonic mortality.
Successful oocyte maturation is dependent on two events, nuclear and cytoplasmic maturation (Fulka et al., 1998). Within the oocyte, levels of cAMP (Conti et al., 2002; Eppig et al., 2004) and a Gs-coupled receptor (GPR3; Mehlmann et al., 2004; Mehlmann, 2005) regulate meiotic arrest. Characteristics of the meiotic spindle can be used to evaluate oocyte quality (Moon et al., 2003; Rienzi et al., 2003). However, the process of nuclear maturation occurs spontaneously upon removal of the cumulus-oocyte complex (COC) from the follicle and does not represent a significant problem using established culture conditions. In contrast, the process of cytoplasmic maturation appears to be the critical factor that determines the success of subsequent embryo development. Determining the indicators of cytoplasmic maturation in the oocyte would enable more efficient selection of oocytes for IVF and IVP. Currently, there is no defined method of measuring cytoplasmic maturation other than successful fertilization and embryo development (Krisher, 2004). Although much more research is required, several cellular and molecular predictors of oocyte quality show promise (Coticchio et al. 2004; Combelles and Racowsky 2005; Wang and Qing-Yuan, 2007) including inadequate distribution of mitochondria (Au et al., 2005; Brevini et al., 2005), mitochondrial DNA deletions (Hsieh et al., 2002; Gibson et al., 2005) and reduced glucose-6-phophate dehydrogenase levels (Alm et al., 2005).
Methods to determine the developmental competence of oocytes has been a strong area of interdisciplinary investigation within the Project (CA, CO, CT, IL, IN, LA, MS, NC, NE, WV, UT). Multiple stations have evaluated gene expression profiles during oocyte maturation (CO, CT, IL, IN, NC, WV). Aberrant metabolism, incorrect mRNA post-transcriptional processing and increased apoptosis were shown to be some of the mechanisms contributing to reduced developmental potential of gilt vs. sow oocytes. Gene expression was altered in IVM compared to in vivo matured oocytes. Improper regulation of gene expression in the oocyte resulting in altered DNA methylation and up-regulation of cell death pathways are potential developmental mechanisms influenced by IVM conditions, correlating with reduced embryonic developmental potential. A method to study how bovine oocytes modify mRNA during maturation, mostly by selective degradation of specific mRNAs (GDF9 and cyclin), was validated. The presence of a novel importin alpha and SPP1 mRNAs were identified in oocytes. A functional role for Nr4A1 mRNA in FSH-induced initiation of germinal vesicle breakdown during IVM was determined. Finally, sperm receptors on the oocyte plasma membrane were confirmed as biomarkers of oocyte quality. Other stations (LA, IL, MS, NE, UT) evaluated methods to improve IVM culture conditions, as well as determining the effects of leptin, glucose, osteopontin, ammonium, biotin and nicotine on oocyte maturation.
Fertilization - Successful fertilization in mammals is dependant on a number of factors including meiotic/morphological changes during spermatogenesis, acquisition of epidydymal/seminal plasma proteins, attainment of motility, capacitation, binding of acrosome-intact sperm to the zona pellucida of the oocyte, acrosome reaction, fusion of the acrosome-reacted sperm with the oocyte plasma membrane, and oocyte activation (for review, see Sutovsky, 2009; Wassarman, 2009). Although the use of IVF to produce farm animal embryos has become routine, subsequent embryonic development in vitro remains far below that of in vivo embryos, suggesting that more research is required to optimize these factors. Some investigators have focused on these factors (AR, CA, CO, LA, MS, NC, UT). In regard to sperm production, Project members have determined that: method of motile sperm selection can influence parameters related to motility and head morphology; grazing bulls on endophyte infected fescue reduced sperm motility and morphology; ejaculated equine spermatozoa contained measurable amounts of mRNAs for genes associated with testicular spermatogenesis; sperm protein-22 was specifically localized in equine spermatozoa and expression was altered with season of collection; and a low sodium medium was a viable alternative to synthetic caudal epididymal fluid medium for maintaining sperm viability during storage. Another station has provided functional genomic blue prints of bovine spermatozoa. Despite some progress in identification of spermatozoa proteins playing a critical role in fertilization, more research is required to determine the underlying functional mechanisms associated with these proteins (Peddinti et al., 2008). As the oocyte matures, the protein composition of the plasma membrane changes including an increased ability to bind and/or fuse with sperm. Integrins located on the plasma membrane of bovine oocytes have been implicated in both fertilization and oocyte activation (Pate et al., 2007). One station linked the alphaV and beta1 subunits of integrins, focal adhesion kinase and Src family kinases to fertilization and activation of bovine oocytes. Also, injection of inositol triphosphate at the time of ICSI of bovine oocytes resulted in improved cleavage rates.
Embryo Development - Early embryogenesis is regulated by developmentally controlled events including proper changes in transcriptional machinery and activation of embryonic genes to support further development (Misirlioglu et al., 2006; Kues et al., 2008). It is important to improve IVC systems in order to produce embryos with high developmental competence for use in agricultural and biomedical research, as well as animal biotechnology (Hansen and Block, 2004). Although the methodology for maintaining embryos from livestock in culture has existed for many years, the ability of the present systems to support normal development is limited. Studies have been performed to remove, replace or delay the addition of serum into the culture systems (Wirtu et al., 2004). However, the results indicated that removal of serum from the culture medium does not always prevent the occurrence of large offspring syndrome (LOS; Rizos et al., 2003), suggesting other causes of this severe side effect of IVP. A number of different types of semi-defined and defined media have been designed utilizing a variety of supplements (for review, see Vanroose et al., 2001; Abe and Hoshi, 2003; Palasz et al., 2006; Harvey et al., 2007). The primary problem associated with current culture systems is that they do not mimic the changing oviductal/uterine environment. The apparent lack of biologic culture conditions necessitates further innovation in tissue culture methodology and the pursuance of further research in this area. One station (IL) developed miniaturization technologies, toward microfluidics and small mechanical systems, creating a means for dynamic culture on a volumetric scale and more consistent with the needs of the embryo.
Epigenetics, including DNA methylation and histone modifications (methylation, phosphorylation and acetylation), has become a primary area of focus during early embryonic development (Jenuwein et al., 2001; Brown et al., 2002). Shortly after fertilization, mammalian embryos undergo genome-wide epigenetic reprogramming by demethylation, followed later by de novo remethylation (Dean et al., 2001). This epigenetic reprogramming is essential for normal development to proceed. Toward this end, aberrant reprogramming has been clearly linked to failed embryonic development (Dean et al., 2001; Shi et al., 2002; Rodriguez-Osorio et al., 2009). Moreover, inadequate epigenetic reprogramming during early mammalian development may result in LOS and other developmental abnormalities (Hill et al., 2000a,b; Brown et al., 2002). Thus, understanding the factors and mechanisms which control epigenetic reprogramming during early mammalian development is of critical importance.
Multiple stations investigated the cellular, genomic and epigenetic aspects of early embryonic development (CO, CT, IA, IL, LA, MD, MS, NC, NE, OR, SC, WA, WV). Periattachment factor mRNA increased dramatically in elongating ovine embryos from day 11 to 16 and subsequently declined by day 30. Two novel Lef1 isoforms expressed in bovine preimplantation embryos were identified. Bovine Air ncRNA was expressed in fetal tissue at the post- and peri-implantation stages but not prior to implantation. A new member of the importin alpha gene family has a potential role in transporting oocyte-specific nuclear proteins and is required during early embryogenesis. Additionally, melatonin and GnRH were found to be important to development of early embryos. The effects of different culture systems and protein sources on Oct4, NANOG, connexin 43, and GLUT1 transcripts were also examined. Further, expression patterns of the chromatin remodeling genes, HMGN3A and SMARCAL1, were determined.
2) Refine methods for production of genetically enhanced animals to improve livestock production efficiency.
Nuclear Transfer - With significant advancements in the area of SCNT (Cibelli, 2007), many experimental stations have evaluated the procedures and underlying biology associated with SCNT (CT, IL, LA, MS, NC, UT). Although scientists can successfully reprogram somatic cells prior to NT and produce live animals (UT-mules; NC-Yucatan swine), many questions remain regarding the inefficiency of the process, the impact of SCNT on the health of resultant clones, and the molecular and cellular effects reprogramming has on somatic cells subsequently used as karyoplasts in SCNT. In NT, the somatic nucleus has to be reprogrammed in order to restart and continue the developmental process. The somatic donor cell inactivates its tissue specific genes and then re-activates embryo specific genes necessary for normal embryo development (Han et al., 2003; Rodriguez-Osorio et al., 2009). The factors affecting the efficiency of NT are: enucleation of the recipient oocyte, fusion of the transplanted nucleus to the enucleated oocyte cytosol, activation of the oocyte, and "re-programming" of the transferred nucleus (Beyhan et al., 2007a,b). Surprisingly, there is evidence of significant reprogramming in cloned embryos compared to their IVF counterparts (Smith et al., 2005; Beyhan et al., 2007a,b; Rodriguez-Osorio et al., 2009). However, evidence seems to suggest that most of the incomplete reprogramming is related to epigenetics (Rodriguez-Osorio et al., 2009), an area of research that has moved to the forefront of science.
Aberrant patterns of DNA methylation, one of the major epigenetic modifications of the genome, have been proposed as a contributing factor in the poor development of embryos following SCNT. Using a bovine model, examination of the methylation pattern of genes involved in reprogramming of somatic cells to a pluripotent state revealed that the methylation patterns of SCNT blastocysts resembled the fibroblast donor cell line rather than IVF control embryos (UT). Another station (LA) determined levels of DNA methylation, histone acetylation and gene expression patterns of the chromatin remodeling proteins, Dnmt1, HDAC1 and MeCP2 in bovine fibroblast cells at different population doublings (PD). Levels of acetylated histone H3 were constant, whereas methylated DNA increased progressively from PD 2 to 45. Compared with cells at PD 2, Dnmt1 gene expression levels decreased by approximately 50% at PD 7 and remained low during culture. In contrast, HDAC1 and MeCP2 mRNA levels decreased significantly after PD 2 (LA). In SCNT-derived pigs, incomplete epigenetic reprogramming of donor cells has been associated with phenotypical abnormalities including dysregulated gene expression in the lungs. A comparison of global and loci-specific methylation of cytosine in the lungs of deceased neonatal and one-month-old live cloned pigs to those of age-matched controls demonstrated that global cytosine methylation is high in pigs and differing levels of methylation in cloned pigs may be associated with improper regulation of global gene expression (CT). Another station (MS) identified potential cumulative errors that could reveal epigenetic blocks in animal cloning by comparing the transcriptome of blastocysts derived from serial bovine NT (1-4 generations) to blastocysts produced by standard IVF procedures. Irregular patterns of gene expression were evident for the cloned blastocysts; genes involved in regulation of the cytoskeleton and cell shape were upregulated in cloned embryos whereas genes associated with chromatin remodeling and coping with stress were increased in standard IVF embryos (MS).
The general consensus of those in the field is that nuclear transfer efficiency is much too low (< 10%) to be economically viable except in very limited applications. Any increase in efficiency will greatly enhance the value of this technology and contribute to our understanding of changes that must occur in chromosomes to allow appropriate embryonic gene expression patterns. It is also critical to understand how potentially subtle modifications in the nuclear structure impact the ability of a cell to contribute to production of offspring. Despite the challenges, nuclear transfer offers unique opportunities in both agriculture and biomedical fields as well as in basic research (Wilmut et al., 2009; Kuroiwa et al., 2009).
Sex Determination - A number of stations have explored methods/impacts of sex selection (AR, CO, IL, LA, SC). The ability to pre-select the sex of offspring would have tremendous economic benefit to livestock producers. One approach has been to physically separate X- from Y-bearing spermatozoa (Amann, 1989). To date, modified flow cytometry (Johnson et al., 1994) is the only separation procedure that has been successful in sorting X- from Y-bearing spermatozoa. Although new methods are being explored, flow cytometry remains the most efficient method to sort semen. While flow cytometry has been improved as evidenced by success with frozen-thawed, sex-sorted and refrozen-thawed ram spermatozoa (de Graaf et al., 2007), the high cost of equipment and complexity of the procedure remains a limitation to widespread application.
Cryopreservation - Cryopreservation of in vivo produced bovine embryos is quite successful and success has been achieved with other species including late-stage porcine (Berthelot et al., 2000), goat (Hong et al., 2007), and equine (Carnevale, 2006) embryos. Alterations in IVM and IVC can affect the susceptibility of in vitro-produced embryos to cryo-damage. With increased utilization of embryo manipulation procedures, it is critical that we gain a better understanding of the impact these technologies have on successful cryopreservation and develop strategies to prevent the occurrence of detrimental alterations to embryos exposed to manipulation (Dinnyes and Nedambale, 2009). One station (CO) made in vitro-produced embryos more like in vivo-derived embryos, with respect to lipid content, by treating with forskolin. Reduction of the cytoplasmic lipid content of bovine embryos with phenazine ethosulfate (PES) improved cyrotolerance (Seidel, 2006). Furthermore, recent advances in vitrification have allowed for successful cryopreservation of goat (Hong et al., 2007), porcine (Somfai et al., 2008), equine (Oberstein et al., 2001), ovine (Dattena et al., 2000) and bovine embryos (Massip, 2001). One station (CO) developed a fairly efficacious method of freezing small equine embryos that appeared superior to a standard vitrification procedure. Another station developed a successful procedure for cryopreservation of porcine embryos using a microdroplet procedure and established divergent survival rates, within the first 24 h following thawing, for cryopreserved embryos from Chinese Meishan and white crossbred lines of swine (NE). More recently, scientists have focused on methods for cryopreservation of oocytes, which has presented many new challenges. Successful vitrification of porcine (Liu et al., 2008), equine and bovine oocytes (Hurtt et al., 1999) has been achieved, although mature oocytes survive vitrification better than immature oocytes. Other stations have investigated strategies to improve oocyte freezing survival (CO, CT, LA).
Stem Cell Biology - Despite the historic success with the isolation and subsequent gene targeting of mouse ES cells, isolation of pluripotent stem cells for agricultural species has been extremely challenging. This lack of success has been offset by dramatic improvements in SCNT, potentially bypassing the need for ES cells. However, as described in the Nuclear Transfer subsection, many difficulties associated with SCNT remain to be solved, especially the inefficiency of the process. Another concern with SCNT is the ability to maintain somatic cells in culture long enough for successful gene targeting and subsequent selection (Denning and Priddle, 2003). Multiple laboratories have characterized ES-like or EG (derived from primordial germ) cells for livestock animals (for reviews, see Prelle et al., 2002; Wheeler and Malusky, 2003; Thomson and McWhir, 2004; Gjorret and Maddox-Hyttel, 2005), however, germline chimaerism has not been established (Wells et al., 2003). More recently, investigators have focused on the isolation of stem cells from a multitude of tissue sources such as adipose and bone marrow (Kues et al., 2005; Trounson, 2005; Oback, 2009).
Some stations (MD, OR, WA) examined how embryonic cells begin to differentiate. While selective ablation of differentiating cells in ICM explant culture was not sufficient for short-term pluripotent cell survival, supplementation with the cytokine, Noggin, prolonged proliferation of undifferentiated cells as well as upregulated expression of the pluripotency-related transcription factor, NANOG. The role of urokinase-type plasminogen activator on outgrowth of endodermal cells from bovine ICM was evaluated. Production of trophoblastic vesicles from dispensed embryo cells represented a model to study cardiac organ formation and a source for cardiac stem cells. Several other stations have isolated stem cell cultures from a variety of non-embryonic tissues/cells (GA, IL, LA, MD, NC). Neural, amniotic, mesenchymal, and adipose-derived stem cells as well as induced pluripotent stem (iPS) cells have been isolated and/or investigated. Adipose-derived stem cells (ADSCs) and mesenchymal stem cells (MSCs) had very similar transcriptomes before differentiation, including expression of two osteogenic genes most abundant in MSCs (COL1A1 and SPARC). However, several genes, unique to each type of differentiation, were revealed including SPP1 and BGLAP. Interestingly, adipogenic differentiation was distinguished by genes involved in triacylglycerol transport and storage but not de novo synthesis. In addition, ES cells can differentiate into germ cells, representing an important germ cell source for production of genetically enhanced animals (West et al., 2008). Another station has developed a novel differentiation culture system that is capable of producing large numbers of germ-like cells that undergo advanced stages of development from ES cells.
Related Multistate Projects
A CRIS search with the major areas of research under the objectives of this Project (oocyte maturation, fertilization, embryo development, nuclear transfer, sex determination, cryopreservation and stem cell biology) revealed some related regional research projects. These projects included: NCERA-057, Swine Reproductive Physiology; NE-1027, Ovarian Influences on Embryonic Survival in Ruminants; W-1112, Reproductive Performance in Domestic Ruminants; S-1020, Enhancing Reproductive Efficiency of Poultry; NC-1170, Advanced Technologies for the Genetic Improvement of Poultry; NC-1010, Interpreting Cattle Genomic Data: Biology, Applications and Outreach; S-1031, Improvement and Sustainability of Channel-Blue Hybrid Catfish Embryo Production and Performance; NC-1031, Nanotechnology and Biosensors; and NC-1131, Molecular Mechanisms Regulating Skeletal Muscle Growth and Differentation. Most of these projects are only slightly related to W-1171, as they are investigating alternative animal species (poultry and catfish), biological systems (skeletal muscle) or related technologies (nanotechnology, biosensors and genomic data analysis). The multistate projects examining aspects of reproductive physiology (NCERA-057, NE-1027 and W-1112) are most closely related, however, the work of these groups is complementary rather than duplicative. For example, one objective of NE-1027 is to identify genetic, morphological and physiological attributes of the ovary considered to improve fertility in ruminants. Although members of W-1171 are investigating aspects of the ovary, they are specifically focused on oogenesis as it relates to increasing the efficiency of embryo production for genetic manipulation technologies.
Objectives
-
Understand the biology and underlying mechanisms of gamete development, fertilization and embryogenesis.
-
Refine methods for production of genetically enhanced animals to improve livestock production efficiency.
Methods
Objective 1: Understand the biology and underlying mechanisms of gamete development, fertilization and embryogenesis. The overall aim of the research to be conducted under this objective is to gain a better understanding of the biological requirements for successful oocyte maturation, fertilization, and subsequent embryonic development. The production of live offspring is dependant upon all of these events occurring in a well-orchestrated fashion. More importantly, perhaps, is the fact that a better understanding of the mechanisms underlying these biological processes (i.e., epigenetics) will lead to the production of more healthy, viable offspring. This, in turn, will improve many of the in vitro systems even further, advancing them yet closer to conditions present in vivo. A number of member experiment stations will be investigating this objective including: AR, CA, CT, CO, IL, IN, LA, MD, MS, NC, NE, NV, OR, SC, UT, WV. Oocyte Maturation and Developmental Competence - This area of investigation will represent a strong collaborative subgroup of this multistate research project (CA, CT, CO, IL, LA, MS, NC, NE, NV, WV) whose goal will be to investigate the factors associated with development of the oocyte. The efficiency of IVM systems is still sub-optimal. A better understanding of the molecular and cellular mechanisms underlying oocyte maturation will provide information that will help increase the efficiency of IVM procedures. One focus area of the group will be regulation of nuclear and cytoplasmic maturation in domestic species. Although questions regarding the molecular mechanisms underlying nuclear maturation remain, nuclear maturation does not present a significant problem with current IVM culture systems. However, there is no defined method of measuring cytoplasmic maturation other than successful fertilization and embryo development (Krisher, 2004). This group will evaluate changes that occur during cytoplasmic maturation using standard IVM protocols for respective livestock species in an attempt to isolate specific predictive indicators of mature oocyte cytoplasm. Similarly, a better understanding of oocyte maturation will lead to the isolation of biomarkers for the ability of the oocyte to fertilize and develop as an embryo, or developmental competence. Although intensively investigated, there is a gap in knowledge regarding markers of developmental competence. Researchers will identify markers for developmental competence of oocytes in livestock species by: 1) comparing IVM-derived vs. in vivo-derived oocytes; 2) examination of oocytes from more compared to less mature females (i.e., sow vs. gilts or pubertal vs. pre-pubertal); 3) determining differences between oocytes exposed to hormones/growth factors/supplements known to enhance development either in vivo or in vitro in comparison with controls; and 4) utilizing genetic strains of animals that are divergent for quality and/or rates of oocyte/embryo development. The search for markers of developmental competence will utilize both functional genomic (microarray), proteomic (immunofluorescence, immunocytochemistry, stains/dyes for cellular organelles and viability, Western blots, protein arrays) and other molecular (chromatin immunoprecipitation and siRNA protocols) approaches. In addition, new generation sequencing procedures (i.e., Illumina sequencing technology) which allow high-throughput analysis of the whole genome will be employed. This technology will allow for easier identification of genetic markers, or small nucleotide polymorphisms (SNPs), that might be linked to developmental competence of the oocyte. Sperm Physiology - This workgroup (AR, CA, CO, LA, MS, SC) will evaluate the biology associated with meiotic and morphological changes that occur during spermatogenesis, leading to the production of fertile sperm. Certainly, this area of research is critical to improving the efficiency of IVF procedures and subsequent embryonic development. Bulls will be evaluated for fertility measures including CASA analysis of semen samples and standard staining protocols. Similar to the Oocyte Maturation and Developmental Competence group, this subgroup will utilize functional genomics approaches to identify markers of fertility in bulls and implement these into functional genetic tests to predict fertility/infertility in males. Genetic markers will be correlated to measures of fertility from the same animals, as well as with cleavage rates and embryo development after fertilization of oocytes using IVF, intracytoplasmic sperm injection (ICSI) or natural fertilization. Fertilization Mechanisms - The cellular and molecular aspects of fertilization and oocyte activation will be explored by this group (CA, CO, MS, NC, NV, UT, WV). One mechanism identified by this group for further investigation is the regulation of polyspermy. Although its importance may vary between species, polyspermy remains a significant difficulty in successful porcine IVF procedures. Researchers will try to isolate sperm/oocyte factors as well as conditions that may contribute to polyspermic conditions. Similarly, the group is also interested in examining basic mechanisms of fertilization in domestic livestock species. Despite some progress in identification of spermatozoa proteins playing a critical role in fertilization, more research is required to determine the underlying functional mechanisms associated with these proteins (Peddinti et al., 2008). Collaborative efforts of the group will continue to isolate fertilization-specific proteins, including analysis of sperm protein-22 in equine spermatozoa, using proteomic approaches. As the oocyte matures, the plasma membrane increasingly gains the ability to bind and/or fuse with sperm. Integrins located on the plasma membrane of bovine oocytes have been implicated in both fertilization and oocyte activation (Pate et al., 2007). Two stations (CO, UT) linked the ±V and ²1 subunits of integrins, focal adhesion kinase and Src family kinases, as well as IP3, to fertilization and activation of bovine oocytes. The group will investigate downstream intracellular signaling intermediates specific to these cellular pathways that are activated within the oocyte during fertilization. In the pig, downstream pathways associated with two putative oocyte membrane receptors for sperm, shown to be a markers of oocyte fertilizability and reduced by heat stress, will be examined utilizing established protocols that incorporate specific antibodies directed against these to factors. Early Embryonic Development - Another large collaborative workgroup (CO, CT, IL, IN, MD, NC, NE, OR, WV, UT), these scientists will investigate factors underlying the early stages of embryonic development. One major area of collaboration will be to optimize methods for performing microarray analysis on embryos at early stages of development as well as analysis of single embryos from mice, cattle and pigs. The CT station will provide vast expertise with microarray analysis of embryos. Other stations will collect embryos from specific experimental designs to isolate factors vital to these early developmental pathways and send them to CT for microarray studies and subsequent statistical analyses. Specifically, some investigators will focus on genetic regulation of the maternal to zygotic transition in livestock species. Identification of factors influencing this critical step in preimplantation development will greatly enhance our ability to optimize IVC systems, enhancing the competence of embryos. Shortly after fertilization, mammalian embryos undergo genome-wide epigenetic reprogramming by demethylation, followed later by de novo remethylation (Dean et al., 2001). Of primary interest, the effects of epigenetics at this extremely important stage of development will be targeted. Additional studies will pursue peri- and post-implantation development, including genetic regulation of conceptus and placental development. In Vitro Embryo Manipulation - This workgroup (CO, CT, LA, NC, UT) will examine the effects of in vitro manipulation procedures on both peri- and post-implantation development, especially the effect of embryo manipulation on gene expression during development. As suggested below, embryos that are manipulated in vitro are much more difficult to freeze than in vivo-derived embryos. In a similar vein, this group will determine the impacts of embryo manipulation on global gene expression patterns and epigenetics as these embryos continue development leading up to, during and after implantation, including effects during fetal development (i.e., intrauterine growth restriction). Objective 2: Refine methods for production of genetically enhanced animals to improve livestock production efficiency. The overall aim of the research to be conducted under this objective is to enhance the success of each step in the embryo micromanipulation process that is required for successful production of genetically enhanced livestock animals. Continual advances in gene transfer techniques and NT methodology have led to a crucial need for more research that will lead to increased incorporation of these methods into livestock production systems. Research under Objective 2 will be conducted by multiple research institutions comprising the Project: AR, CO, CT, GA, IA, IL, IN, LA, MD, MS, NC, NE, OR, UT, WA. Transgenic Animals - This workgroup (CT, IL, LA, MD, NE) will work together toward the production of transgenic animals with agricultural applications, exploiting siRNA gene knock-down technologies. Investigators at NE are studying a recently idenfied form of the gonadotropin-releasing hormone (GnRH) receptor (GnRHR), termed the GnRHR II. This 5- and/or 7-transmembrane receptor is expressed ubiquitously and likely has a strikingly different function than the historic GnRHR, now termed GnRHR I, whose primary functional site is gonadotrope cells within the anterior pituitary gland. Uniquely, the GnRHR II gene is absent in mice and rats; therefore, the pig represents a primary model to determine the in vivo function of this novel G-protein-coupled receptor. Various siRNA oligonucleotides will be tested in a swine testis cell line to determine the siRNA with the strongest ability to reduce the production of GnRHR II. Next, lentiviral vectors will be constructed that contain a constitutively active promoter fused to the selected siRNA and viral particles will produced. Viral particles will be sent to stations with micromanipulation expertise (CT, IL, LA, MD) for injection of particles within the perivitelline space. Embryos will either be transferred into recipient females or rapidly returned to the NE station for subsequent transfer into surrogate mothers. We anticipate that a collaborative experiment of this nature could eventually lead to a team of highly trained scientists with expertise in the production of siRNA knock-down livestock animals. Both anticipated increases in efficiency of gene delivery and simpler microinjection procedures will likely lead to accelerated use of this technology. This group will also pursue the production transgenics with his-tag constructs, development of a mesenchymal stem cell knock-out animal, and production of genetically enhanced animals that would address environmental concerns such as CO2 fixation in ruminants. Nuclear Transfer - This subgroup of the Project (CT, IL, IN, LA, MD, MS, NC, UT) will focus on the procedures and underlying biology associated with significant advancements in the area of SCNT. Although scientists can successfully reprogram somatic cells prior to NT and produce live animals, there is a gap in knowledge regarding the inefficiency of the SCNT process (<10%), especially with respect to genetic modification. Aberrant patterns of DNA methylation, one of the major epigenetic modifications of the genome, have been proposed as a contributing factor in the poor development of embryos following SCNT. Certainly, epigenetic influences on progression of SCNT embryos will be further investigated as this area has moved to the forefront of science. Another concern with SCNT is the ability to maintain somatic cells in culture long enough for successful gene targeting and subsequent selection (Denning and Priddle, 2003). This group will evaluate culture conditions in an attempt to enhance the number of cloned embryos that develop to term, including addressing why somatic cell cultures often phase out by 25 to 28 passages. Next, the group realizes the importance of developing cells from livestock species to compare to cell lines in mice that develop into multiple tissue types. Finally, many developmental abnormalities are associated with SCNT procedures and systems for optimal survival post-transfer remain to be developed. This group will attempt to identify why gene expression patterns and morphology are abnormal in clones. One methodology will be to consider species differences. The developmental potential of hybrid NT embryos, such as sheep-cow, to produce viable offspring will be studied. Another approach is to evaluate the time NT embryo clones spend in vitro prior to being placed into an optimal in vivo environment. Would some abnormalities associated with bovine SCNT be overcome if cloned embryos were transferred earlier (i.e., surgically into the oviduct) rather than non-surgical transfer into the uterus after 5 to 6 days of culture? Another methodology will be to tag potentially important factors in somatic cells with fluorescent proteins and determine if these factors become reprogrammed following SCNT. Gamete and Embryo Cryopreservation - This subgroup of the Project (AR, CO, CT, IA, LA, NE) will develop methodologies for improvements in cryopreservation of sperm, oocytes and embryos, as well as gain a better understanding of the molecular and cellular processes influenced by freezing and thawing procedures. Investigators have recently focused on developing methods for cryopreservation of oocytes, which has presented many new challenges. Successful vitrification of porcine (Liu et al., 2008), equine and bovine oocytes (Hurtt et al., 1999) has been achieved, although the efficiency and success of these cryopreservation technologies remain low. The group will build on these successes to further enhance the efficiency of oocyte cryopreservation. Although the metaphase II spindle can be preserved during the slow freezing process, it is gradually disassembled during thawing of oocytes. Efforts will be concentrated on identifying cryoprotectants/proteins and cellular components that prevent disassembly of the metaphase II spindle during thawing. Oocytes will be fixed before, during and after cryopreservation and examined by immunocytochemistry and confocal microscopy. Further, this group will analyze differences in mammalian sperm cryopreservation. Male to male variation in the ability of sperm to survive the freezing and thawing process is significant and more investigation is required to identify the factors contributing to these differences in fertility. These researchers will cryopreserve sperm from males of different species/breeds with divergent fertility using established protocols and identify differences at the genomic, cellular and protein levels following the thawing process. Studies will not be limited to sperm obtained from standard collection methods but epididymal reservoirs of sperm will also be evaluated, including those of livestock animals that are injured or post-mortem. Finally, the group will continue to advance their knowledge base in regard to cryopreservation of embryos. Efficient production of genetically enhanced animals depends on successful protocols for short and long-term storage of manipulated embryos (Dinnyes and Nedambale, 2009). Consistent with this, cryopreservation of manipulated and/or IVF embryos need to be investigated and improved. The group will examine the effects of various manipulation methods (IVF, IVC, microinjection, assisted hatching) on the viability of embryos following freezing and thawing compared to standard in vivo-derived embryos. Culture conditions will be critically examined, alternative cryoprotectants will be analyzed, molecular and cellular mechanisms will be compared and new protocols identified to make manipulated embryos more similar to in vivo-derived controls. In addition, new techniques for freezing smaller equine embryos (<300 ¼M in diameter) by vitrification and slow cooling have been developed. The large equine embryo routinely collected non-surgically is particularly challenging to cryopreserve. This group will further evaluate and optimize these promising protocols. In addition, these techniques will be evaluated with embryos from other species. Another area of focus will be to determine why embryos from different genetic lines have divergent survival rates following vitrification and thawing. Following standard vitrification and thawing protocols, embryos from genetic lines with divergent survival rates will be examined to determine gene expression profiles, differences in proteins/factors associated with plasma membrane integrity, timing of detrimental effects following freezing/thawing, and whether alternative cell signaling/apoptotic pathways are activated/inhibited. Stem Cells This group of investigators (CT, GA, IL, MD, NC, OR, UT, WA) will study biological mechanisms and procedures involved in isolation of both ES cells as well as tissue-specific stem cells. Our understanding of cellular differentiation has greatly expanded, largely due to the dramatic advances in SCNT methodologies. However, one major limitation to the livestock industry is the absence of ES cells, largely due to inappropriate culture conditions to maintain these undifferentiated cells appropriately. Isolation of ES cells in livestock species will continue to be of upmost importance to this scientific group. Procedures for isolation of mouse ES cells are well established and have been attempted with ES-like cells from livestock species. The group will focus on development of new, unique methods that might lead to an improvement in isolation of these cells in livestock animals. As an alternative to ES cells, much research expertise has been dedicated to the isolation of tissue-specific stem cells. This group has already isolated neural, amniotic, mesenchymal, and adipose-derived stem cells as well as pursued induced pluripotent stem (iPS) cells for livestock species. Member stations will collaborate on studies with mesenchymal and adipose-derived stem cells, focusing on epigenetic modification and trans-differentiation of these cells. Another station has developed a novel differentiation culture system that is capable of producing large numbers of germ-like cells that undergo advanced stages of development from ES cells. The group envisions major impacts of this system on the successful outcomes of NT as well as providing a source for renewal of gametes. Finally, this research collaboration will continue to explore the mechanisms underlying differentiation with particular attention to changes that occur upon formation of the ICM in blastocyst stage embryos. Collaboration between Member Stations Members of the Project meet annually to present/discuss research results, develop/critique experimental designs for proposed collaborative research projects, determine industry needs, and evaluate newly established technologies. This is characteristic of the original goal established by the founding members of the W-171 Regional Research Project twenty years ago. The overall goal of the Project founders was the development of a cooperative, multistate research group comprised of basic and applied scientists to investigate the biological mechanisms underlying germ cell and embryonic development so that these processes could be manipulated for the improvement of livestock. The production of a bound formal report comprised of experimental station reports, minutes of the previous meeting and contact information for station representatives is considered a real positive for the Project. Sharing of resources, protocols and technology is a key advantage of multistate research projects. We will collaborate through sharing resources, protocols and technologies developed at member stations such as DNA constructs, tissue-specific DNA, RNA and protein, oligonucleotide probes, antibodies, cell lines, culture media, cryopreservation protocols, and microfluidic/nanoscale embryo culture chambers. In addition, oocytes, sperm, embryos, genetically transformed somatic cells, or stem cells from various tissue sources can be shared between institutions. This has allowed our member stations to develop strong scientific collaborations. For instance, micromanipulation for the production of cloned embryos will occur at stations with that expertise (CO, CT, IL, LA, NC, UT). Cloned embryos can then be sent to other stations for cryopreservation via different methodologies and subsequent analysis of developmental competence following thawing of those embryos (IA, NE, NV, WV). Similarly, these same cloned embryos can be sent to institutions with more advanced analysis technologies and/or core facilities with experts in the field of bioinformatics to statistically analyze high-throughput genomic, epigenetic and proteomic assays. Additionally, stations from states with larger livestock industries (AR, CO, IA, IL, NE, NC) will isolate gametes and/or embryos and ship the material to states with reduced animal production numbers or laboratories lacking access to livestock handling facilities (CT, MD, NV, OR, WA, WV). Combined, the representative scientists from each experimental station embody a wealth of knowledge and experience regarding experimentation with gamete development, fertilization and embryogenesis, as well as technologies for the production of genetically-enhanced livestock animals. The numerous communications (i.e., phone calls, emails) that occur between station investigators outside of the annual and other national meetings really epitomizes the collaborative, multistate approach to research. In addition to these valuable features of exchange within the Project, the often overshadowed educational aspects remain important to collaborative efforts. A considerable amount of mentoring occurs within the project, at both the faculty and graduate student levels, all taking an active role in helping one another succeed.Measurement of Progress and Results
Outputs
- The principal output of this regional research project will be publications. A total of 687 publications over the first 4 years of the previous project is indicative of the potential of this group. These articles will largely impact the scientific field, advancing the areas of gamete and embryo development as well as production of genetically enhanced livestock. However, lay publications associated with these scientific discoveries will impact livestock producers across the Western region, the U.S. and the world.
- An output of the previous project period was a joint grant proposal focused on sperm sexing applications in the cattle industry was submitted to USDA. Members of the Project served as Co-Principal Investigators and collaborated extensively during the preparation of the proposal. Although the proposal was not funded, the process did serve to establish collaborative research projects among stations. We hope to submit similar proposals in the future.
- With advances in the efficiency of transgenic animal production and approved mechanisms/regulations for production of genetically enhanced livestock animals, we expect these animals to make their way to production systems. This will allow for dramatic improvements in the efficiency of livestock production and significantly enhance the animal biotechnology field.
Outcomes or Projected Impacts
- Being able to identify and/or predict a skewed sex ratio in individual ejaculates of semen may lead to a practical, cost effective method to control the sex of offspring in livestock species.
- Improvement in semen storage procedures could enhance the efficiency and use of artificial insemination, especially in species where the semen cannot be successfully cryopreserved.
- In vitro-produced embryos have excess lipid content, and developing a method to decrease lipids will aid in improving systems of producing embryos in vitro.
- The development of efficient IVP systems using microscale technologies will enable the automation of such techniques for production of large numbers of embryos in vitro, which will speed the genetic improvement and selection of superior livestock.
- Manipulation of specific molecular mechanisms critical to oocyte competence, including DNA methylation, cell death pathways, cellular metabolism and mRNA processing, will allow scientists and clinicians to positively alter the developmental potential of oocytes.
- Identification of regulatory mechanisms controlling ICM and trophectoderm lineage differentiation will provide a better understanding of fetal and placental development and insights into the etiology of early embryonic loss and implantation failure.
- Identified spermatozoal transcripts in bull spermatozoa can be used to understand biology of spermatozoa and improve bull fertility.
- Improving our understanding of the mechanisms underlying gene expression in cloned vs. control animals has the potential to improve the survival of cloned animals, therefore providing a much needed increase in the efficiency of nuclear transfer procedures.
- Manipulation of the interaction between GnRH and its receptor in preimplantation embryos could culminate in novel contraceptive procedures.
- Research in bovine embryo physiology may provide insights into aberrant mechanisms that predispose the embryo to pregnancy loss due to abnormal development and/or implantation failure.
- Improving methods for manipulating the timing of meiotic maturation will improve the efficiency and effectiveness of in vitro embryo production.
- Identification of specific mRNAs regulating meiotic maturation will provide novel opportunities for development of gene-targeted contraceptive methods useful for the manipulation of reproductive cycles in livestock and other mammalian species.
- Improvements in livestock reproductive efficiency will provide consumers food and fiber products at reduced cost.
- The production of alpha-lactalbumin and IGF transgenic swine allow for improvement of lactation in swine production systems.
- The ability to isolate and differentiate mesenchymal lineage stem cells in vitro and the transplant them back into live animals with corresponding proper differentiation will allow stem cell therapy for production parameters such as lactation and muscle growth in livestock.
- Embryonic stem cells in livestock species, which are still unavailable due to inadequate culture conditions, will provide an invaluable tool in genetic engineering of transgenic animals for improved production traits, disease resistance and production of biopharmaceuticals.
- Results from freezing studies with embryos from different lines of swine could provide improved methods for cryopreservation of swine embryos so that valuable germplasm, including genetically altered animals, can be maintained for future use.
- Understanding the regulation of fetal development will lead to methods for identifying normal and abnormal growth patterns resulting from ARTs, and improved systems for producing embryos in vitro.
Milestones
(2010): At the annual meeting, elected members of the Project will present the first of a series of educational workshops focused on specific themes associated with the goals of the Project (i.e., oocyte maturation, microarray analysis, or stem cells). Presentations will be distributed via CDs or through a link on the NIMSS homepage for the Project.(2014): The development of a transgenic line of siRNA knock-down livestock. This exciting technology to analyze gene function in production animal species represents a major advancement in animal biotechnology and this Project will be at the forefront of this research focus area.
Projected Participation
View Appendix E: ParticipationOutreach Plan
Results from the proposed research are expected to have tremendous impacts on scientists, on persons engaged in animal production, and consumers. Gaining insights into the biological mechanisms underlying early embryonic development will enable researchers to devise better systems for the production of embryos genetically capable of more efficient production of food and fiber. Some of the immediate tangible benefits from this research will include the generation of scientific publications, which reflect the advancement of science, development of new methodologies that can be utilized in the private sector, training of graduate and postdoctoral students, and education of society through extension reports, and popular press publications. Once the new techniques have been incorporated into biomedical research and production agriculture, consumers will see new products emerging onto the marketplace as well as traditional products being sold at a reduced price because of their more efficient production. Of course, it will also offer the opportunity to decrease the number of animals needed to produce products that reach market, lessening animal waste production and reducing potential environmental concerns.
Members of the Project are committed to extension efforts, producing approximately 33 semi-technical/lay publications during the previous Project. It is expected that additional extension publications will result from the proposed research. In addition to extension reports/guides specific to individual states and/or experiment stations, research results will be disseminated via popular press articles in magazines such as Beef, Hoard's Dairyman, and National Hog Farmer. Another important avenue, relative to this committee, is the International Embryo Transfer Society (IETS) Newsletter which provides an excellent format for delivery of research results to an applied audience. Project members will continue to develop and participate in workshops designed to teach the principles and procedures of embryo-related technologies to applied specialists and clientele in the field. These concepts are further communicated to the public via CD and DVD media formats. Additionally, member institutions (IA, NE) have developed undergraduate and graduate level Embryo Transfer courses designed to educate and train students that represent future scientists, practitioners and embryo technologists. Finally, the annual meeting of the committee typically corresponds with the IETS Annual Meeting, limiting attendance to only Project members. The committee has elected to meet at member institutions when the IETS Annual Meeting is scheduled outside of the US. For example, the 2010 annual meeting of the committee will be held on the campus of Colorado State University. Platforms of this nature are much more conducive to interactions with extension faculty and clientele.
Studies on oocyte maturation, fertilization, and embryonic development are crucial to enhance livestock reproductive efficiency. Once the underlying biological mechanisms are better understood, problems with infertility that arise in the field may be more easily overcome. It will also enable a more prolific generation of embryos for research purposes, which ultimately will lead to further advances in many fields. Information generated from this research may be used to increase fertility of livestock species, decrease embryonic death, and ameliorate the declining fertility of older animals. There also will be more direct benefits for farmers who use assisted reproductive technologies such as superovulation, in vitro fertilization, and embryo transfer. These technologies increase reproductive rates of valuable females, even enabling generation of in vitro produced embryos from cows that are pregnant. These techniques already are being used to circumvent the drastic decline in fertility of dairy cows that has occurred over the past 2 decades, particularly with heat stress, and they also enable older, infertile females to continue to reproduce and provide products to market in the form of pregnant recipients and frozen embryos. The latter can be marketed easily internationally without a concern for transmitting diseases.
Studies on nuclear transfer, transgenesis, and cryopreservation are important to the future of society. It is likely that the research on cell cycle control, conducted as a part of research on somatic cell nuclear transfer, may lead to cures for cancer, treatments to reduce the aging process, and methods to generate organs for transplant. When nuclear transfer is combined with transgenesis, even greater possibilities exist for both animal agriculture and biomedicine.
Significant research effort concerning selective genetic modification of animals is occurring at public institutions and in private companies, and it is imperative that research of this type be publicly funded to ensure availability of results to animal agriculture and to private industry. Combining technologies such as in vitro fertilization, nuclear transfer, and cryopreservation provides phenomenal opportunities for increased efficiency of livestock production. Efficacious cryopreservation of oocytes would enable storage of female gametes for many purposes including banking to conserve genetic resources, particularly the mitochondrial component. Although embryos from ruminant species can be frozen reasonably well, the ability to cryopreserve oocytes will increase flexibility in animal production and in research. In vitro embryo production could be timed to use naturally cycling recipients. Oocytes from genetically elite females could be better utilized, leading to a faster rate of genetic improvement due to increased female selection intensity. Cryopreservation of embryos provides many benefits to the livestock industry, such as not needing recipients in "real time" and being able to export genetic material.
Organization/Governance
Governance of this Technical Committee will include the election of a Chair, a Vice-Chair, and a Secretary. Election of Secretary will occur annually. Secretary will rotate to Vice-Chair, and the Vice-Chair to Chair. The agenda for the annual meeting of the Technical Committee will be set by the Chair and he/she will preside over the meeting. The Secretary will prepare minutes of the annual meeting as well as the annual report. Administrative guidance will be provided by an assigned Administrative Advisor and a CSREES Representative.
Literature Cited
Abe, H., Hoshi, H. 2003. Evaluation of bovine embryos produced in high performance serum-free media. J. Reprod. Dev. 49:193-202.
Alm, H., Torner, H., Lohrke, B., Viergutz, T., Ghoneim, I.M., Kanitz, W. 2005. Bovine blastocyst development rate in vitro is influenced by selection of oocytes by brillant crexyl blue staining before IVM as indicator for glucose-6-phosphate dehydrogenase activity. Theriogenology 63:2194-2205.
Amann RP. 1989. Treatment of sperm to predetermine sex. Theriogenology 31:49-60.
Anonymous. 2003. FAIR 2002 Animal products for the next millennium - an agenda for research and education. May 2002.
Anonymous. 2009. Agricultural Outlook. 2008. Economic Research Service, U.S.D.A.
Au, H.K., Yeh, T.S., Kao, S.H., Tzeng,C.R., Hsieh, R.H. 2005. Abnormal mitochondrial structure in human unfertilized oocytes and arrested embryos. Ann. N.Y. Acad. Sci. 1042:177-185.
Bethelot, F., Martinat-Botte, F., Locatelli, A., Perreau, C., Terqui, M. 2000. Piglets born after vitrification of embryos using the open pulled straw method. Cryobiology 41:116-124.
Beyhan, Z., Forsberg, E.J., Eilertsen, K.J., Kent-First, M., First, N.L. 2007a. Gene expression in bovine nuclear transfer embryos in relation to donor cell efficiency in producing live offspring. Mol. Reprod. Dev. 74:18-27.
Beyhan, Z., Ross, P.J., Iager, A.E., Kocabas, A.M., Cunniff, K., Rosa, G.J., Cibelli, J.B. 2007b. Transcriptional reprogramming of somatic cell nuclei during preimplantation development of cloned bovine embryos. Dev. Biol. 305:637-649.
Brevini, T.A., Vassena, T., Francisci, C., Gandolfi, F. 2005. Role of adenosine triphosphate, active mitochondria, and microtubules in the acquisition of developmental competence of parthenogenetically activated pig oocytes. Biol. Reprod. 72:1218-1223.
Brown, R., Strathdee, G. 2002. Epigenomics and epigenetic therapy of cancer. Trends Mol. Med. 8(4 Suppl):S43-8.
Carnevale, E.M. 2006. Vitrification of equine embryos. Vet. Clin. North Am. Equine Pract. 22:831-841.
Cibelli, J. 2007. A decade of cloning mystique. Science 316:990-993.
Combelles, C.M., Racowsky, C. 2005. Assessment and optimization of oocyte quality during assisted reproductive technology treatment. Semin. Repod. Med. 23:277-284.
Conti, M., Andersen, C.B., Richard, F., Mehats, C., Chun, S.Y., Horner, K., Jin, C., Tsafriri, A. 2002. Role of cyclic nucleotide signaling in oocyte maturation. Mol. Cell. Endocrinol. 187:153-159.
Coticchio, G., Sereni, E., Serrao, L., Mazzone, S., Iadarola, I., Borini, A. 2004. What criteria for the definition of oocyte quality? Ann. N.Y. Acad. Sci. 1034:132-144.
Dai, Y., Vaught, T.D., Boone, J., Chen, S.H., Phelps, C.J. 2002. Targeted disruption of the alpha1,3-galactosyltransferase gene in cloned pigs. Nat. Biotechnol. 20:251-255.
Dattena, M., Ptak, G., Loi, P., Cappai, P. 2000. Survival and viability of vitrified in vitro and in vivo produced ovine blastocysts. Theriogenology 53:1511-1519.
Dean W., Santos, F., Stojkovic, M., Zakhartchenko, V., Walter, J., Wolf, E., Reik, W. 2001. Conservation of methylation reprogramming in mammalian development: aberrant reprogramming in cloned embryos. Proc. Natl. Acad. Sci. USA 98:13734 13738.
de Graaf, S.P., Evans, G., Maxwell, W.M.C., OBrien, J.K. 2007. Birth of offspring of pre-determined sex after artificial insemination of frozen-thawed, sex-sorted and refrozen-thawed ram spermatozoa. Theriogenology 67:391398.
Denning, C., Priddle, H. 2003. New frontiers in gene targeting and cloning: success, application and challenges in domestic animals and human embryonic stem cells. Reproduction 126:1-11.
Dinnyes, A., Nedambale, T.L. 2009. Cryopreservation of manipulated embryos: tackling the double jeopardy. Reprod. Fertil. Dev. 21:45-59.
Donovan, D.M., Kerr, D.E., Wall, R.J. 2005. Engineering disease resistant cattle. Transgenic Res. 14:563-567.
Eppig, J.J., Vivieros M.M., Marin-Bivens C., De La Fuente R. 2004. Regulation of mammalian oocyte maturation. In, (Leung, P.C.K., Adashi, E.Y. Eds.). The Ovary. Elsevier Academic Press, Amsterdam. pp. 113-129.
Forsberg, E.J. 2005. Commercial applications of nuclear transfer cloning: three examples. Reprod. Fertil. Dev. 17:59-68.
Fulka, Jr., J., First, N.L., Moor, R.M. 1998. Nuclear and cytoplasmic determinants involved in the regulation of mammalian oocyte maturation. Mol. Hum. Reprod. 4:41-49.
Gibson, T.C., Kubisch, H.M., Brenner, C.A. 2005. Mitochondrial DNA deletions in rhesus macaque oocytes and embryos. Mol. Hum. Reprod. 11: 785-789.
Gjorret, J.O., Maddox-Hyttel, P. 2005. Attempts towards derivation and establishment of bovine embryonic stem cell-like cultures. Reprod. Fertil. Dev. 17:113-124.
Golovan, S.P., Meidinger, R.G., Ajakaiye, A., Cottrill, M., Wiederkehr, M.Z., et al. 2001. Pigs expressing salivary phytase produce low-phosphorous manure. Nat. Biotechnol. 19:741-745.
Han Y.M, Kang Y.K, Koo D.B, Lee KK. 2003. Nuclear reprogramming of cloned embryos produced in vitro. Theriogenology 59:33-44.
Hansen, P.J., Block, B.J., 2004. Towards an embryocentric world: the current and potential uses of embryo technologies in dairy production. Reprod. Fertil. Dev. 16:1-14.
Harvey, A.J., 2007. The role of oxygen in ruminant preimplantation embryo development and metabolism. Anim. Reprod. Sci. 98, 113-128.
Hill, J.R., Burghardt, R.C., Jones, K., Long, C.R., Looney, C.R., Shin, T., Spencer, T.E., Thompson, J.A., Winger, Q.A., Westhusin, M.E. 2000a. Evidence for placental abnormality as the major cause of mortality in first-trimester somatic cell cloned bovine fetuses. Biol. Reprod. 63:1787-1794.
Hill J.R., Winger, Q.A., Long, C.R., Looney C.R., Thompson, J.A., Westhusin, M.E. 2000b. Development rates of male bovine nuclear transfer embryos derived from adult and fetal cells. Biol. Reprod. 62:1135-40.
Hong, Q.H., Tian, S.J., Zhu, S.E., Feng, J.Z., Yan, C.L., Zhao, X.M., Liu, G.S., Zheng, S.M. 2007. Vitrification of boer goat morulae and early blastocysts by straw and open-pulled straw method. Reprod. Domest. Anim. 42:34-38.
Hsieh, T.H., Tsai, N.M., Au, H.K., Chang, S. J., Wei, Y.H., Tzeng, C.R. 2002. Multiple rearrangements of mitochondrial DNA in unfertilized human oocytes. Fertil. Steril. 77:1012-1017.
Hurtt, A.E., Seidel, G.E., Jr., Squires, E.L. 1999. Vitrification of equine and bovine oocytes in an ethylene glycol, Ficoll and sucrose solution using open pulled straws. Theriogenology 51:166 abstr.
Jenuwein, T., Allis, C.D. 2001. Translating the histone code. Science 293:1074-1080.
Johnson, L.A., Cran, D.G., Polge, C. 1994. Recent advances in sex preselection of cattle: flow cytometric sorting of X- and Y-chromosome bearing sperm based on DNA to produce progeny. Theriogenology 41:51-56.
Keefer, C.L., Pommer, J., Robl, J.M. 2007. Role of transgenic livestock in the treatment of human disease. CAST Issue Paper 35:1-11.
Krisher, R.L. 2004. The effect of oocyte quality on development. J. Anim. Sci. 82:E14-E23.
Kues, W.A., Carnwath J.W., Niemann H. 2005. From fibroblasts and stem cells: implications for cell therapies and somatic cloning. Reprod. Fertil. Dev. 17:125-134.
Kues W.A. Sudheer S.,1, Herrmann D., Carnwath J.W. Havlicek V., Besenfelder U., Lehrach H., Adjaye J., Niemann H. 2008. Genome-wide expression profiling reveals distinct clusters of transcriptional regulation during bovine preimplantation development in vivo. Proc Natl Acad Sci USA 105:1976819773.
Kuroiwa Y, Kasinathan P, Sathiyaseelan T, Jiao JA, Matsushita H, Sathiyaseelan J, Wu H, Mellquist J, Hammitt M, Koster J, Kamoda S, Tachibana K, Ishida I, Robl J.M. 2009. Antigen-specific human polyclonal antibodies from hyperimmunized cattle. Nat Biotechnol. doi:10.1038/nbt.1521
Kuroiwa, Y., Kasinathan, P., Choi, Y.J., Naeem, R., Tomizuka, K., et al. 2002. Cloned transchromosomic calves producing human immunoglobulin. Nat. Biotechnol. 20:889-894.
Kuroiwa, Y., Kasinathan, P., Matsushita, H., Sathiyaselan, J., Sullivan, E.J., Kakitani, M., Tomizuka, K., Ishida, I., Robl, J.M. 2004. Sequential targeting of the genes encoding immunoglobulin-mu and prion protein in cattle. Nat. Genet. 36:775-780.
Lai, L., Kolber-Simonds, D., Park, K.W., Cheong, H.T., Greenstein, J.L., et al. 2002. Production of alpha-1,3-galactosyltransferase knock-out pigs by nuclear transfer cloning. Science 295:1089-1092.
Liu, Y., Du, Y., Lin, L., Li, J., Kragh, P.M., Kuwayama, M., Bolund, L., Yang, H., Vajta, G. 2008. Comparison of efficiency of open pulled straw (OPS) and Cryotop vitrification for cryopreservation of in vitro matured pig oocytes. Cryo Letters 29:315-320.
Lonergan, P., Rizos, D., Gutierrez-Adan, A., Fair, T., Boland, M.P. 2003. Oocyte and embryo quality: effect of origin, culture conditions and gene expression patterns. Reprod. Domest. Anim. 38:259-267.
Massip, A. 2001. Cryopreservation of embryos of farm animals. Reprod. Domest. Anim. 36:49-55.
Mehlmann, L.M., Saeki, Y., Tanaka S., Brennan T.J., Evsikov A.V., Pendola F.L., Knowles B.B., Eppig J.J., Jaffe L.A. 2004. The Gs-linked receptor GPR3 maintains meiotic arrest in mammalian oocytes. Science 306:1947-1950.
Mehlmann, L.M. 2005. Stops and starts in mammaliam oocytes: recent advances in understanding the regulation of meiotic arrest and oocyte maturation. Reproduction 130:791-799.
Misirlioglu, M., Page, G., Sagirkaya, H., Kaya, A., Parrish, J.J., First, N.L., Memili, E. 2006. Dynamics of global transcriptome in bovine matured oocytes and preimplantation embryos. Proc. Natl. Acad. Sci. USA 103:1890518910.
Miyano, T. 2003. Bringing up small oocytes to eggs in pigs and cows. Theriogenology 59:61-72.
Moon, J.H., Hyun, D.S., Lee, S.W., Son, W.Y., Yoon, S.H., Lim, J.H. 2003. Visulalization of the metaphase II meiotic spindle in living human oocytes using the Polscope enables the prediction of embryonic developmental comptence after ICSI. Hum. Reprod. 18:817-820.
Mullins, L.J., Wilmut, I., Mullins, J.J. 2004. Nuclear transfer in rodents. J. Physiol. 554:4-12.
Nagai, T. 2001. The improvement of in vitro maturation systems for bovine and porcine oocytes. Theriogenology 55:1291-301.
Nagai, T., Funahashi, H., Yoshioka, K., Kikuchi, K. 2006. Up date of in vitro production of porcine embryos. Front. Biosci. 11:2565-2573.
NASS. 2009. National Agricultural Statistics Service. USDA.
Niemann, H., Kues, W.A. 2000. Transgenic livestock: premises and promises. Anim. Reprod. Sci. 60-61:277-93.
Niemann, H., Rath, D. 2001. Progress in reproductive biotechnology in swine. Theriogenology 56:1291-304.
Niemann, H., Rath, D., Wrenzycki, C. 2003. Advances in biotechnology: new tools in future pig production for agriculture and biomedicine. Reprod. Domest. Anim. 38:82-89.
Oback, B. 2009. Cloning from stem cells: different lineages, different species, same story. Reprod. Fertil. Dev. 21:83-94.
Oberstein, N., ODonovan, M.K., Bruemmer, J.E., Seidel, G.E., Carnevale, E.M., Squires, E.L. 2001. Cryopreservation of equine embryos by open pulled straw, cryoloop or conventional slow cooling methods. Theriogenology 55:607-613.
Palasz, A.T., Beltran, B.J., De la Fuente, P., Martinez, M.F., Gutirrez-Adan, A., 2006. The effect of amino acids and hyaluronan on bovine embryo development and gene expression pattern. Reprod. Fertil. Dev. 18:194-194.
Pate, B.J., White, K.L., Winger, Q.A., Rickords, L.F., Aston, K.I., Sessons, B.R., Li, G.P., Campbell, K.D., Weimer, B., Bunch, T.D. 2007. Specific integrin subunits in bovine oocytes, including novel sequences for alpha 6 and beta 3 subunits. Mol. Reprod. Dev. 74:600-607.
Paterson, L., DeSousa, P., Ritchie, W., King, T., Wilmut, I. 2003. Application of reproductive biotechnology in animals: implications and potentials. Applications of reproductive cloning. Anim. Reprod. Sci. 79:137-43.
Peddinti, D., Nanduri, B., Kaya, A., Feugang, J.M., Burgess, S.C., Memili E. 2008. Comprehensive proteomic analysis of bovine spermatozoa of varying fertility rates and identification of biomarkers associated with fertility. BMC Systems Biology 2:19.
Prather, R.S. 2006. Cloned transgenic heart-healthy pork? Transgenic Res. 15:405-407.
Prelle, K., Zink, N., Wolf, E. 2002. Pluripotent stem cells: model of embryonic development, tool for gene targeting, and basis of cell therapy. Anat. Histol. Embryol. 31:169-186.
Rienzi, L., Ubaldi, F., Martinez, F., Iacabelli, M., Minasi, M.G., Ferrero, S., Tesarik, J., Greco, E. 2003. Relationship between meiotic spindle location with regard to the polar body position and oocyte developmental potential after ICSI. Hum. Reprod. 18:1289-1293.
Rizos, D., Gutierrez-Adan, A., Perez-Garnelo, S., de la Fuente, J., Boland, M.P., Lonergan, P., 2003. Bovine embryo culture in the presence or absence of serum: implications for blastocyst development, cryotolerance, and messenger RNA expression. Biol. Reprod. 68:236-243.
Rodriguez-Osorio, N., Wang, Z., Kasinathan, P., Page, G.P., Robl, J.M, Memili, E. 2009. Transcriptional Reprogramming of Gene Expression in Bovine Somatic Cell Chromatin Transfer Embryos. BMC Genomics (In Press).
Seidel, G.E., Jr. 2006. Modifying oocytes and embryos to improve their cryopreservation. Theriogenology 65:228-235.
Shaw, P.W., Bernard, A.G., Fuller, B.J, Hunter, J.H., Shaw, R.W. 1992. Vitrification of mouse oocytes using short cryoprotectant exposure: effects of varying exposure times on survival. Mol. Reprod. Dev. 33:210-214.
Shi, W., Haaf, T. 2002. Aberrant methylation patterns at the two-cell stage as an indicator of early developmental failure. Mol. Reprod. Dev. 63:329-34.
Somfai, T., Ozawa, M., Noguchi, J., Kaneko, H., Nakai, M., Maedomari, N., Ito, J., Kashiwazaki, N., Nagai, T., Kikuchi, K. 2008. Live piglets derived from in vitro-produced zygotes vitrified at the pronuclear stage. Biol. Reprod. 80:42-49.
Smith SL, Everts RE, Tian XC, Du F, Sung LY, Rodriguez-Zas SL, Jeong BS, Renard JP, Lewin HA, Yang X. 2005. Global gene expression profiles reveal significant nuclear reprogramming by the blastocyst stage after cloning. Proc. Natl. Acad. Sci. USA 102:17582-17587.
Sullivan, E.J., Pommer, J., Robl, J.M. 2008. Commercialising genetically engineered animal biomedical products. Reprod. Fertil, Dev. 20:61-66.
Sutovsky, P. 2009. Sperm-egg adhesion and fusion in mammals. Expert Rev. Mol. Med. 11:e11.
Thompson, A.J., McWhir, J. 2004. Biomedical and agricultural applications of animal transgenesis. Mol. Biotech. 27:231-244.
Trounson, A. 2005. Derivation characteristics and perspectives for mammalian pluripotent stem cells. Reprod. Fertil. Dev. 17:135-141.
Valdez, C.A., Abas Mazni, 0., Takahashi, Y., Fujikawa, S., Kanagawa, H. 1993, Successful cryopreservation of mouse blastocysts using a new vitrification solution. J. Reprod. Fertil. 96:793-802.
Vanroose, G., Van Soom, A., de Kruif, A. 2001. From co-culture to defined medium: State of the art and practical considerations. Reprod. Dom. Anim. 36:25-28.
Wall, R.J. 2002. New gene transfer methods. Theriogenology 57:189-201.
Wang, B., Zhou, J. 2003. Specific genetic modifications of domestic animals by gene targeting and animal cloning. Reprod. Biol. Endocrinol. 1:103.
Wang, Q., Qing-Yuan, S. 2007. Evaluation of oocyte quality: morphological, cellular and molecular predictors. Reprod. Fertil. Dev. 19:1-12.
Wassarman, P.M. 2009. Mammalian fertilization: the strange case of sperm protein 56. BioEssays 31:153-158.
Wells, D.N., Oback, B., Laible, G. 2003. Cloning livestock: a return to embryonic cells. Trends Biotechnol. 21:428-432.
West, F.D., Machacek, D.W., Boyd, N.L., Pandiyan, K., Robbins, K.R., Stice, S.L. 2008. Enrichment and differentiation of human germ-like cells mediated by feeder cells and basic fibroblast growth factor signaling. Stem Cells 26:2768-2776.
Wheeler, M.B. 2003. Production of transgenic livestock: Promise fulfilled. J. Anim. Sci. 81:32-37.
Wheeler, M.B., Malusky, S.A. 2003. Transgenesis in mammals: status and perspectives. Acta Sci. Vet. 31:119-136.
Wheeler, M.B., Walters, E.M., Clark, S.G. 2003. Transgenic animals in biomedicine and agriculture: outlook for the future. Anim. Reprod. Sci. 79:265-289.
Wilmut I, Sullivan G, Taylor J. 2009. A decade of progress since the birth of Dolly. Reprod. Fertil. Dev. 21:95-100.
Wood, M.J., Barros, C.J., Candy, C.J., Carroll, J., Melendez, J., Whittingham, D.G. 1993. High rates of survival and fertilization of mouse and hamster oocytes after vitrification in dimethylsulphoxide. Biol. Reprod. 49:489-495.
Wirtu, G., Pope, C. E., Damiani, P., Miller, F., Dresser, B. L., Short, C. R., Godke, R. A., and Bavister, B. D. 2004. Development of in vitro-derived bovine embryos in protein-free media: effects of amino acids, glucose, pyruvate, lactate, phosphate and osmotic pressure. Reprod. Fertil. Dev. 15: 439-449.
Zhu, S.E., Kasai, M., Ocog, H., Sakurai, T., Machida, T. 1993. Cryopreservation of expanded mouse blastocysts by vitrification in ethylene glycol-based solutions. J. Reprod. Fertil. 98:139-145.