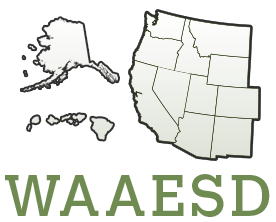
W2128: Microirrigation for sustainable water use
(Multistate Research Project)
Status: Inactive/Terminating
W2128: Microirrigation for sustainable water use
Duration: 10/01/2009 to 09/30/2014
Administrative Advisor(s):
NIFA Reps:
Non-Technical Summary
Statement of Issues and Justification
The water crisis and how microirrigation can address it.
The Challenge of the 21st century will be coping with water scarcity according to the Food and Agriculture Organization of the United Nations (FAO) Director-General, Dr Jacques Diouf (FAO,2007). Much effort will be required to meet food and freshwater demands for an anticipated 2030 global population of 8.1 billion. On a worldwide basis there was a five-fold increase in irrigation during the 20th century (ONeill and Dobrowolski, 2005) which probably cannot be repeated in the 21st century. The agricultural industry diverts the largest amount of water on a worldwide basis, so it must take a leading role in achieving sustainable water use that will provide for both the growing need for food and the need for clean and safe water supplies. Within the United States there has also been a realization of our own countrys water crisis and a call for action. According to the U.S. Department of the Interior (USBR, 2005) Water 2025 report, water planning for the year 2025 must be based on the reality that demand for water in many basins of the West exceeds the available supplies even in normal years. Since the West is also home to some of the fastest growing communities in the nation, increasingly frequent water supply crises are anticipated. In the past century water crises were intense but typically occurred in drought years; they only affected resources and economies of local and regional importance. Unless timely action is taken, it is anticipated that water supply-related crises will affect economies and resources of national and international importance. The U.S. Department of Interior has moved forward from its Water 2025 report with what it considers necessary efforts (USBR, 2008a and b) to increase water conservation, improve efficiency, and help secure future water supplies through competitive grants and technical assistance. The U. S. Department of Agriculture Cooperative States Research Education and Extension Service (USDA-CSREES) through its Agricultural Water Security (AWS) Initiative have identified three promising areas for CSREES research, education and extension programs. They are: 1) Exploring new technologies for the use of recycled water and water conservation in agricultural, rural, and urbanizing watersheds; 2) Probing the human, social, and economic dimensions of agricultural water security with a focus on adoption-outreach; and 3) Researching biotechnological improvements in water use efficiency of crop and horticultural plants to yield greater crop per drop. There are many challenges facing irrigated agricultural both domestically and abroad. Howell (2006) points out that until the mid 1990s, irrigated land increased at a faster than did the worldwide population growth rate. After that it appears that overall irrigated area growth has stopped while population continues to grow. He further stated that irrigation would remain vitally important in meeting the growing food and feed demands and that there were still great opportunities through agronomic, engineering, and management technologies to reduce non-productive water use in irrigated agriculture. Most water planners and resource managers recognize that there will be no magic bullet that will remove all of the worlds or nations water problems. Instead there is a growing realization that it will take many tools working together to help avoid the significant disruption in the economies and societies grown accustomed to widespread irrigation use. Microirrigation is just one of the many irrigation and water management technology tools, but it is a tool that has several advantages. Microirrigation can reduce the waste of water to a negligible amount and the transport of contaminants to surface water and groundwater. Irrigation events can be fine-tuned to spoon feed water and nutrients just in time to avoid plant stress. It can optimize crop production (more crop per drop) and in many cases increase the quality of agricultural products.
Issues and Justification for Objective 1
As the pressures on water resources continue to tighten the definition of sustainable water use, even microirrigation which is considered to be the most efficient irrigation method must be closely examined for additional water savings. The simplest and most common definition of irrigation scheduling is simply the determination of when and how much water to apply. Improvements in microirrigation timing and amounts represents an important topic area where additional water savings can be made, but it must be carefully balanced with the primary economic goal of microirrigation which is to improve crop yield and quality. Several microirrigation scheduling approaches could be chosen for a particular crop in a particular environment. There are also many practical factors which must be considered when developing an irrigation schedule (e.g., system type and design), but the most widely accepted framework for understanding overall plant water requirements is a mass balance approach based on the concept of evapotranspiration (e.g., Howell and Meron, 2007.). In this approach, reference ET for a standard canopy is calculated from weather data and then multiplied by one or more crop coefficients (Kc) to estimate the evapotranspiration for the crop in question. This basic approach was already well developed over 40 years ago (Doorenbos and Pruitt, 1977) and has been further refined (Allen et al., 1998), but current research efforts are still underway to reevaluate published crop coefficients, particularly in woody perennials (Snyder, 2008). The methods used for on-farm irrigation scheduling can be roughly classified into three types, depending on the primary basis for the schedule: 1) ET-based, 2) soil-based and 3) plant-based. An ET-based schedule may use current weather data together with a published Kc to primarily determine how much water is used by the crop on a daily basis, and secondarily consider factors such as irrigation system capacity (typically designed to meet maximal crop ET (ETc)), root depth and soil characteristics to determine how often to replace ETc. A soil-based schedule may use a direct or indirect measurement of soil water to primarily determine when water must be added to the soil, and secondarily consider factors such as irrigation system capacity and root and soil factors to determine how much water can be reasonably added during the irrigation event. A plant-based schedule may use a direct or indirect measurement of plant water status to primarily determine when water must be applied, and secondarily consider factors such as irrigation system capacity and soil factors to determine how much can be added during the irrigation event.
While most practical guides to irrigation scheduling (e.g., extension publications such as Ashley, et al., 1998; Ley et al., 1994; Neibling et al., 2004, and Reddy et al., 2007) recommend that all of the above mentioned factors should be considered, it is generally assumed that crop ET needs must be fully met by a combination of precipitation, applied water, and stored soil water, in order to achieve maximum production. However, crops are complex biological systems, and in some crops, economic and/or horticultural benefits of deficit irrigation have been shown (e.g., for "regulated deficit irrigation (RDI)," Boland et al., 1993, Shackel et al., 2000). A key limitation to the use of this approach is that the benefits of RDI have also been shown to be highly site specific, even within a field (Lampinen et al., 1995). Current microirrigation systems are capable of a substantial degree of scheduling flexibility, including the ability to respond to current weather conditions (Shedd et al., 2007), and it is not unreasonable to anticipate that future systems may include the ability to control water application at a very fine spatial scale (e.g., individual trees in an orchard) through wireless networks or by other means. The key issue for each crop and environment however, will be to what extent a deficit and/or spatially variable irrigation strategy will influence ultimate crop productivity (yield, quality). For field corn, Evett et al. (1996) reported that one plant-based approach (based on a threshold canopy temperature) required more irrigation water, but gave significantly higher yields than an approach based on replacing 100% of ETc (based on soil water depletion using neutron scattering) for field corn in Texas. In this study, similar plant-based approaches, but using different temperature thresholds, achieved slightly higher yields (although not statistically significant) compared to the control for about the same amount of irrigation as used in the control. Data are needed from a range of crops and environments to quantify the effects of different irrigation scheduling approaches on the relation of yield or product quality to applied/available water.
Soil-based scheduling methods which use sensors and controls to initiate and terminate irrigation can also be highly appropriate for microirrigation with its high degree of automation and application uniformity. There have been major advances in these sensors, including improved reliability and communication capabilities in the age of the internet. Several participants of the proposed project will be comparing and calibrating different types of sensors and controls for irrigation to more traditional irrigation scheduling techniques.
Issues and Justification for Objective 2
Sustainable water use through microirrigation can be approached through the concept of more crop per drop. Thus in addition to improving sustainable water use through Obj. 1 of the proposed project with water savings obtained with improved microirrigation scheduling, it is also important to improve crop yields through improved microirrigation management and to increase usage and reliability of microirrigation systems through better design and maintenance. Microirrigation is by far not the predominant irrigation method in the United States but interest in the technology is growing. Early and growing adoption of microirrigation usually begins with comparison of microirrigation to more traditional irrigation methods for the region. These system comparisons may be based on pertinent factors such as crop yield and economics, water use and conservation, and environmental issues (chemical leaching and drainage). Although the pertinent factors may differ with region, crop, soil, and climate constraints, the goal is primarily to develop proper management strategies for the various irrigation methods, particularly for those methods such as microirrigation less familiar to producers. In the proposed study, much needed baseline information about alternative irrigation systems for the typical crops in each region will be obtained. The project participants will share results and develop common guidelines for optimizing performance of the various irrigation systems from both an economic and environmental standpoint. Subsurface drip irrigation (SDI) is one of the fastest growing irrigation methods in the non-traditional microirrigation regions of the country because subsurface installation allows for high initial system costs to be amortized over many years. Formal and informal producer surveys conducted under the current USDA-RRF W-1128 project have indicated that SDI is undergoing rapid expansion in some areas of the United States, particularly in TX for cotton production. As SDI continues to penetrate into new areas, there will be greater needs for research and extension efforts to help producers manage for optimal crop production, protect the environment and maximize system life through proper maintenance. Emitter clogging remains the primary cause of microirrigation system failure around the world, so improved emitter maintenance will be a key factor in having sustainable microirrigation systems. There have been few efforts to unify and summarize the emitter clogging results from the diverse regions of the United States primarily because microirrigation is a small evolving technology and because newly emerging regions did not have or could not allocate sufficient expertise to build on earlier research efforts from other regions. Several participants working within Obj. 2 and 4 of the proposed project will be working to create a web-based tool for microirrigation that can extend across the United States.
Issues and Justification for Objective 3
The conjunctive use of agricultural chemicals (agrochemicals) with microirrigation can help achieve sustainable water use in the United States through higher water productivity, through greater crop yields and improved crop quality, and through protection of surface and ground water resources from agrochemical pollution in runoff and leachates.
Agrochemicals, whether applied through the irrigation system or through other means, are used for a wide range of purposes. In maintenance of microirrigation systems, acids, chlorine, herbicides and other products are sometimes used to prevent emitter clogging due to chemical precipitates, biological growths, or root intrusion. Precise application of fertilizers and/or pesticides through the microirrigation system is often cited as an advantage of microirrigation (Ayars et al., 2007). Effective use of fertilizers or other agricultural chemicals applied through other means (ground rig or aerial application, for instance) may require extra considerations in microirrigated conditions. Potential obstacles to agrochemical applications with microirrigation include limitations to applicability of soil injected chemicals and limitations due to limited agricultural chemical labeling for microirrigation application. Microirrigation chemigation is based on the principles of precision farming where system inputs are qualitatively and quantitatively matched to the needs of the crop. Subsurface drip (SDI) and surface drip (DI) systems can be used to for the injection of systemic pesticides and some biocontrol agents while surface microsprinklers may be used to apply biocontrol agents over larger areas and on plant canopies. Use of SDI systems for systemic insecticide or fungicide application has the advantage of compatibility with integrated pest management principles. However, the use of pesticides through microirrigation systems is much less advanced as compared to nutrient fertigation. Current research programs conducted by participants of this proposed project as well as by others across the United States are beginning to address fertigation and chemigation through microirrigation (particularly through subsurface drip irrigation), yet results are generally preliminary or otherwise not sufficiently interpreted for development of best management practices. Research and extension/outreach associated with this project will advance knowledge necessary to develop BMPs and interpret this knowledge into BMP recommendations.
Issues and Justification of Objective 4.
Sustainable water use in the United States can be greatly augmented by microirrigation use of non-potable waters. Extending the concept of sustainability, the sustainable use of microirrigation for use with non-potable waters requires careful selection of system components and appropriate management of the whole microirrigation system. Use of non-potable waters as a microirrigation water sources is becoming more common as limited high quality water sources are first used by municipal and industrial users. Agricultural water needs are then supplied by lower quality water, including saline waters, reclaimed water from treatment plants, and water produced by animal agriculture operations. Use of non-potable water for irrigation has advantages since the water is often rich in nutrients beneficial to crops and use of the non-potable water for irrigation often reduces treatment costs and environmental impacts. Non-potable water use through microirrigation also has disadvantages since some of the water constituents may adversely impact soil quality and plant growth. Operation and maintenance of microirrigation systems may also be a challenge when poor water quality sources are used. Because these non-potable waters can come from processing facilities, homes, municipal treatment plants, rural municipal lagoons, and livestock lagoons, the characteristics of these non-potable water sources can vary widely in terms of chemistry, biological activity, and physical condition. These characteristics will influence filtration requirements, treatment practices, emitter performance, soil quality, and crop and landscape performance. Sometimes the non-potable water is used primarily as a substitute in irrigation, saving fresh water for other uses, while in other cases such as in on-site residential wastewater use this application method is primarily for pathogen reduction and protection of freshwater sources. Additional research is needed to evaluate water treatment practices with non-potable water sources that include assessment of filter systems and the effects of emitter design on long-term performance, as well as other factors. This collaborative research effort being proposed in this project will allow researchers to develop better recommendations for system hardware selection, improved maintenance procedures and guidelines for non-potable water utilization for different geographic locations, environmental conditions, soil characteristics, and water sources.
Why this project and why now?
A cooperative multi-state approach towards technology development can be extremely fruitful as evidenced by the significant body of research and extension efforts developed by W-128 and W1128 participants since 1972. This cooperative approach allows for the evaluation of group hypotheses and for distillation of common results into general guidelines. The multi-state, regional project approach can also be utilized as a peer review process for hypotheses developed by individuals with the hopes of expanding or advancing the overall scientific understanding. Another advantage of the cooperative approach is its utilization as a strong feedback mechanism to point out where there is not enough information or theoretical understanding to develop general guidelines. In the proposed revision, W-2128 members will utilize the distillation process, peer review process and the feedback mechanism to accomplish the project objectives. In many cases, it will be necessary to refine and adapt microirrigation technologies for site specific conditions (crops, soils, water quality and availability, climate and irrigation system characteristics). In other cases, it will be necessary to improve and develop management strategies to take advantage of the inherent capabilities of microirrigation.
Some scientists, water planners, and resource managers have been disappointed with the rate of adoption in microirrigation. The U.S. land area that is microirrigated varies on an annual basis but during the last 10 years has hovered in the range of 3 to 4% of the total irrigated area. It is recognized that some crops and locations are not physically or economically suitable for microirrigation, but this is probably the exception rather than a common situation. Although microirrigation is expensive, there is a growing realization that leaders in the farming community can and will adopt and adapt cutting-edge technologies when given the knowledge and incentives to do so. This is evidenced in the tag line of the recent USDA-NRCS Conservation Security Program (CSP) which is reward the best and motivate the rest.
Success within the proposed project will have a positive impact on addressing the Challenge of the 21st century. The primary consequences of not doing the research are that significant knowledge gaps about the use of microirrigation and its associated technologies will continue to discourage producers from adopting it and through its continued lack of growth, worldwide water problems will continue unabated.
Related, Current and Previous Work
A recent CRIS search indicated 445 records related to microirrigation (63), micro-irrigation (140), micro irrigation (140), drip irrigation (362) or trickle irrigation(30) although the ASABE has defined the preferred spelling as microirrigation (ASABE Soil and Water Division Terminology, ASAE S526.3). There were 256 active records. Most of these records can be categorized into 4 major categories that are not strongly related to the proposed project. These categories include a large number of projects related to finding a suitable alternative to fumigation with methyl bromide, an appreciable number of crop breeding projects and other similar issue projects where microirrigation just provides a highly uniform irrigation method, and a moderate number of studies where microirrigation is desirable for minimizing pest and disease control within the research. The search found 76 records that could be classified as at least semi-related to the efforts of the proposed project. Fifty of these 76 records were either the current project USDA-RRF, W-1128, Reducing Barriers to Adoption of Microirrigation, related to current projects of specific member individuals of W-1128, or related to current projects of specific member individuals proposing membership in this project revision W-2128. A project at the University of Arizona will be examining pathogen transfer in microirrigation which has some relationship to efforts in the proposed revision under Obj. 4. Another Univ. of Arizona project will evaluate SDI for vegetable production. The project revision W-2128 will use its current University of Arizona member to keep the project informed of these other projects results. Similarly there were two projects in FL that had some relationship to current W-1128 members as were two projects related to our new USDA-ARS/GA member. Four projects from Nebraska with microirrigation are related to irrigation and nutrient management on silt loam and sandy soils. These projects can be a good complement but do not duplicate other proposed efforts in KS and TX. Several other projects were related to members of the USDA Ogallala Aquifer Project of which proposed revision W-2128 participants KS, TX and USDA-ARS/Texas are working members of that group. The vast majority of the remaining projects were related to a multi-state project to be discussed in the next paragraph.
The USDA RRF S-1018 project, Irrigation Management for Humid and Sub-Humid Areas does have some activities that are somewhat similar in nature to the proposed project revision W-2128. Several current participants in W-1128 also have membership in this other project (AL, FL, USDA-NRCS and VI and a large number of other W-1128 participants have strong working relationships through serving on professional society committees. As the title of S-1018 implies, much of the effort of the project is focused on improving management of irrigation in the humid and semi-humid regions. However, their first objective (Improve automation, control, and distribution technology to increase irrigation efficiency) does complement some of the activities being proposed under Obj. 2 of this project revision, W2128. The S-1018 project is being revised and has temporary number S_TEMP2042. Although the proposed new objectives were not at the NIMSS site as late as January 13, 2009, it appears from the available Statement of Issues and Justification that the major thrusts will be related to the continuing drought situation in the SE United States and the need to adequately assess surface water supplies, improving water management procedures for situations where in-season water supplies are inadequate and increased efforts on automated and wireless sensor control of irrigation in municipal and residential settings.
The current project W-1128 had three objectives: 1) to identify and assess the significance of barriers to adoption of microirrigation; 2) to reduce technical barriers associated with microirrigation system design, performance, and maintenance; and 3) to reduce existing water and nutrient management barriers associated with microirrigation. Although overall accomplishments of the project has been good as evidenced by 178 total publications in the annual reports from 2004 through 2008, there was variation in progress between objectives. Retirements and position changes of some of the participants did affect progress for some objectives. Although many participants indicated they would participate in Obj. 1 through producer surveys, the efforts were mostly limited to CO, KS and TX. Some sources of information that had traditionally been used for collecting information about microirrigation scaled back their efforts and some increased restrictions on access. Good progress was achieved with Objective 2 and as a result a spin-off outreach effort in the project revision is to develop a interactive microirrigation maintenance website. Similarly comparison of irrigation scheduling approaches through ET, irrigation and precipitation indexes was initiated under W-1128, but will be further examined within the project revision. Some additional review of W-1128 progress will be indicated in various topics discussed later in this section.
Irrigation scheduling and water management takes on many forms (Hoffman et al., 1985). Some of these techniques are soil-based, some are plant-based, some are climatic-based and some are a combination of two or more approaches. In specific circumstances, all of these methods can be particularly meritorious for microirrigation. As part of the USDA RRF W-1128 project, members shared data on the relation of yield to applied water from irrigation experiments that covered a range of crops and environments. In order to account for differences in crops, years, and environments, the relative yield was compared to various estimates of relative water use. In some cases, the relation of yield to water use exhibited the typical pattern for a crop water production function (e.g., Al-Jamal et al., 2000), but in other studies there were less clear relationships with surprisingly low amounts of water being adequate to achieve full yield. For the data set as a whole, there was also some evidence that plant-based irrigation approaches required less water to achieve maximum yield than soil or ET-based approaches, but since most studies only considered soil- or ET-based approaches, more comparisons involving plant-based approaches will be needed to allow firm conclusions. One explanation for the maintenance of yield without application of a full crop water requirement is that the full water requirement may have been met by the use of soil water reserves. If this is the case, and plant or soil-based approaches allow for a more efficient or reliable use of soil water reserves than an ET-based approach, then this will be an important advantage of these alternative irrigation scheduling methods to document. Another possibility is that published crop coefficients are not correct in some climates (Annandale and Stockle, 1994). In the humid Northeast US, it was found that apple tree crop coefficients in relation to ETo varied daily over 2-fold especially in relation to vapor pressure deficit (VPD), Dragoni, et al., 2005), indicating that a grass reference ETo may not be a good model for tree or vine crops under some conditions.
One important plant-based scheduling approach for woody perennials is the measurement of midday stem water potential (SWP), and a recent USDA-SCRI grant (#2008-04985: " Advanced sensing and management technologies to optimize resource use in specialty crops: case studies of water and nitrogen in deciduous crops," P.H. Brown et al.) was funded to develop a remote sensing approach for determining SWP. Since this project already involves current W-1128 members (CA, NM), there will be a great opportunity for synergism in relating the plant-based measures of stress and yield effects across a wide range of crops and environments in the current proposal to the remotely sensed measures of stress that can be made available from the SCRI proposal. The Temperature-Time Threshold (TTT) was developed as a new plant-based irrigation scheduling technique for evaluating crop water stress by USDA-ARS research scientists at Lubbock, Texas. This technique has been patented as Biologically-Identified Optimal Temperature Interactive Console (BIOTIC) for Managing Irrigation by the USDA under Patent No. 5,539637 (Upchurch et al., 1996). Briefly, the TTT technique can be described as comparing the accumulated time that the crop canopy temperature is greater than a crop-specific temperature against a specified critical time developed for a well-watered crop in the same region. Irrigation is applied when the threshold time is exceeded. Studies in Texas have shown that TTT technology can be used to effectively irrigate cotton, soybeans, and corn (Wanjura et al., 2003; Evett et al., 2002). Research by Evett et al. (2002) has shown that the TTT technology can be used for different goals such as crop yield optimization or maximization of water use efficiency with just minor adjustments to the protocols.
Although a thorough review of the use of soil water sensors for irrigation management was provided by Phene et al. (1990), there have been many advances since that time. There are now soil water sensors that can be networked to provide data from multiple areas in the field and thus giving a more reliable estimate of soil water conditions. These sensors which use a variety of physical and theoretical approaches to measurement sometimes are only appropriate for specific soil types and uses. This can particularly be the case in use with microirrigation where the soil is not uniformly wetted (Zoldoske and Solomon, 1990). In other cases the sensors can be used only with proper calibration. Several participants of the proposed project will be comparing and calibrating different types of sensors and controls for irrigation to more traditional irrigation scheduling techniques. Kaleita, et al. (2003) has developed a methodology for mapping surface soil water content across an agricultural field from optical remote sensing and ground sampling. After determining appropriate wavelengths for soil water estimation from spectral reflectance, a cokriging scheme was used to generate soil water maps. Results indicate that combining reflectance and ground measurements can yield more detailed maps of soil water than ground measurement alone. Yang and Kaleita (2007) found that elevation explains a large portion of spatial pattern and variability of soil water. Other factors that affected water patterns were soil type, vegetation and other topographic indices. Microirrigation scheduling with TDR probes controlling an SDI system was used for sweet corn production in Florida (Dukes and Scholberg (2004). The soil water-based irrigation scheduling regime resulted in high frequency short duration irrigation events to meet crop water needs. In contrast, timebased SDI treatments were underirrigated but showed evidence of considerable drainage based on soil water measurements due to single daily irrigation events that promoted movement of irrigation water below the root zone. Soil water-based microirrigation scheduling can also reduce the amount of water applied to a crop, while maintaining ideal soil water contents for crop production. Shock, et al. (2002) monitored soil water content and demonstrated irrigation scheduling using Watermark Soil Moisture Sensors and Hansen units (soil moisture dataloggers with graphic display). The units were found to be a very effective irrigation scheduling aid.
Universities in TX and KS and the USDA-ARS sites in TX (which are also participants in this proposed project) have considerable joint research activity concerning development of SDI as part of the USDA-ARS Ogallala Aquifer Project. KS has maintained a website devoted to SDI topics (SDI in the Great Plains, http://www.oznet.ksu.edu/sdi/) since 1998. Although there have been some very good summaries and discussion of SDI research efforts (Camp, 1998; Camp et al., 2000; Ayars, et al., 1999; Lamm and Trooien, 2003, Lamm and Camp, 2007) which have outlined many of the design considerations for SDI from a conceptual standpoint, there still remains a need to develop management procedures and design adaptations as SDI expands into new areas with different climates, soils and cropping systems. The proposed project can help to develop new and broaden existing guidelines. When considering the use microirrigation, often the first question is how it will perform compared to other alternative irrigation systems. Sammis (1980) compared sprinkler, surface drip, subsurface drip, and furrow irrigation for the production of potato and lettuce in New Mexico. Subsurface drip irrigation (SDI) with a -20 kPa irrigation criterion was among the most productive irrigation systems. Smajstrla et al. (2000) compared automated controlled SDI irrigation with the conventional semi-closed seepage irrigation (subirrigation) in Florida. The SDI system required more electrical energy but used 36% less water to obtain the same potato yield. They concluded that at that time the economics favored semi-closed seepage irrigation. Comparison of drainage calculations beneath SDI treatments and sprinkler treatments indicated that up to 24% less drainage may have occurred on SDI plots compared to sprinkler plots largely because SDI applied water to the root zone and not to the furrows (Dukes and Scholberg, 2004). Colaizzi et al. (2006) summarized research in KS and TX compared with SDI and various types of in-canopy and near-canopy center pivot sprinkler irrigation for corn, cotton, grain sorghum, soybean, and sunflower. In general SDI performed as well or better than sprinkler irrigation, particularly so under deficit irrigation. SDI is particularly suited to small and oddly-shaped fields where that center pivot sprinkler quickly lose their cost advantage where they cannot make a complete circle (OBrien et al.,1998; Bosch et al., 1992). KS has developed a spreadsheet to compare the economics of SDI and sprinkler irrigation for corn production (Lamm et al., 2008).
Emitter clogging problems often have specific characteristics that are related to the water source and the region. Gilbert and Ford (1986) specifically listed three regions of the United States with distinctive differences in clogging issues. In the Southwestern United States, clogging was primarily associated with total suspended solids and water hardness. In the Southeastern United States, high biological activity associated with iron and sulfide-laden waters was a concern. In Hawaii, the clogging issue was fine sand particles. Since 1986, microirrigation has expanded into additional U. S. locations and along with this expansion came new clogging issues and constraints. Scientific approaches to the assessment and correction of clogging and maintenance issues have been reported by Meyer (1985), Ford (1979), Pitts et al. (1990), and Kidder and Hanlon, (1997). Recent publications from California (Morris and Schwankl, 2008; Schwankl et al., 2008) and a recent microirrigation book (Lamm et al., 2007) provide excellent sources of information on emitter clogging and system maintenance, but the logical next step is to provide web-based interactive tools for microirrigation maintenance.
Many microirrigation systems are well suited for agrochemical applications. Appropriate BMPs can reduce losses of agricultural chemicals through runoff or deep percolation, thereby reducing risk of contamination of groundwater or surface water resources. Management practices and technical considerations factor into maximizing effectiveness and efficiency in application of agrochemicals. While advancements have been made in this area, there are still significant knowledge gaps, technical limitations and other concerns related to agricultural chemical use with microirrigation systems. Several recent and ongoing studies are addressing crop-specific recommendations for nutrient management for microirrigated crops. Fertigation of nitrogen and potassium for cotton production indicated fertigated cotton yields were higher than non-fertigated control, with higher yields observed in treatments receiving fertigation in a one-year study (Dougherty, et al., 2007), but mixed results were observed in comparing surface-applied (only) and SDI drip fertigation with and without surface applied pre-plant fertilizer applications when the study was continued over two years (Dougherty, et al., 2008). Residual ammonium- and nitrate-N levels in the soil profile, corn yields, apparent nitrogen uptake (ANU), and water use efficiency (WUE) were utilized as criteria for evaluating N fertigation rates and in developing a best management practice recommendation for irrigation and fertigation of corn on the deep silt loam soils of western Kansas (Lamm, et al., 2003). Applications of this BMP for in-season fertigation through SDI resulted in high yields with high efficiency in both nutrient and water use. Fertigation through SDI systems at recommended nitrogen rates according to corn growth stages can increase yields and gross return after N application costs, and reduce nitrate-N leaching in soils compared to concentrated early season applications (Tarkalson and Payero, 2008). Noting a lack of (and growing producer demand for) information regarding nutrient management for subsurface drip irrigated cotton production systems, Bronson, et al. (2004) developed best management practices based upon the current knowledge of the rapidly expanding SDI cotton production in the Texas High Plains. The proposed studies in the W-2128 project can continue to develop BMPs for fertigation with microirrigation systems and broaden their applicability. Although nutrient application through microirrigation is the primary chemigation activity, there are some opportunities to also apply pesticides that can enhance crop production and protect the environment. Drip chemigation of imidacloprid under plastic mulch prevented both pesticide leaching through the root zone and pesticide transport via soil erosion off raised beds, proving water quality protection benefits as well as yield increase in muskmelon production (Leib, et al., 2000). In another study, Leib et al., (2003) used a finite element model (LEWASTE) to simulate drip chemigation of a pesticide (imidacloprid) in raised beds under plastic mulch; advective/dispersive movement of the pesticide from rainwater infiltration and drip irrigation; and root extraction of the pesticide with soil water. The model successfully predicted soil concentrations of imidacloprid that were highly correlated with measured concentrations. According to Trout and Ajwa (2003) chemical fumigants can be applied effectively and economically in irrigation water through drip irrigation systems with efficacy equivalent to shank-applied fumigants for bedded, plastic mulched, drip irrigated crops.
When considering agrochemical application in conjunction with microirrigation, water quality protection must be considered in addition to improving crop production and improving agrochemical efficacy. Modeling approaches such as HYDRUS 2D can be used to identify both design and operational procedures for using microirrigation systems that can minimize leaching and runoff. Preliminary results from development of a spatially variable microsprinkler system for orchard irrigation and fertigation have shown that spatially variable management is possible (Coates, et al., 2004). Anticipated benefits include higher crop yield, water conservation, and reduced nutrient leaching. In addition there are opportunities to use soil amendments in conjunction with microirrigation to prevent environmental contamination. Entry and Sojka (2003) found that water-soluble anionic PAMs that are typically used for erosion control were also effective in reducing concentrations of nitrate-N, total phosphorus and other micronutrients in runoff water. Flanagan et al. (1997), examined effects of electrolyte concentration in rainfall and runoff water, as well as the effect of different soil surface amendments on infiltration, runoff, and soil loss. Through rainfall simulator studies conducted on a silt loam soil, they found that addition of anionic PAM increased rate of infiltration of water into the soil. The long term effectiveness of these soil amendments and their economics may be questionable, but they may be especially beneficial in specific circumstances such as container production of ornamental plants and also to help stabilize highly disturbed soils in urban landscapes.
The use of non-potable waters and biological effluents through microirrigation systems is growing worldwide (Asano et al., 1996; Angelakis et al., 1999; Trooien and Hills, 2007). Reclaimed water, from various levels of treatment, is becoming frequently used for crop production and residential landscape irrigation (Parsons, et al., 1995; Parsons et al., 2001; Jnad 2001a and b; Yost, 2002; Trooien and Hills, 2007). Much of this use is through microirrigation systems to minimize associated safety and health concerns. While drip irrigation is well matched to reclaimed water use, issues with emitter clogging, plant response, and pathogen transport need additional investigation to develop management practices to make drip irrigation with reclaimed water successful.
The advantages and disadvantages of using of biological effluents through SDI were listed by Gushiken (1995) and Trooien et al. (2000). A study of wastewater effluent application through four different dripline products was conducted by Hills and Brenes (2001). That study used secondary effluent from an activated sludge wastewater treatment plant. The water was filtered and treated with chlorine. However, livestock lagoon effluent has high levels of ammonia and other constituents that make continuous chlorination impractical. Trooien et al. (2000) evaluated livestock lagoon wastewater applications with 5 different drip irrigation laterals with emitter flow rates that ranged from 0.57 to 3.5 L/h. They stated that the lower flow rate emitters (<0.91 L/h) may be risky for long-term use with wastewater. Lamm et al. (2002) continued this research and found that aggressive flushing resulted in partial recovery of emitter flow rate with higher discharge emitters showing greater recovery. Hagedorn (2003) is evaluating the soil microbiological impacts associated with subsurface drip irrigation of septic tank-based effluent. Yost (2002) is measuring the long-term effects of domestic wastewater effluent application on soil quality and turfgrass growth. Trooien (2002) is evaluating filtration system design and requirements for use on lagoon systems and microirrigation. Some of the current microirrigation products have been modified and are promoted for use with wastewater sources. However, some of these products can cost twice as much or more than standard, non-treated drip products. Therefore, additional research is needed on the long-term hydraulic performance and required maintenance of these products with various wastewater sources and under varying operational and environmental conditions. Where freshwater, in addition to reclaimed water or animal lagoon effluent, is available for irrigation, periodic chlorination when using freshwater needs to be investigated as a maintenance strategy.
Objectives
-
Compare irrigation scheduling technologies and develop grower-appropriate scheduling products
-
Develop design, management and maintenance recommendations
-
Develop best management practices for application of agrochemicals
-
Evaluate use of non-potable water through microirrigation
Methods
Methods for Subobjective 1a (Correlation of applied water, reference ET and yield) Current and historical data on applied water, reference ET and yield for a range of crops and environments will be pooled into a common data base in order to compare results between states, particularly with respect to comparisons of alternative microirrigation scheduling approaches (ET-based, soil-based and plant-based). In each experiment involving more than one level of applied water, reference ET will be calculated for both short and tall crops (ETos and ETrs, respectively) based on the standardized Penman Monteith equation from ASCE-EWRI (see http://biomet.ucdavis.edu/). Either daily or hourly weather data will be acceptable as inputs for the calculation of ET, and as far as possible, weather data will be obtained under environmental conditions representative of the agricultural conditions under study. All quantities of applied water, rain and ET will be expressed as equivalent depth units (mm) over the entire planted area under consideration, and both yield and relative yield (relative to the highest yielding treatment) will be reported. Data will be contributed from the following participants and crops: AL (cotton, corn soybean, wheat), CA (almond, pecan, grape), FL (citrus, blueberry, strawberry) HI (sweet corn, vegetables), ID (turf, alfalfa, sugar beets), KS (corn, grain sorghum, sunflower, alfalfa, soybean), NM (pecan, poplar, tomato, onion, pepper, and squash), NY (apple, grape), OR (onion, poplar, potato), PR (citrus, avocado), TX (corn, cotton, sorghum, soybean) and USDA-ARS/GA (cotton, corn, peanut). The participants will work jointly to cross-correlate results and/or to develop generalized indexes so that the alternative scheduling approaches can be used over a broader range of conditions. Methods for Subobjective 1b (Development of irrigation scheduling technologies and grower-appropriate scheduling products) A technical session at an irrigation-related conference devoted to comparing and contrasting these alternative microirrigation scheduling approaches will be proposed as a service to both academics and irrigation consultants and will aid the members of USDA RRF W-2128 (this proposed project) to focus their efforts on filling the information gaps. In addition to summarizing the technical aspects of the alternative approaches, there will also be presentations covering the social and economic aspects of the scheduling approaches. Project participants CA, ID, KS and TX have agreed to spearhead this effort. An additional thrust of this subobjective will be to compare and test various soil- and plant-based irrigation thresholds and approaches for on-farm use. Project participants that will primarily test soil-based thresholds for irrigation scheduling will be FL (citrus, blueberry, and strawberry), USDA-ARS/GA (cotton, corn, and peanut), HI (sweet corn), and ID (alfalfa and sugar beet). Some participants will also focus on field calibration of specific soil moisture sensors (ID, using Decagon EC-5 soil moisture probes and EM50R data loggers, NM using TDR and hydra sensors, IA using Decagon EC-5 and EC-10 soil moisture probes, Campbell Scientific CS616 soil moisture probes, and Dynamax Theta probes). Proposed advantages for this approach are the possibility for full automation, and with proper set-points, minimization of unnecessary leaching. At USDA-ARS/GA, non-weighing mini-lysimeters will be used to document drainage, and in HI, soil solution sampling suction cups will be installed at 50 cm depth, just below the corn root zone, to monitor leaching. In IA, assimilation of observed soil water data with model-based soil water balance estimates will be investigated. Whereas model calibration attempts to make the observations and model estimates match up by reparameterizing the model, data assimilation updates the model predictions in real time without changing the parameters of the model. Participants utilizing plant-based sensors and approaches to irrigation scheduling will be CA (midday stem water potential), KS (canopy temperature using the Temperature-Time Threshold method, Upchurch et al., 1996), and NY (trunk-embedded microsensors for stem water potential). All participants will record weather data for determination of reference ET, but states emphasizing ET-based approaches to irrigation scheduling will be AL (pan evaporation), HI (IManSys, an Irrigation water Management System), KS, NM, and TX (standard Kc approach), and PR (Penman-Monteith and Priestly-Taylor methods in combination with GOES-derived solar radiation and surface temperature for remotely sensed methods, and Bowen ratio and eddy covariance for ground-based methods). In all states, the range of irrigation levels used will provide empirical data to be used as a basis for the development of grower-appropriate irrigation schedules. Two participants (CA, NM) will be developing a web-based tool for this purpose as part of a recently funded USDA/SCRI proposal (#2008-04985). Data from relatively large field plots will be used to calibrate satellite image-based estimates of canopy ET using the SEBAL program (see http://www.sebal.us/) against other measures of crop water status. Cooperation in this SCRI project will provide an independent measure of crop ET and water status to members of W-2128. Data on yield and other ground-truth information collected as part of W-2128 will improve the ability of the SCRI project to extend the scope of information to additional crops (particularly field crops). Methods for Subobjective 2a (Improved SDI management for row crop production) SDI systems are being used for row-crop production in many areas of the country for various crops. Because crop rotation is a common practice in many agricultural regions for a variety of reasons, several participants (AL, KS, PR, USDA-ARS/Georgia, USDA-ARS/Texas), will conduct research on examining and optimizing crop production when using SDI systems. Alabama (AL) will examine crop production of wheat, soybeans and corn on silt loam and silty clay loam soils in northern Alabama. The focus of this research will be to develop SDI scheduling criteria that optimize crop production and to provide preliminary basic design and management information for expansion of SDI into this region of the state. The potential for using SDI in conjunction with fertigation will be explored. Puerto Rico (PR) will conduct research on dripline depth and irrigation levels on the growth and yield of subsurface irrigated vegetables, grains, and fruits. This variety of crops has large differences in rooting patterns and also sensitivity to water stress so it will be important to be able to plan and design systems that will optimize production for a given producers overall cropping strategy. USDA-ARS/Georgia will concentrate efforts on improving SDI management crop rotations using peanut, cotton and corn. Peanut is the most profitable crop of interest for this effort, but requires annual rotation with other crops. Subsurface dripline placement (under each crop row or between adjacent rows) will be compared for the various crops in terms of crop yield and quality and the resulting economics. Kansas (KS) and USDA-ARS/Texas will continue cooperative research (initiated under both USDA-RRF W-1128 and the USDA ARS Ogallala Aquifer Project) concerning optimizing production of SDI production of various crops (corn, cotton, wheat, soybeans, grain sorghum and sunflowers). In this drier, semi-arid region of the country, some of the focus will be on managing tillage systems and bed designs to improve crop germination and establishment, while other efforts will develop best scheduling procedures for various irrigation levels or irrigation capacities. All four participants (AL, KS, USDA-ARS/Georgia, USDA-ARS/Texas) will compare results from the various studies to develop those management and design procedures that are common between regions and provide rationale for fine-tuning adjustments for specific locales. Methods for Subobjective 2b (Improved emitter maintenance, selection, and design) A key effort spearheaded by CA initiated under the current W-1128 regional project, but to be expanded and augmented by efforts of AZ, KS, and TX, will be to develop a website-based tool for maintenance of microirrigation systems. The tool will use a decision tree approach centering around four issues (prediction of potential problems, determination of an existing problems, solutions to existing problems, and maintenance to prevent clogging problems). Around each of these issues (modules) will be appropriate calculation tools, advisory tables, and/or descriptive publications. It can be noted that this effort also has an interrelationship with Objective 4. Laboratory and field research studies as well as data from previously conducted studies by the participants (AZ, CA, KS, TX) will be used to develop emitter selection guidelines based on water quality aspects (suspended materials, chemical constituents, biological activity). The experimental aspects of these studies will be looking at various levels of filtration and operating pressure as they affect emitter discharge and clogging for different types of emitters and their characteristic parameters (e.g., flow regime, emitter pathway length, width, depth, shape, and elasticity). These studies are difficult and time consuming to conduct, so a strong effort will be made to accumulate and distribute among the participants results from previous studies in addition to the newly acquired data and to develop common indexes or characterization procedures that can be used by end-users to select emitter type. Some of this effort is also closely associated with Objective 4. Methods for Subobjective 2c (Irrigation system comparisons) Several participants (AL, KS, NM, USDA-ARS/CA, USDA-ARS/GA, USDA-ARS/TX) will compare microirrigation system performance to more traditional means of irrigation. Research to compare different types of irrigation systems and to optimize their performance on different lower value commodity crops (e.g., cotton, corn, peanut, grain sorghum, soybean and sunflower) will be conducted by Kansas, USDA-ARS/GA and USDA-ARS/TX. The USDA-ARS/GA will compare non-irrigation, overhead sprinkler (SI) at three levels of ET replacement (100, 66, and 33%), subsurface drip (SDI) at three levels of ET replacement (100, 66, and 50%), and surface drip (DI) at 80% of ET replacement for 6 crop rotations using peanut, cotton and corn. The crop rotations range from continuous peanut to four year rotation with peanut where the rotation is with corn and cotton. The study will include analysis of crop yield and quality components, irrigation amounts, water use efficiency and the resulting economics for the Southeast Coastal Plains. Similarly long term research in KS will compare simulated low energy precision application (LEPA) sprinkler irrigation with SDI on corn, grain sorghum, sunflower and soybean in terms of crop yield and yield components, irrigation amounts, water use efficiency and the resulting economics for deep silt loam soils in the semi-arid Central Great Plains. The three irrigation levels will be imposed by limiting the irrigation capacity to approximately 4, 5 or 7 mm/day but with the need for irrigation being determined by the calculated water-budgets. Kansas will update and continue to develop a Microsoft Excel template to compare conversion to center pivot sprinkler irrigation or SDI for crop production. This template will allow users to input their own cost and performance estimates but will provide suggestions for those items when they are unknown by the producer. The USDA-ARS/TX will compare cotton and corn production under mid-elevation spray application (MESA) sprinklers, low-elevation spray application (LESA) sprinklers, LEPA sprinklers and SDI for a range of irrigation rates. Study parameters will include crop yield and yield components, water use and water productivity and the resulting economics for deep clay loam soils in the semi-arid Southern Great Plains. Irrigation rates will consist of 100, 75, 50, 25, and 0% of full ET replacement, where full ET is measured with neutron scattering to the 2.3 m depth. Additionally, the economic analysis will assess farm profitability for the different irrigation systems for various crops (corn, grain sorghum, soybeans, and cotton) using data from both previous and on-going studies. Research in NM and USDA-ARS/CA will compare different irrigation systems with the goals of optimizing production and minimizing environmental concerns for higher-value vegetable crops. Onion and chile production will be compared in NM under furrow irrigation (FI) and SDI with a special emphasis on evaluating nitrate -N leaching below the root zone. This research will carry out the complete water balance, salt balance, nitrogen balance and determine the leaching fraction, and depth of fertilizer and water pulses and determine the irrigation efficiencies under both methods. The study areas will be instrumented with TDR probes to monitor diurnal variations of soil moisture and soil temperature and monthly soil samples will be collected up to a depth of 1.5 m. The soil samples will be analyzed for soil EC, pH, chloride and nitrate concentration. The USDA-ARS/CA will conduct a study to optimize vegetable production while minimizing non-beneficial water losses under FI, DI and SDI systems by focusing on development and fine-tuning of crop coefficients for vegetables. Water will be applied with each system at four different irrigation levels in order to determine the best application rates for obtaining optimal yield. Amounts of applied water will be equal to either 50, 75, 100 or 125% of the crop evapotranspiration rate determined from water losses in the crop lysimeter. Soil water balance calculations will estimate ETc in the field treatments. To calculate daily crop coefficent values, crop ETc from the crop lysimeter (measured by weight changes) will be divided by reference ETo from the well-irrigated grass lysimeter. Reference values will also be compared to those estimated from meteorological measurements made by the CIMIS weather station located on the grass field. Methods for Subobjective 3a (Development of fertigation recommendations) Fertigation recommendations will be developed based upon placement, formulations and interactions of applied nutrients in the root zone and with irrigation applications. The interaction of microirrigation method (drip and microsprinklers) and N fertilizer type (UN32, Calcium Ammonium Nitrate) on nitrogen use efficiency and greenhouse gas generation will be investigated by CA for tree crops (almonds and pistachios). Serial sampling of plant tissues will be used in conjunction with pulsed 15N labeling to determine the relative rates of N uptake in subsets of trees. The potential for precision fertigation will be examined by CA through the use of soil EC and ion probes to optimize the fertilizer 'positioning' within the tree root profile. As part of the aforementioned USDA-SCRI grant (#2008-04985) CA will relate remotely sensed data to measured water and nitrogen status in pecans, almonds and grapes to improve grower management and to provide increased options if/when there is a drought. Puerto Rico (PR) will examine fertigation and use of slow release fertilizers in avocado fruit trees (avocado and citrus) using two fertilizers application methods and two microirrigation levels scheduled by tensiometers (40-45 kPa or 10-15 kPa). The two fertilizer application methods will be the use of slow release fertilizers (SRF) and fertigation with the same amount of nutrient applied in both cases. The difference is that the SRF will be a one-time application and fertigation will be applied monthly. Vacuum lysimeters will be installed and water samples taken periodically and analyzed for nitrate-N content. Improving kernel set in field corn grown with SDI will be examined by KS. In this study three nitrogen fertilization strategies (all broadcast preplant; one-half preplant and one-half in weekly applications until pollination; or weekly applications until pollination) will be compared under two irrigation levels (50% and 100% of ETc). Corn yield components, crop uptake, water use and water productivity will be measured. Both nitrogen and potassium fertigation with SDI will be examined for cotton production in AL. Five different nutrient timing treatments (combinations of preplant and no preplant applications and total applications within specific growth stages) will be compared in terms of cotton lint yield and quality and plant tissue nutrient analysis. Conjunctive management of water and nutrients will be the focus of VI efforts for cucurbit production. This effort will concentrate on irrigation management factors of frequency and amount with various nutrient sources to optimize production and to minimize environmental concerns. Participants in AL, KS, and TX will work together to develop grower publications related to fertilization for SDI and KS and TX will also work together to provide grower meetings on the topic as part of their Ogallala Aquifer Project commitments. Methods for Subobjective 3b (Agrochemical efficacy and water quality protection) With appropriate BMPs, agrochemicals applied with microirrigation can offer crop yield and quality advantages, as well as potentially reduce risk of negative environmental and off-site water quality effects. Florida (FL) will examine the use of polyacrylamide soil additives for enhancing microirrigation of fresh market tomato grown on coarse sandy soils with the goal of having optimal production while minimizing nitrate leaching below the root zone. Treatments will include placement, amount, and formulation of the soil additives with measurements to include tomato fruit yield and quality, nitrate leaching below root zone, and fertilizer use. The use of composted cattle manure as a pot mix component for production of microirrigated landscape and bedding plants and as a soil additive for plant maintenance in the disturbed soils of urban landscapes will be an addition effort of FL. The goal of this study is to determine if disturbed urban soils which are commonly created from fill and subsurface soil materials, can be modified using an organic soil additive (composted dairy cattle manure solids) to improve the soil quality with respect to water and nutrient retention and quality of plant growth. Efficacy of agrochemicals for pest management through chemigation and in combination with various plasticulture strategies will be evaluated by CO for melon and vegetable production in terms of marketable yield and quality. Some of these agrochemicals for pest management and microirrigation are very soluble, so procedures and guidelines to avoid leaching must be developed. California (CA) will take a different approach to minimizing water quality hazards and maximizing fertilizer efficacy by evaluating different strategies through simulation modeling. A two dimensional computer simulation model (HYDRUS-2D) will be used to determine nutrient distributions (phosphorus, potassium, nitrate, ammonium) and losses around driplines using different fertigation strategies for different types of microirrigation systems and soil types. Methods for Subobjective 4a (Testing and selection of equipment for livestock effluents) Project participants CA, KS, and ID will investigate use of dairy and livestock effluents as microirrigation water sources and will develop recommendations for equipment selection. These types of studies can be difficult, time consuming, expensive, and extremely variable in results so it will be important to pool the results and experiences to develop the best recommendations. Various filters will be evaluated by ID to determine their effectiveness in reducing concentration of phosphorous passing beyond the filter when used with dairy manure effluent. Because P concentrations often limit the overall application of livestock effluents reducing the P concentration can allow for higher use of the livestock effluent and allow for greater N utilization from the effluent. Emitter clogging as affected by additions of dairy manure effluent to irrigation water will be evaluated by CA and ID for a number of emitter types. Drip emitter products commonly used for irrigating tree and vine crops (hard hoses) will be emphasized by CA, but thin-walled, collapsible emitting hoses (drip tapes) will also be tested (ID and CA). A mix of dairy effluent and freshwater will be used as the irrigation water during testing due to nutrient loading restrictions. These CA and ID field research efforts will be combined with previous livestock effluent field research efforts using SDI from KS to improve the hardware selection knowledge base and to develop more generalized criteria for system design when using livestock effluents. Methods for Subobjective 4b (Use and disposal of waters from human sources) The use of municipally-reclaimed water for irrigation of vegetable crops (FL) and minimally treated waste waters for crop and landscape use (AL, TX) will be investigated. Using reclaimed water from human sources saves fresh water for other uses but introduces new issues and hazards. Florida (FL) will use highly-treated municipal reclaimed water to irrigate citrus trees with microsprinklers and will examine the effects of withholding irrigation during different times of the year on tree performance in terms of yield, quality and total soluble solids. A real-time soil moisture controlled wastewater SDI disposal system will be tested on a high clay soil in the Alabama Black Belt soil area (AL). Previous HYDRUS 2D soil water modeling together with field experiment data demonstrated that evapotranspiration is the major pathway for disposed wastewater so efforts will focus on determining BMPs through selection of an appropriate dosing shut-off point with appropriate schedule adjustments to accommodate crop harvest and planting periods. The TX effort will concentrate on research to determine general design, installation, operation and maintenance information that will allow for optimal dosing and onsite wastewater treatment. Methods for Subobjective 4c (System maintenance for low-quality waters) Recommendations for microirrigation maintenance when irrigating with low quality waters will be developed jointly by several of the project participants (AZ, CA, KS, and TX). These waters are a particular challenge due to their high emitter clogging potential. The water hazards also vary appreciably from one locale to another. Thus, it becomes important to pool information from a variety of sources to develop more general guidelines. Efforts will be made to extend this subobjective to a more national scale because microirrigation maintenance questions arise as soon as the microirrigation method penetrates a new area of the country. One method of improving the universality of these recommendations will be through the maintenance website discussed in Objective 2.Measurement of Progress and Results
Outputs
- (Under Obj 1) Coordination of professional meeting technical sessions on microirrigation scheduling (CA, ID, KS and TX)
- (Under Obj 1) Creation or expansion of websites: a) Soil water sensors for microirrigation management (FL, ID, TX, IA); NUTMAN, Web-based nutrient and water scheduling program (CA); Joint water and nutrient remotely sensed data under SCRI #2008-04985 (CA, NM, TX, KS, IA); Plant-based approaches to microirrigation management (CA, KS, NY). Internet delivery of weather station data (KS, TX)
- (Under Obj 1) Development of microirrigation scheduling procedures for specific crops (AL, CA, FL, ID, KS, NM, NY, OR, PR, TX, USDA-ARS/GA)
- (Under Obj 2) Development of SDI management procedures for various crops and prepare publications and presentations (AL, KS, PR, USDA-ARS/CA USDA-ARS/GA, USDA-ARS/Texas)
- (Under Obj 2) Creation or expansion of websites: a) Microirrigation maintenance (AZ, CA, KS, TX); b) SDI-specific web links (KS, TX, USDA-ARS/TX)
- (Under Obj 2) Development of emitter selection guidelines (AZ, CA, KS, TX)
- (Under Obj 2) Comparison and optimization of alternative irrigation systems (AL, KS, NM, USDA-ARS/CA, USDA-ARS/GA, USDA-ARS/TX)
- (Under Obj 3) Development of BMPs to address fertigation (AL, CA, KS, PR, TX, VI)
- (Under Obj 3) Develop procedures for conjunctive use of agrochemicals and microirrigation with focus on water quality protection (CA, CO, FL)
- (Under Obj 3) Publications and presentations at meetings and conferences (KS and TX)
- (Under Obj 4) Publications and presentations regarding selection of microirrigation emitters and system components for use with livestock effluents (CA, KS, and ID)
- (Under Obj 4) Development of microirrigation system maintenance guidelines for irrigating with low quality water (AZ, CA, KS, and TX)
- (Under Obj 4) Planning and participation in SSSA Workshop on Water Reuse (FL)
- (Under Obj 4) Recommendations for use and disposal of waters from human sources (AL, FL, TX)
Outcomes or Projected Impacts
- The technical session will bring together experts representing different approaches to irrigation scheduling and will contribute to a more integrated approach to this field of research and education.
- Websites dedicated to soil- and plant-based irrigation scheduling approaches, nutrient management and delivery of ET-based information will allow growers to improve their crop production and economics while reducing irrigation withdrawals.
- Broader involvement of multiple members of this proposed regional project will extend the applicability of the UCD SCRI website to a broader range of crops.
- A grower-accessible, real-time measure of crop performance will be an invaluable tool as a check against any proposed microirrigation schedule.
- Growers will be able to use the crop coefficient values along with regional ETo estimates to approximate ETc in their fields and then schedule irrigations.
- Improved design and management procedures for SDI will allow broader penetration of microirrigation into non-traditional microirrigation regions of the country.
- Improved emitter maintenance and emitter selection procedures will reduce non-uniformity in microirrigation and allow for greater system longevity.
- Crop losses due to underirrigation resulting from microirrigation system clogging will be reduced.
- Economic losses associated with microirrigation system failures due to maintenance issues will be reduced.
- Comparisons of alternative irrigation systems will allow growers to make the best system choice for their operations and will allow them to optimize performance of existing systems.
- Benefits of microirrigation will be more fully realized through application of improved fertigation practices that will better match fertilizer applications (rates, timing, placement and formulations) to crop requirements.
- End-users will better understand technical issues (chemical compatibility, irrigation system maintenance, etc.) related to agro-chemical use with microirrigation.
- Risks of negative impacts to environment and water quality will be reduced through reduced losses through leaching or other off-site /non-target chemical movement.
- Pest management options and efficacy will be improved and expanded for production systems using microirrigation.
- Concentrated animal agriculture facility operators who use their effluent for irrigation will be able to determine whether microirrigation is a viable option.
- Selection of microirrigation equipment and components for livestock effluents will be easier for producers.
- Growers, scientists, and regulators will have increased knowledge about the safe reuse of reclaimed water.
- The use and disposal of waters from human sources through SDI will reduce health hazards.
- Effluent from septic systems can be applied with SDI in some cases where conventional systems fail.
Milestones
(2010): Present a technical session entitled: ET-, soil- and plant-based approaches to irrigation scheduling: Where are the information gaps? as part of an irrigation-related technical conference. Yield, precipitation, and applied water data from past and ongoing irrigation scheduling experiments are collected and pooled into a common data set. Laboratory studies to calibrate soil water sensors begin. Field studies using various irrigation scheduling approaches for various crops begin. Field studies initiated to determine optimal SDI management for various crops. Presentations made on SDI management using existing information. Begin work on maintenance website decision tree and identify major information and subject matter gaps. Literature review for emitter selection guidelines begins. Field irrigation system comparison studies begin for various crops. Field studies initiated to determine optimal fertigation for various crops. Field studies to examine use of agrochemicals through microirrigation to enhance crop production begin. Field studies to examine use of soil amendments in conjunction with microirrigation to protect water quality begins. Literature review to develop BMPs for fertigation from existing information begins. Modeling efforts to examine nutrient leaching begin. Planning and design of livestock effluent studies begin. Literature review on system components suitable for effluent use begins. Literature review on microirrigation maintenance with low quality water begins. Field studies with onsite human wastewater disposal begin.(2011): Create draft websites for soil- and plant-based approaches to microirrigation scheduling. Create draft website for NUTMAN (nutrient management). Begin joint participation of W-2128 participants in SCRI#2008-04985 project. Update completed of PNW extension publication "Soil Water Monitoring and Measurement" PNW 475. Project report completed for California Dept. of Water Resources on vegetable crop water requirements for irrigated areas of the Central Valley. Project report completed for CIMIS about vegetable crop coefficients. All field studies initiated in 2010 for Objectives 1 through 4 continue. Laboratory calibration studies of soil water sensors are completed and planning for field studies begins. Work begins on all modules for maintenance website and links are developed. Literature review for emitter selection guidelines is completed and national meeting presentation is made. Literature review to develop BMPs for fertigation from existing information is completed and national meeting presentation is made. Modeling efforts to examine nutrient leaching continue. Field studies for use of livestock effluent through microirrigation systems begins. Literature review on system components suitable for effluent use is completed and national meeting presentation is made. Literature review on microirrigation maintenance with low quality water is completed and national meeting presentation is made.
(2012): Open websites concerning soil- and plant-based approaches to microirrigation scheduling and nutrient management for public comment within W-1128 membership. Complete the analysis of the pooled applied water/yield data and develop indices and start writing report. Field calibration studies of soil water sensors begins. All field studies initiated in 2010 for Objectives 1 through 4 continue. Maintenance website work continues. Grower recommendations developed and released for emitter selection guidelines. BMPs for any completed field studies are developed and released. Modeling efforts to examine nutrient leaching are completed and reports are drafted. Grower presentations on selecting system components suitable for effluent use are made. Grower presentations on microirrigation maintenance when using low quality waters are made.
(2013): Finalize all websites
Projected Participation
View Appendix E: ParticipationOutreach Plan
A broad mix of traditional and non-traditional educational mediums will be used in outreach. This will include but will not be limited to field days, tours, demonstration sites, college class seminars, targeted training sessions (e.g. NRCS staff, Consultants), regional, national, and international conferences, newsletters, newspaper and popular press articles, audio CDs and video DVDs, Powerpoint presentations, factsheets, extension bulletins, research publications, refereed journal articles and Internet-based educational material.
Project members will conduct a technical session at a national or international conference during the second year of the project related to microirrigation scheduling approaches. Possible conferences would include, but not be limited to the Irrigation Association (IA), the American Society of Agricultural and Biological Engineers (ASABE), and the Americal Society of Agronomy and Soil Science Society (ASA-SSSA) of America.
Educating agricultural producers is a critical part of the successful implementation of microirrigation technology. However, educating consultants who are often largely responsible for data interpretation is of equal importance. The Project will conduct targeted training sessions for consultants and staff from Cooperative Extension, USDA-NRCS, other state and local agencies.
The Internet can help broaden the availability of information developed in this project and also provides a method of interaction between author and clientele for feedback and clarifications. Project members agree to work towards improving microirrigation technology transfer through Internet-based educational material. A dedicated Internet website site will be maintained at Oregon State University for project results. New Mexico and California will serve as the host institutions for the SCRI project, and Oregon will host the joint websites on soil- and plant-based approaches to microirrigation management and on microirrigation system maintenance. New York will implement an apple-specific ET model at New York State IPM's Network for Environment and Weather Applications web site. Kansas will continue to expand the website, SDI in the Great Plains.
Organization/Governance
The organization and implementation of the project will be in accordance with the "Manual for Cooperative Regional Research."
The Regional Technical Committee will consist of representatives from each cooperating Agricultural Experiment Station and federal agency cooperating in this project. The representative(s) will be appointed by their respective Experiment Station or Research Director. The above will constitute the voting membership of the technical committee.
The Regional Technical Committee will be responsible for the planning and execution of the research project. It will be responsible for coordinating research activities of each cooperating Experiment Station and federal agency and for the developing of appropriate research methods and procedures.
A Director from the Agricultural Experiment Stations of the Western Region appointed by the Agricultural Experiment Station Directors of the Western Region will serve as Administrative Advisor and an ex-officio (non-voting) member of the technical committee. A representative of the USDA Cooperative State Research, Education and Extension Service (CSREES) will serve as an ex-officio (non-voting) member of the technical committee.
An executive committee, consisting of a chair, vice-chair, and secretary will be elected from the voting members of the technical committee. The executive committee will serve one year in each elected office with the provision that the vice-chair will ascend to chair, and the secretary to vice-chair. A secretary will be elected each year. The executive committee will have the authority to act on behalf of the technical committee.
The chair, with the approval of the Administrative Advisor, will notify technical committee members of the time and place of meetings, prepare the agenda, and preside at meetings of the technical committee and executive committee. The chair will also be responsible for naming appointments to subcommittees for specific assignments. The chair will be responsible for annual and final reports. In the absence of the chair, the vice-chair will perform these duties. The secretary will record and distribute the minutes of the meetings.
Literature Cited
Al-Jamal M. S., T. W. Sammis, S. Ball, and D. Smeal. 2000. Computing the crop water production function for onion. Agricultural Water Management 46: 29-41.
Allen, R. G., L. S. Pereira, D. Raes, and M. Smith. 1998. Crop evapotranspiration, guidelines for computing crop water requirements. FAO Irrig. and Drain. Paper 56, Food and Agric. Organ. United Nations, Rome, Italy. 300 pp.
Angelakis, A. N., M. H. F. Marecos do Monte, L. Bontoux, and T. Asano. 1999. The status of wastewater reuse practice in the Mediterranean basin: Need for guidelines. Water Res. 33(10):2201-2217.
Annandale, J. G. and C. O. Stockle. 1994. Fluctuation of crop evapotranspiration coefficients with weather: a sensitivity analysis. Irrigation Science, 15(1): 1-7.
Asano, T. and A. D. Levine. 1996. Wastewater reclamation, recycling, and reuse: Past, present, and future. Water Sci. Tech. 33(10-11):1-14.
Ashley, R. O., W. H. Neibling and B. A. King. 1998. Irrigation scheduling using water-use tables. Univ. of Idaho CIS 1039. 11 pp.
Ayars, J. E., D. A. Bucks, F. R. Lamm, and F.S. Nakayama. 2007. Introduction. Chapter 1 in Microirrigation for Crop Production - Design, Operation and Management. F.R. Lamm, J.E. Ayars, and F.S. Nakayama (Eds.), Elsevier Publications. pp. 1-26.
Ayars, J. E., C. J. Phene, R. B. Hutmacher, K. R. Davis, R. A. Shoneman, S. S. Vail, R. M. Mead. 1999. Subsurface drip irrigation of row crops: a review of 15 years of research at the Water Management Research Laboratory. Agric. Water Mgt. 42:1-27.
Boland, A. M., P. D. Mitchell, P.H. Jerie, and I. Goodwin. 1993. The effect of regulated deficit irrigation on tree water use and growth of peach. J. Hort. Sci. 68(2): 261-274.
Bosch, D. J., N. L. Powell, and F. S. Wright. 1992. An economic comparison of subsurface microirrigation and center pivot sprinkler irrigation. J. Prod. Agric. 5(4):431-437.
Bronson, K., R. Boman, J. Bordovsky, and D. Porter. 2004. Nutrient management of subsurface drip irrigated cotton. Texas AgriLife Res. and Texas AgriLife Ext. Ser., Lubbock, TX. http://lubbock.tamu.edu/cottondvd/content/cottondvd/Fertility/nutmgmtdripirrcot.pdf
Camp, C. R. 1998. Subsurface drip irrigation: A review. Trans. ASAE. 41(5)1353-1367.
Camp, C. R., F. R. Lamm, R. G. Evans, and C. J. Phene. 2000. Subsurface drip irrigation: Past, present and future. In proc. of the 4th Decennial Natl Irrigation Symp., Phoenix, AZ, Nov. 14-16. pp. 363-372.
Coates, R. W., M. J. Delwiche, P. H. Brown, and K. A. Shackel. 2004. Precision irrigation/fertilization in orchards. ASAE Paper Number: 042249. ASAE/CSAE Annual International Mtg., Ottawa, Ontario, Canada, 1 - 4 August 2004.
Colaizzi, P. D., F. R Lamm, T. A. Howell, and S. R. Evett. 2006. Crop production comparison under various irrigation systems. In: Proc. Central Plains Irrigation Conference, Colby, KS., Feb. 21-22, 2006. Available from CPIA, 760 N.Thompson, Colby, KS. pp. 189-207.
Doorenbos, J. and W.O. Pruitt., 1977. Crop Water Requirements. Irrigation and Drainage Paper No. 24, (rev.) FAO, Rome, Italy. 144 pp.
Dougherty, M., J. Fulton, C. Burmester, C. Norris, D. Harkins, L. Curtis, and D Monks. 2008. Sub-surface drip irrigation (SDI) fertigation for site-specific, precision management of cotton. Alabama Cotton Commission 2007 Rep.
Auburn University Precision Ag Portal. Alabama Coop. Ext. Sys.
http://www.aces.edu/precisionag/staticpages/index.php?page=20081217203945319
Dougherty, M., J. Fulton, C. Burmester, L. Curtis, and D. Monks. 2007. Precision fertilization using sub-surface drip irrigation (SDI) for site-specific management of cotton. ASABE Paper No. 072194. Presented at: 2007 ASABE International Mtg., Minneapolis, MN., 17 - 20 June 2007
Dragoni, D. and A. N. Lakso, 2009. An apple-specific ET model. Acta Horticulturae (in press).
Dragoni, D., A.N. Lakso and R.M. Piccioni. 2005. Measuring transpiration in apple trees using heat pulse sap flow gauges calibrated with whole-canopy gas exchange chambers. Ag. Forest Meteorol. 130:85-94.
Dukes, M. D., J. M. Scholberg. 2004. Soil moisture controlled subsurface drip irrigation on sandy soils. Appl. Engr. in Agric. 21(1): 89101.
Entry, J. A. and R. E. Sojka. 2003. The efficacy of polyacrylamide to reduce nutrient movement from an irrigated field. Trans ASAE 46(1): 7583.
Evett, S. R., T. A. Howell, A. D. Schneider, D. R. Upchurch, and D. F. Wanjura. 1996. Canopy temperature based automatic irrigation control. In: Evapotranspiration and Irrigation Scheduling. C.R. Camp, E.J. Sadler, and R.E. Yoder (eds). Proc. International Conf., San Antonio, TX., ASAE, St. Joseph, MI. pp. 207-213.
Evett, S.R., T. A. Howell, A. D. Schneider, D. F. Wanjura, and D. R. Upchurch. 2002 Automatic drip irrigation control regulates water use efficiency. International Water and Irrigation, 22(2):32-37.
Flanagan, D. C., L. D. Norton, and I. Shainberg. 1997. Effect of water chemistry and soil amendments on a silt loam soil Part 1: Infiltration and runoff. Trans. ASAE. 40(6):1549-1554.
Ford, H. W. 1979. A key for determining the use of sodium hypochlorite (liquid chlorine) to inhibit iron and slime clogging of low pressure irrigation systems in Florida. Lake Alfred AREC Research Report-CS79-3. Mimeograph. 5 pp.
Gilbert, R. G. and H. W. Ford. 1986. Emitter clogging. Chapter 3.1 in Trickle Irrigation for Crop Production. F. S. Nakayama and D. A. Bucks, Eds. Elsevier Pubications, Amsterdam. 383 pp.
Gushiken, E. C. 1995. Irrigating with reclaimed water through permanent subsurface drip irrigation systems. In: Microirrigation for a changing world. Proc 5th Intl. Microirrigation Congress. F. R. Lamm, (Ed.), St. Joseph, Michigan. ASAE. pp. 269-274.
Hagedorn, C. 2003. Subsurface drip irrigation for onsite/decentralized wastewater systems. HATCH Project VA-135691.
Hills, D. J. and M. J. Brenes. 2001. Microirrigation of wastewater effluent using drip tape. Appl. Engr. Agric. 17(3):303-308.
Hoffman, G.J., T. A. Howell, and K. H. Solomon. 1990. Management of farm irrigation systems. ASAE Monograph. ASAE, St. Joseph MI. 1015 pp.
Howell T. A. and M. Meron. 2007. Irrigation Scheduling. In: Microirrigation for crop production. Elsevier. (F.R. Lamm, J.E. Ayars, F.S. Nakayama, eds.)
Jnad, I., B. Lesikar, A. Kenimer, and G. Sabbagh. 2001a. Subsurface drip dispersal of residential effluent: I. Soil chemical characteristics. Trans. ASAE 44(5): 1149-1157.
Jnad, I., B. Lesikar, A. Kenimer, and G. Sabbagh. 2001b. Subsurface drip dispersal of residential effluent: II. Soil hydraulic characteristics. Trans. ASAE 44(5): 1159-1165.
Kaleita , A. L., L. Tian, and H. Yao. 2003. Soil moisture estimation from remotely sensed hyperspectral data. ASAE Paper No: 031047. Presented at: 2003 Annual International Mtg. of ASAE, Las Vegas, Nevada. July 27-30, 2003.
Kidder, G. and E. A. Hanlon, Jr. 1997. Neutralizing excess bicarbonates from irrigation water. Univ. of Florida, Coop. Ext. Ser., SL-142. 7 pp.
Lamm, F.R. and C.R. Camp. 2007. Subsurface drip irrigation. Chapter 13 in Microirrigation for Crop Production - Design, Operation and Management. F.R. Lamm, J.E. Ayars, and F.S. Nakayama (Eds.), Elsevier Publications. pp. 473-551.
Lamm, F. R. and T. P. Trooien. 2003. Subsurface drip irrigation for corn production: A review of 10 years of research in Kansas. Irrig. Sci. 22(3-4):195-200.
Lamm, F. R., J. E. Ayars, and F. S. Nakayama (Eds.). 2007. Microirrigation for Crop Production - Design, Operation and Management. Elsevier Publications. 608 pp.
Lamm, F. R., A. J. Schlegel, G. A. Clark. 2003. Development of a best management practice for nitrogen fertigation of corn using SDI. Appl.Engr. Agric. 20(2): 211-220.
Lamm, F. R., D. M. OBrien, D. H. Rogers, and T. J. Dumler. 2008. Using the K-State center pivot sprinkler and SDI economic comparison spreadsheet 2008. In: Proc. Central Plains Irrigation Conf., Greeley, CO., Feb. 19-20, 2008. Available from CPIA, 760 N.Thompson, Colby, KS. pp. 61-70.
Lamm, F. R., T. P. Trooien, G. A. Clark, L. R. Stone, M. Alam, D. H. Rogers, and A. J. Schlegel. 2002. Using beef lagoon wastewater with SDI. In: Proc. 2002 Irrigation Assoc. Tech. Conf. Falls Church, Virginia. The Irrigation Association. Also available at http://www.oznet.ksu.edu/sdi/Reports/2002/MWIAPaper.pdf
Lampinen B.D., K.A. Shackel, S.M. Southwick, B. Olson, and J.T. Yeager. 1995. Sensitivity of yield and fruit quality of French prune to water deprivation at different fruit growth stages. J. Amer. Soc. Hort. Sci. 120(2):139-147.
Leib, B. G., J.D. Jabro, and A. R. Jarrett. 2003. Pesticide movement under drip chemigation: model simulations and field measurements. ASAE Paper No. 032022. Presented at International Mtg. of the ASAE, Las Vegas, NV. July 27-30, 2003.
Leib, B. G., A. R. Jarrett, M. D. Orzolek, R. O. Mumma. 2000. Drip chemigation of imidicloprid under plastic mulch increased yield and decreased leaching caused by rainfall. Trans. ASAE. 43(3): 615-622.
Ley, T. W., R. G. Stevens, R. R. Topielec, and W. H Neibling. 1994. Soil water monitoring and measurement. Pacific NW Pub. PNW 475. 35 pp.
Meyer, J. L. 1985. Cleaning drip irrigation systems. In Proc. Third International Drip/Trickle Irrigation Congress, Drip/Trickle Irrigation in Action, Fresno, California, November 18-21, 1985. pp 41-44.
Morris, M. and L. Schwankl. 2008. The California microirrigation pocket guide - System management and maintenance. National Center for Appropriate Technology. 92 pp.
Neibling, W.H. and W. Weihing. 2004. Using the IDWR "Keep-up and Catch-up" slide rule. University of Idaho CIS 1117. 8 pp.
OBrien, D. M., D. H. Rogers, F. R. Lamm, and G. A. Clark. 1998. An economic comparison of subsurface drip and center pivot irrigation systems. Appl. Engrg. Agric. 14(4): 391-398.
Oron, G., Y. DeMalach, Z. Hoffman, Y. Keren, H. Hartman, and N. Plazner. 1991. Wastewater disposal by sub-surface trickle irrigation. Water Sci. Tech. 23:2149-2158.
Parsons, L. R., T. A. Wheaton, and W. S. Castle. 2001. High application rates of reclaimed water benefit citrus tree growth and production. HortScience 36(7):1273-1277.
Parsons, L. R., T. A. Wheaton, and P. Cross. 1995. Reclaimed municipal water for citrus irrigation in Florida. In: Microirrigation for a changing world. Proc 5th Intl. Microirrigation Congress. F. R. Lamm, (Ed.), St. Joseph, Michigan. ASAE. pp. 262-268.
Phene, C. J., B. Itier, and R. J. Reginato. 1990. Sensing irrigation needs. In Proceedings of the Third National Irrigation Symposium, Oct. 28- Nov. 1, 1990, Phoenix, AZ. ASAE, St Joseph, MI. 761 pp.
Pitts, D. J., D. Z. Haman and A. G. Smajstrla. 1990. Causes and Prevention of Emitter Plugging In Microirrigation Systems. Univ. of Florida, Cooperative Extension Service, Bulletin 258. 12 pp.
Reddy, S., J. Neufeld, J. Gallian, H. Neibling, J. Ellsworth, and S. Gortsema. 2007. Sugarbeet irrigation management using Watermark moisture sensors. University of Idaho CIS 1140. 7 pp.
Sammis, T. W. 1980. Comparison of sprinkler, trickle, subsurface and furrow irrigation methods for row crops. Agron. J. 72(5):701-704.
Schwankl, L., Hanson, B., and T. Prichard. 2008. Maintaining Microirrigation Systems. University of California ANR Publication 21637.
Shackel KA, B. Lampinen, S. Southwick, W. Olson, S. Sibbett, W. Krueger, J. Yeager, and D. Goldhamer. 2000. Deficit irrigation in Prunes: Maintaining productivity with less water. HortScience 35:1063-1066.
Shedd M, M.D. Dukes , and G.L. Miller. 2007. Evaluation of evapotranspiration and soil moisture-based irrigation control on turfgrass. Proceedings ASCE EWRI World Environmental & Water Resources Congress.
Shock, C., K. Kimberling, A. Tschida, K. Nelson, L. Jensen, and C. Shock. 2002. Soil moisture based irrigation scheduling to improve crops and the environment. http://www.cropinfo.net/AnnualReports/2002/Hansen2002.htm
Smajstrla, A. G., S. J. Locascio, D. P. Weingartner, and D. R. Hensel. 2000. Subsurface drip irrigation for water table control and potato production. Appl. Engr. Agric. 16(3):225-229.
Snyder, R.L. 2008. Personal communication. University of California. Davis, CA
Snyder, R.L. and S. Eching. 2003 (revised 2007). PMday.EXL: Penman-Monteith daily evapotranspiration calculator. University of California. Davis, CA. http://biomet.ucdavis.edu/evapotranspiration.html.
Tarkalson, D. D., J. O. Payero. 2008. Comparison of nitrogen fertilization methods and rates for subsurface drip irrigated corn in the semi-arid Great Plains. Trans ASABE. 51(5): 1633-1643.
Trooien, T. P. and D. J. Hills. 2007. Application of biological effluent. Chapter 9 in Microirrigation for Crop Production. - Design, Operation and Management. F.R. Lamm, J.E. Ayars, and F.S. Nakayama (Eds.), Elsevier Publications. pp. 329-356.
Trooien, T. P., F. R. Lamm, L. R. Stone, M. Alam, G. A. Clark, D. H. Rogers, G. A. Clark, and A. J. Schlegel. 2000. Subsurface drip irrigation using livestock wastewater: Dripline flow rates. Appl. Engr. Agric. 16(5):505-508.
Trooien, T. P. 2002. Management of water and biological effluent for crop production in South Dakota. HATCH Project SD00072-H.
Trout, T. J. and H. A. Ajwa. 2003. Application of soil fumigants through micro-irrigation systems. ASAE Paper No. 032021. Presented at: 2003 ASABE Annual International Mtg., Las Vegas, Nevada, USA, 27- 30 July 2003.
Upchurch, D. R., D. F. Wanjura, J. J. Burke, and J. R. Mahan. 1996. Biologically-identified optimal temperature interactive console (BIOTIC) for managing irrigation. U.S. Patent No. 5539637.
Wanjura, D. F., D. R. Upchurch, G. Sassenrath-Cole, and W. R. DeTar. 1995b. Calculating time thresholds for irrigation scheduling. In: Proc. 1995 Beltwide Cotton Conferences, Jan 4-7, San Antonio, Texas. pp. 449-452.
Yang, L. and A. Kaleita. 2007. Understanding spatio-temporal patterns of soil moisture at the field scales. ASABE Paper No: 072108. Presented at: 2007 Annual International Mtg. of the ASABE, Minneapolis, MN., June 17-20, 2007.
Yost, R. 2002. Fate of wastewater effluent used for irrigation on turfgrass landscape in the American Pacific. Project No. HAW00849-1012S.
Zoldoske, D. F. and K. H. Solomon. 1990. Micro-irrigation scheduling and management. Irrigation Notes. CATI, California State University, Fresno, California. June 1990. http://cati.csufresno.edu/cit/rese/90/900606/index.html