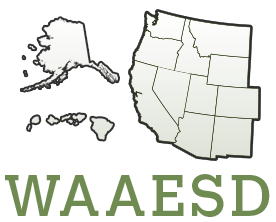
W2147: Managing Plant Microbe Interactions in Soil to Promote Sustainable Agriculture
(Multistate Research Project)
Status: Inactive/Terminating
W2147: Managing Plant Microbe Interactions in Soil to Promote Sustainable Agriculture
Duration: 10/01/2008 to 09/30/2013
Administrative Advisor(s):
NIFA Reps:
Non-Technical Summary
Statement of Issues and Justification
The future of sustainable agriculture in the U.S. will increasingly rely on the integration of biotechnology with traditional agricultural practices. Although genetic engineering promises enhanced yields and disease resistance, it is also important to recognize that plants exist in intimate associations with microorganisms, some of which cause plant disease while others protect against disease. Identifying, understanding and utilizing microorganisms or microbial products to control plant disease and enhance crop production are becoming more central parts of sustainable agriculture. Biological control or biologically-based pest management (BBPM) has the potential to control crop diseases while causing no or minimal detrimental environmental impact. For this proposal, we define biological control as the manipulation of microbial populations through cultural, physical or biological means including plant mechanisms. Some of the benefits of utilizing microorganisms include:
reduced dependence on chemical pesticides, which is important because of expanding demand for organic produce and increasing costs of such petroleum-based inputs;
lack of development of pathogen resistance to biological control organisms, important due to the observed increase in resistance to many chemical controls;
more selective action against pathogens and not against beneficial organisms;
biodegradability of microbial pesticides and the by-products of their manufacture;
reduced danger to humans or animals;
improvement of soil quality and health;
increased food safety;
long term solutions for management of soilborne pathogens;
management of diseases in natural ecosystems;
During the past project period, W-1147 made major contributions towards our understanding of microbial biological control of plant disease. Such understanding is necessary for expanding the adoption of pest management strategies that employ native and applied biological controls.
Demand for biopesticides has continued to expand dramatically in the last five years, and the biopesticide industry is expected to double or triple in the next five to ten years (International Biocontrol Manufacturer's Association, 2004). This expansion is evidenced by the Biopesticide Industry Alliance, established in 2001, which had 31 member companies in 2006. This growth has been driven by expanding organic markets as well as increased public sensitivity to the risks and hazards of chemical pesticides. To date several dozen commercial biocontrol products and processes have been registered by the EPA, over half of these were registered in the last five years. These include fifteen bacterial and seven fungal products, and three activators of plant defenses (http://www.apsnet.org/online/feature/biocontrol/). However, further research is required to overcome problems related to high-volume production, storage, delivery and formulation of such products. With increased demand for biological options, new active ingredients will need to be identified and characterized. In addition, regulatory agencies are increasingly interested in understanding the mode of action of such novel microbials. Therefore, basic research into the physiology and genetics of biocontrol microbes will continue to be needed. More research is also need on how to use these products with integrated pest management (IPM) programs that have been developed for many crops.
This proposed research fits two of the Strategic Goals for 2007-2012 established by CSREES: Strategic Goal 4: Enhance Protection and Safety of the Nation's Agriculture and Food Supply and Strategic Goal 6: Protect and Enhance the Nation's Natural Resource Base and Environment. These two goals are tied together by biological control and biologically based pest management- by reducing our reliance on fungicides and nematicides to manage disease, the environment is enhanced.
Why a Multi-State, Multi-Disciplinary Approach? Because biological control is the result of complex interactions between the agent, the environment, and the pathogen, this research area must be multi-disciplinary and collaborative. No single research institution has sufficient resources and diversity of expertise to solve the diverse disease problems that might be addressed through the use of biological controls. Many of these pathogens occur in multiple states and a coordinated research effort could provide more cost-effective outcomes. Because the results of our efforts are only now beginning to affect U.S. agriculture and the biopesticide industry, continuation of the W-1147 project for another five years will lead to further improvements in the efficacy and adoption of biological controls in American agriculture. In addition, these biological and cultural control techniques need to be tested under a range of environmental conditions and cropping systems that reflect the diversity of U.S. agriculture. The more than 20 researchers in this multistate project also collaborate with researchers in the U.S. and around the world, providing further impact and cross-fertilization of knowledge, as well as conducting the needed outreach activities for implementation of biocontrol options.
JUSTIFICATION:
Economic Costs Due to Soilborne Plant Pathogens
From 2001-2003, an average of 7% to 15% of the major world crops (wheat, rice, potatoes, maize and soybean) were lost due to diseases caused by fungi and bacteria (41).Yield failures resulting from acute diseases such as vascular wilts, take-all of cereals, Phymatotrichum root rot, Verticillium and Phytophthora may be even more severe and have destroyed entire agriculture industries. Detailed studies on the wheat crops in the Pacific Northwest had documented loss of up to 36% due to Pythium, Fusarium, Rhizoctonia, and Pratylenchus (13,17,54,55).
For root diseases of mature crops, there are few effective and economical post-plant strategies for control.
About 90% of the 2000 major diseases of the principal crops in the US are caused by soilborne plant pathogens (30).
Monetary losses due to soilborne diseases in the U.S. are estimated to exceed $4 billion per year (32), and losses due to parasitic nematodes exceed $100 billion per year world wide (5).
Several of the top 15 restricted, invasive quarantine pathogens listed by APHIS are soil borne, and could represent a biosecurity risk.
New invasive species have been discovered in N. America in the last five years, including Phytophthora ramorum, cause of sudden oak death, the potato cyst nematode, Globodera pallida in Idaho and most recently golden cyst nematode G. rostochiensis in Alberta and Quebec. In Nov. 2007, Phytophthora alnus was discovered in Alaska. This pathogen causes dieback of alders in Europe. Ralstonia solaneacearum race 3 biovar 2 has been periodically detected on geranium cuttings in the US, but has not been detected in the field, and would be a serious pathogen of potatoes. The root knot nematode Meloidogyne mayaguensis was first detected in the U.S. in Florida a few years ago, and could overcome genetic resistance to other root knot species. In natural ecosystems, once they become established, these pathogens cannot be easily managed.
Environmental Costs of Soilborne Plant Pathogens
The cost of soilborne plant pathogens to society and the environment far exceeds the direct costs to growers and consumers. The use of chemical pesticides to control soilborne pathogens has caused significant changes in air and water quality, altered natural ecosystems resulting in direct and indirect affects on wildlife, and caused human health problems. For example, methyl bromide, a fumigant used to control soilborne diseases, has become notorious in recent years for contributing to the depletion of the ozone layer. The planned ban on production and importation of this product has been repeatedly delayed by a lack of cost-effective alternatives, and there remains an intensive search for replacement control methods. This fumigant was to be totally banned by 2005, but critical use exemptions for the U.S. resulted in 2007 usage of 29% of the 1991 levels. Several other chemicals could be removed from the market due to regulatory and public concerns. For example, Nemacur (fenamiphos) was taken off the U.S. market this spring, triggered by a regulatory action. Many of these chemicals are now regulated by cities and towns. Additionally, plants evolved in the presence of microorganisms and are dependent on them in order to carry out many activities necessary for growth and reproduction. Thus, long-term chemical applications may permanently alter the microbial community structure sufficiently such that sustainable agriculture may be impossible.
Society's Expectations
As is readily apparent from reading the popular press, consumers are demanding plentiful low cost but safe food while simultaneously requiring the use of fewer chemical controls. From 2000 to 2005, the number of organic acres in the U.S. increased 128%, to around 4 million acres. In 2005, about 5% of vegetable acreage was organic. In the state of Washington, certified organic vegetable production increased 41% in 2006, and organic fruit production is expected to increase by 54% by 2008. The sale of organic milk reached $1 billion in 2005, up 25% from 2004, which has increased demand for organic feed. Organically-grown crops require non-synthetic methods for management of diseases, and organic growers are seeking scientifically-based disease management methods. Recent national surveys (2004) by the Organic Farming Research Foundation have identified pest and disease problems as a major concern for organic growers. Many of our products are certified as organic with the Organic Materials Review Institute (OMRI). During the last few years, more and more pesticides that control soilborne diseases have been taken off the market or regulated, including methyl bromide. Several soilborne diseases, for example, those caused by Phytophthora, Verticillium, Gaeumannomyces, nematodes, Pythium, Rhizoctonia, Fusarium, Streptomyces and Sclerotinia remain major problems after more than 100 years of study. Soilborne pathogens are well adapted to soil conditions, and once established are very difficult to eliminate. Chemical controls are often too expensive to be economically practical and chemicals effective against many pathogens have yet to be identified. Other approaches with great potential include the development of transgenic crops engineered with resistance genes to several pathogens. However, there is widespread public reluctance to accept these crops as evidenced by protests both here and in Europe. This public concern, combined with the natural ability of pathogens to overcome introduced resistance genes, has frustrated efforts to maximize this approach.
The ultimate goals of this collaborative work of W-1147 are to:
Provide society with a safe, low cost food supply.
Reduce the environmental impact of soilborne disease control on ornamental, bioenergy, fiber and food crop production.
Protect natural ecosystems from invasive species
Development of new industries and products for biologically based disease control
Biological Control and Soil IPM Systems As Attractive Alternatives
Biological control is an attractive approach for the control of soilborne diseases (15,16,25,30,60,61,43,62,63,39,40,33,12,27). Advantages of a biological approach to disease control include a lack of environmental damage, reduced human health risks, lack of resistance development in the pathogen, selectivity in mode of action, lack of activity against most beneficial microorganisms, and improved soil conditions and agricultural sustainability.
Biological control of soilborne plant pathogens has made large strides over the past several years. Much of this success is due to activities of the members of W-1147. Today the EPA lists more than 24 commercial biocontrol agents that are registered and commercially available in North America. Nearly all of them have been registered during the past five to ten years. However, most of these products are for seed and seedling diseases. W-1147 project is unique in emphasizing biological control of root diseases of perennial crops, including tree fruits and turfgrass, which are generally not treatable with chemicals or other methods as well as annual crops (Table 2). Since our last renewal, members of the former NC-125 have joined our group, extending expertise to important field crops, including soybean, corn, and alfalfa.
Interest and enthusiasm about biocontrol continues within the science of plant pathology. Since 2000, over 600 peer-reviewed articles have been published on biological control of plant pathogens (Web of Science, Dec. 2007). In fact, two new journals were launched in the 1990s-the journal Biological Control, which covers both arthropod and microorganism-mediated control methods, and Biocontrol Science and Technology. Combined with the increasing resistance in parts of the world to transgenic plants, it appears that the W-1147 regional project is both very timely and successful. Commercial interest has also increased substantially. In the past five years, over a dozen new companies have been formed that develop and market biopesticides. During that time, the EPA has registered over 100 new active ingredients as biopesticides and expects to see a continuation of such development in the years ahead. Indeed, the industry projects a rapid expansion of biopesticide products and a doubling of sales within the next five years.
In spite of the strides made in biological control research and development, there are many areas that require work before biocontrol will be used extensively. Current areas of research include:
Identification of more effective agents. Workers are isolating potential antagonists from soils where many pathogens originated and testing on a range of pathogens.
New bioinformatics information through the genomic analysis of the biocontrol agents and using microarrays to study gene expression in the plant. For example, P. fluorescens Pf5 and Burkholderia ambifaria AMMD have been sequenced since the last proposal, and P. chlororaphis 30-84, P. fluorescens Q2-87, and Q8r1-96 are currently being sequenced.
Advances in metabolomics and proteomics are also being used to study the biochemical pathways and in-situ detection of antifungal metabolites produced by biocontrol bacteria
Understanding the genetic diversity of pathogens, biocontrol agents and beneficial microbes, using advances in DNA sequencing, such as pyrosequencing.
Identification and characterization of natural disease suppressive soils.
Development of lower cost production, storage, and distribution systems.
Improved quality control assays.
Improved stability of the agent during production, storage and application.
Integration of biocontrol into current agronomic practices.
Identification of parameters affecting efficacy and survival after application.
Understanding the mechanisms of action of control, especially at the molecular and biochemical level.
Mycofumigation-(production of organic antimicrobial volatiles with activity against fungi.bacteria and nematodes)
Investigation of manipulation of cultural parameters that advance biological control (eg. uses of compost, green manures, and rotation crops).
Understanding the role of the plant in biological control (vis-a-vis induced resistance pathways).
Microbial community and plant-microbe interactions with biocontrol agents.
Use of microbial products or metabolites and other biorational approaches, such as compost teas, plant strengtheners, etc.
The promise, public acceptance and environmental benefits of non-chemical management of root diseases continue to make research on this area both timely and of critical importance to the future of U.S. and world agriculture.
Clearly there is much to be done in order to improve biocontrol agents so that they will continue to become major factors in the control of soilborne diseases. Biocontrol agents isolated by participants of W-1147 at ARS-WA, ARS-CA, CA-R, OR, MT, AK, OH, NB and NY have the ability to suppress a wide variety of plant pathogens that cause serious diseases of food, fiber and ornamental crops. The need for "high quality" biocontrol agents has never been more critical because of the pending loss of fungicides and fumigants upon which agriculture has been dependent for the last 50 years. Understanding the complex biological and environmental interactions that must occur for biocontrol to be effective requires the combined efforts of multiple investigators at multiple institutions focusing on different aspects of the problem, from applied to basic research. This logical approach is an area in which the W-1147 regional project has excelled and will continue to depend on during the next five years.
This project also fits the goals of other CSREES initiatives, including the National Integrated Food Safety Initiative of 1998, and other programs, such as Integrated Pest Management (IPM), Integrated Organic Program, Methyl Bromide Transition Program (MTB), Pest Management Alternatives Program (PMAP) and Sustainable Agriculture Research and Education Program (SARE). Other programs with similar goals include Risk Avoidance and Mitigation Program (RAMP), Crops at Risk (CAR), Minor Crop Pest Management (IR-4), Organic Transitions Program (OTP), and the Western Regional IPM Program.
The need for this project has become even greater in the last few years, given the elimination of the NRI program on Biologically-Based Pest Management in 2004 that has left many biocontrol researchers with reduced or eliminated funding, and this research has not been funded by other programs. W-1147 and S-1028 met jointly in Oct. 2006 with the National Program Staff at CSREES in Washington D.C. to discuss this problem and possible solutions.
Related, Current and Previous Work
There are a number of reviews on the use of biocontrol organisms to control soilborne plant pathogens (3, 14, 15, 16, 25, 25a, 25b, 30, 50, 60, 61, 62, 63, 43, 19, 39, 40. The EPA currently lists more than 25 biocontrol agents that are registered or pending registration with the EPA and available for commercial use in the U.S. (http://www.oardc.ohio-state.edu/apsbcc/ click on products list)
Identification of New Biocontrol Agents
A list of 44 biocontrol agents which have reduced disease are listed by Cook and Baker (1983); however, many more are likely to exist. One promising approach is to search for new and better biocontrol agents. Most of these efforts involve isolating and selecting biocontrol agents on growth media and testing in the laboratory or the greenhouse. Few are looking for biocontrol agents in foreign lands where pathogens may have evolved. Few are isolating slow growing or non-cultivable biocontrol agents using the pathogen as bait. Few are using methods that detect non-culturable organisms as an initial screen in searching for new biocontrol agents. Estimates suggest that previous isolations have yielded only a tiny fraction of the microorganisms that exist in soils and on plant surfaces. For example, it is estimated that there are over 1.5 million species of fungi that have not been described (23). Thus, we have barely scratched the surface in our hunt for biocontrol agents. W-1147 members have been instrumental in discovering many of these new biocontrol agents, including strains of Pseudomonas fluorescens with superior colonizing ability (Q8R1-96) (52), diacetylphoroglucinol-producing strains of P. fluorescens (51), Trichoderma atroviride 901C (36); Pseudomonas aureofaciens AB254 (33); Rozella sp., and Hyphodontia alutacea, (Menge, unpublished); Dactylella oviparasitica (44) for control of cyst nematodes ) Since our last proposal, this list has been expanded to include Muscodor albus (21, 57a), Lysobacter enzymogenes (65), Bacillus and Paenobacillus spp. (20), Myxobacteria (Myxococcus spp.) (10), Burkholderia ambifaria, Pochonia chlamydosporia (Borneman and Becker, unpublished), and Tetracladium (Borneman and Becker, unpublished). Other biorational products have evolved from biocontrol research. For example, rhamnolipids are biosurfactants produced by Pseudomonas aeruginosa, which lyse zoospores of Pythium and Phytophthora. This research has led to a commercial EPA-registered product (ZonixTM). The bioactive cyclic lipopeptide orfamide A, was discovered using a genomisotopic approach from the sequenced genome of P. fluorescens Pf-5 (31). Tables 5, 6, and 7 in the Attachments outline some of the biocontrol agents and strategies that are being developed and researched by members of W-1147.
Disease Suppressive Soils and Plant Protecting Microorganisms
Over the last 15 years there have been surprising and exciting discoveries for natural methods to suppress or eliminate pathogens, and/or protect plants. Intensive studies of disease suppressive soils have led to the development of new methods of analysis (22, 9, 7, 4) and new insights into the nature of soilborne disease suppression (61, 24). Many of these advances have been made by members of W-1147. Such advances indicate that active management of soil microbial communities can be an effective approach to develop natural suppression of soilborne diseases and improve crop productivity (35). Generally speaking, there are two approaches to actively managing crop-associated microbial communities.
The first approach is to develop disease suppressive soils through manipulation of carbon inputs. This involves adjusting the types and timing of organic inputs, such as cover crops, animal manures, composts, compost teas, and crop sequencing. Such approaches have been shown to provide site-specific reductions in disease and pest incidence (1, 11, 64, 53, 58, 18, 29). The advantage of this approach is that it relies on locally available resources to maximize soil health in a sustainable way. The disadvantage is that outcomes tend to be more variable depending on soil type, so knowledge of soils and available inputs is essential.
The second approach involves inoculation with disease suppressive microorganisms. All soils harbor detectable populations of disease suppressive organisms, however, the diversity, relative abundance and activities of these organisms can vary substantially from site to site. Historically, researchers recovered microbes and then screened them for disease suppressing activity. However, molecular tools now allow us to identify microbes that are suppressive in situ and recover them in a directed fashion (9). Such an approach has already proven useful at collecting indigenous fungi that acted as effective inoculants to suppress soilborne diseases caused by nematodes (44). In addition, analyses of the genetics and genomics of disease suppressive microbes (45, 2, 26) have led to new methods to isolate disease suppressive microbes in a directed fashion from any location. This approach too, has led to the development of effective and low cost inoculants (38).
Methods that transform resident microbial communities in a manner which induces natural soil disease suppression have potential as components of environmentally sustainable systems for management of soilborne plant pathogens to reduce the need for pesticides.
Mechanisms of Disease Control
Biological control agents express a variety of mechanisms that are responsible for pathogen inhibition. Therefore, if we are to maximize the effectiveness of any biocontrol agent, we must understand the function of the mechanism in the biocontrol agent's lifestyle. Known mechanisms by which biocontrol organisms reduce disease include:
Induction of plant resistance mechanisms.
Antibiotic and toxin production.
Cell-wall degrading and lytic enzymes.
Siderophore production.
Biosurfactant production
Mycoparasitism
Mycofumigation (production of organic antimicrobial volatiles with activity against fungi, bacteria and nematodes)
New mechanisms and chemistries are being discovered by genomic analysis.
Several of these mechanisms were identified only recently by members of the W-1147 project. These include the role of biofilms (66, 56), the genetic and biochemical pathways for production of phloroglucinol and phenazine, (47, 37), the regulation of phenazine production (34), the role of phloroglucinol producers in suppressive soils (51) and the in situ detection and quantification of antifungal compounds produced by biocontrol agents in the soil and rhizosphere (59). There are certainly many more mechanisms as yet undiscovered. Furthermore, although the mechanisms are known for some biocontrol agents, these agents do not control disease efficiently. This suggests that we do not yet understand the effects of nutrients, environment and growth stage on the control mechanisms. For instance Pierson and Pierson, (46) have recently shown that environmental factors and other organisms regulate the amount of phenazine antibiotic produced by the biocontrol bacterium, Pseudomonas aureofaciens. This means that it is not enough to understand that phenazine production is the mechanism for biological control, but we must understand: when it is produced, where it is produced, why it is produced, how much of it is produced, which environmental factors affect its production and what influence the indigenous microflora has on its production. A number of unknown "antibiotic islands" were detected in the genome of P. fluorescens Pf-5, which led to the discovery of the bioactive lipopeptide orfamide A (31). A screening of the bacterium Lysobacter enzymogenes strain C3 led to the discovery of HSAF (heat stable antifungal factor). The structure of this compound has been determined to be a dilrydromaltophilin, a unique macrocyclic lactam with a tetramic acid moiety (65). This group of compounds could be exploited for fungicides and antifungal drugs. The endophytic fungus Muscodor albus, originally isolated from the cinnamon tree in the tropics, produces volatile organic compounds that kill or inhibit other fungi, bacteria, or nematodes (21, 57a), and is being currently commercialized by Agriquest, a company in California.
These examples serve to illustrate the point that every biocontrol agent-plant pathogen-host crop system requires special insight on how best to utilize the biocontrol agent to maximize disease control. This maximization of biocontrol will be different for different regions of the United States.
In summary, research related to the objectives outlined above is in progress throughout the world. However, the lack of broad acceptance of biocontrol agents reflects two major obstacles. First is the complexity of the ecological systems in which the biocontrol agents must operate. Second is the current mindset of growers that biocontrol is expensive and inconsistent. To overcome both hurdles, we must better understand the factors that influence the efficacy of biocontrol agents once released in the field in order to begin to manipulate the system as a whole and maximize the benefits of biocontrol and its contribution to sustainable agriculture.
Differentiation from other regional workgroups
Currently only one other regional workgroup, S-1028, is focusing on various aspects of microbial biological control of soilborne plant pathogens. The Southern group, S-1028, is focusing on testing of commercial and near-commercial seed and plant treatments, as well as the genetic diversity of the soilborne pathogens and indigenous microbes. The W-1147 project has focused on characterizing new biocontrol agents, understanding the biological mechanisms of naturally suppressive soils, and applying these agents in agriculture.
Each project utilizes a different approach to attain their objectives. Research conducted under W-1147 involves a greater emphasis on understanding the mechanisms involved in plant-microbe interactions. In addition, W-1147 research is unique in that biocontrol organisms and approaches must be customized for a more arid climate than that of the other regions. W-1147 project is unique in emphasizing biological control of root diseases of mature crops, which are generally not treatable with chemicals or other methods. The combination of approach, crops, pathogens, cropping systems and biocontrol agents distinctive to W-1147 provides a unifying theme that facilitates progress toward the objectives. Differences among the two regional projects will serve as a benefit by allowing these groups to compare results across the U.S. during the joint meetings held every 3 years.
Table 1 in the Attachments shows the differences in objectives between the two projects, and Table 2 provides a comparison of the disease systems and hosts to be investigated by members of the two regional projects. It is apparent even where there is overlap of host crop and pathogen, different research approaches and objectives differentiate the projects. Again, regional, soil, climate and farming practices also separate what appear to be similar projects.
Research leaders, area of specialization and resources are listed in Table 3. The responsibilities of the states with respect to soilborne plant pathogens and objectives addressed are shown in Table 4.
Objectives
-
To identify and characterize new biological agents, microbial community structure and function, naturally suppressive soils, cultural practices, and organic amendments that provide control of diseases caused by soilborne plant pathogens.
-
To understand how microbial populations and their gene expression are regulated by the biological (plants and microbes) and physical environment and how they influence disease.
-
Implement sustainable management strategies for soilborne pathogens that are biologically based and compatible with soil health management practices.
-
Provide outreach, education, extension and technology transfer to our clients and stakeholders- growers, biocontrol industry, graduate and undergraduate students, K-12 students and other scientists.
Methods
Objective 1: To identify and characterize new biological agents, naturally suppressive soils, cultural practices, and organic amendments that provide control of diseases caused by soilborne plant pathogens. A. Assessing isolated microbes for biocontrol activity. In the past, extensive efforts were made to isolate microorganisms at random from soil and plant material and then identify, through in vitro, greenhouse and field tests, those with potential as biological control agents. This strategy tended to yield organism species that occur in high populations or those that grow quickly in culture. While such "prospecting" is still important in the search for new biocontrol agents, isolation and assessment efforts in this project will be more directed. Studies on suppressive soils and agricultural practices described below will likely indicate microorganism taxa that have not been investigated previously in the context of disease control. Experiments in controlled and field environments will focus on representative strains of these new taxa to determine their efficacy. Tables 5-7 show some of the biocontrol agents that are being investigated by W-1147.B. Examining naturally suppressive soils. Suppressive soils hold considerable potential for managing soilborne pathogens. Such soils have been defined as "soils in which the pathogen does not establish or persist, establishes but causes little or no damage, or establishes and causes disease for a while but thereafter the disease is less important, although the pathogen may persist in the soil" (Cook and Baker, 1983). When the suppressiveness has a biological origin, the crucial steps in realizing this potential are to identify the causal organisms and then to understand the agronomic and environmental factors that enable them to function. Armed with such knowledge, it may be possible to develop effective and sustainable pest management strategies through the application of the organisms and through agronomic practices that influence their populations.
To identify microorganisms involved in pathogen suppressiveness, we frequently employ a population-based approach. Population-based investigations of suppressive soils can be performed by examining the microbial community compositions in soils possessing various levels of suppressiveness. Microbial communities can be examined using a variety of culture or culture-independent methods. Different levels of suppressiveness can be created by manipulating the microbial communities with physical, chemical and biological methods such as heat treatments, antimicrobial agents, and nutritional or microbial amendments. Alternatively, naturally occurring soils with different levels of suppressiveness can also be utilized. The relative abundance of each taxon can then be associated with levels of suppressiveness. Taxa exhibiting the strongest correlations will represent organisms putatively involved in the suppressiveness.
Members of our workgroup have successfully employed this strategy in several systems. A few examples include Borneman and Becker's (CA-R) work, which identified fungi involved in soils that naturally suppress two important plant pathogens: sugarbeet cyst and root knot nematodes. Paulitz (ARS-WA) has documented the influence of agricultural practices on Rhizoctonia suppressiveness and characterized shifts in bacterial communities associated with such practices. McSpadden Gardener (OH) has recently discovered bacteria associated with general disease suppression arising from the application of different organic field management strategies.
One major advantage of investigating microorganisms associated with suppressive soils is that these organisms have demonstrated the ability to function in real agricultural systems. We will continue to identify and characterize such organisms towards the development of more sustainable and effective strategies to manage soilborne pathogens.
C. Examining cultural practices that influence soilborne pathogens. Documenting the effects of soil management practices on the incidence and damage of root diseases will lead to the identification of suppressive soils and the design of soil management strategies that are also effective in suppressing soilborne pathogens. In addition, characterizing the role of cover crops and their incorporated green manures against soilborne pathogens will contribute to the development of culturally and biologically-based management options for root diseases.
Towards this goal, members of our W-1147 workgroup are participating in a collaborative and multidisciplinary project termed the Cornell Soil Health Program Work Team (PWT). The goal of the Cornell Soil Health Project Work Team (PWT) is to address the progressive deterioration of agricultural soils and provide information that will aid growers in the assessment and management of their soils. The PWT is also interested in developing a proper management strategy to maintain high quality productive soils without measurable deterioration. The project seeks to incorporate a broader range of soil tests to provide greater information to growers, identify soil health problems and means of addressing them, as well as to create a database by which to evaluate soil test results. Members of the W-1147 workgroup have performed numerous experiments examining how agricultural practices influence soilborne pathogens. For example, soil samples collected from various grower fields were assessed for their general suppressive capacity to soilborne pathogens (root health) using a soil bioassay with bean. In general, root health was improved with the implementation of reduced tillage, long rotations with grain crops, certain cover crops and organic amendments. Researchers (ARS-WA) have explored how tillage affects root diseases, and how they can be managed with crop rotation, fallow, and proper herbicide application to control reservoirs of inoculum from crop volunteers and weeds. They have also initiated research characterizing the microbial communities from these suppressive soils and to assess the mechanism(s) involved. In addition, they will continue their evaluations of green manures, plant activators, and available biological control preparations against soilborne pathogens and their damage to vegetable crops. Table 8 in the Attachments shows W-1147 efforts in the area of suppressive soils and cultural control.
OBJECTIVE 2. To understand how microbial populations and their gene expression are regulated by the biological (plants and microbes) and physical environment and how they influence disease.
Previous cooperation among W-1147 members has led to the identification of several new mechanisms of disease control. Environmental and biological factors in the field affect both the populations of biocontrol agents and the expression of genes within the biocontrol agents, which are responsible for disease control, and must be understood in order to improve the consistency of biocontrol.
The following is a brief list of some of these projects and participants.
Induction of plant resistance mechanisms. This includes both systemic acquired resistance (SAR) and induced systemic resistance (ISR). Researchers in NY and MT are looking at commercial plant inducers, testing them in the field, and looking at plant defense response gene expression. Researchers in NE are looking at ISR induction by Lysobacter. Researchers at ARS-WA are looking at how antifungal compounds such as phloroglucinol induce resistance in Arabidopsis. Microarray technology is being used to determine what genes are stimulated by colonization with Pseudomonas (ARS-WA)
Antibiotic and toxin production. Researchers in ARS-WA continue to investigate the role of phenazine and phloroglucinol in suppressing soilborne pathogens. They have elucidated the 3-dimensional structure of enzymes in the phenazine biosynthesis pathway, and found a unique group of phenazine producers in eastern Washington that may be involved in suppression of Rhizoctonia. Researchers at OR have discovered a new class of lipopeptides (orfamide A), based on mining the genomic sequence of P. fluorescens PF-5. A new class of antibiotics (HSAF-heat stable antifungal factor), a unique macrocyclic lactam with a tetramic acid moiety, has been discovered by researchers in NE, and may lead to new natural products. Researchers in OH are examining the diversity of cyclic lipopeptide synthase genes.
Biosurfactant production- Researchers at CA-R have identified rhamnolipids that are produced by Pseudomonas in hydroponic systems, which lyse zoospores of Pythium and Phytophthora.
Mycofumigation (production of organic fungistatic volatiles). Muscodor albus produces volatile organic compounds which inhibit a wide range of pathogens, both soilborne and postharvest (MT, CA).
Genomic sequencing and analysis. The first genome sequence of a biocontrol agent was accomplished by members of W-1147 (P. fluorescens Pf-5). This has led to a new paradigm of discovery of mechanisms, based on "mining" the genome and looking for undiscovered chemistries and enzymes. This effort continues with researchers at AZ, ARS-WA, and OR, who are involved in sequencing projects of three isolates of Pseudomonas.
How bacteria colonize the rhizosphere. Workers at ARS-WA are using subtractive hybridization to identify genes involved in superior colonization by isolates of Pseudomonas. They have shown how different genotypes have different preferences for different hosts and rotation crops. ARS-WA has discovered Type III secretion systems in biocontrol Pseudomonas spp. This is a major determinant of pathogenesis in pathogenic species, where the bacterium can inject effectors into the cell of the host. However, the role of this system in biocontrol bacteria remains unknown.
How bacteria interact with other microbes- Cross-talk and quorum sensing among Pseudomonas strains (AZ), and how different genotypes compete on the root system (ARS-WA), are just two examples.
Regulatory systems in biocontrol agents- There is a major focus by OR, AZ and ARS-WA to understand the regulatory mechanisms utilized by biocontrol agents to control the expression of genes encoding products involved in disease suppression. For example, because antibiosis is involved in the efficacy of many bacterial biocontrol agents, researchers will be focusing on the regulation, biosynthesis and mutations of the pathways leading to antibiotic production.
OBJECTIVE 3: Implement sustainable management strategies for soilborne pathogens that are compatible and integrated with good soil health practices.
Intensive production of agronomic crops has contributed to gradual deterioration of soil health, resulting in reduced yield and profitability. Characteristics of deteriorated soils include crusting, compaction, low content of organic matter, the increased incidence and damage of root diseases caused by soilborne pathogens, and the prevalence of other pests. In addition, it is well documented that damage due to diseases caused by soilborne pathogens is greater in poor quality and unhealthy soils. Thus, there has been an increasing interest in addressing soil health constraints in a holistic, sustainable, and environmentally compatible manner. Among the long-term management practices promoted to maintain and/or improve soil health status are various reduced tillage systems, more diverse crop rotations, numerous cover crops, green manures and the application of composts. All these soil and crop management practices have profound direct and indirect effects on the populations and disease potential of soilborne pathogens as well as the diversity and dynamics of the soil microbial communities. The increased implementation of soil health management practices; specifically reduced tillage systems are impacting many of our current recommendations and strategies for controlling plant pathogens. Thus, there is a great opportunity and a challenge for scientists associated with W-1147 to become involved in the multi-disciplinary investigations dealing with soil health management to assure the development and implementation of soil health management programs that are also suppressive to soilborne pathogens and plant diseases in general.
Directly or indirectly, all members of W-1147 will be involved in this objective (AK, AZ, ARS-WA, CA-R, MD, MI, MN, MT, NE, NM, NY, OH, OR, and WA). Results obtained from the first two objectives of this proposal, results accumulated from previous W-1147 investigations and the wealth of experience of the participating scientists will be utilized to evaluate, fine-tune and implement ecologically-based control options against soilborne pathogens as integral components of long-term and sustainable soil health management programs. The latter will be possible by assessing the impact of soil health management practices and the mechanism(s) involved in soilborne pathogen control. It will also be crucial to educate growers on how to augment the promoted soil management practices with previously identified microbial biocontrol agents or products applied to seeds or planting mix, how to enhance native soil suppressive communities by modifying cropping sequences, and the best approach to applying appropriate composts and/or the use of selective and low risks chemical products. It is expected that the management programs which have been developed for, and are effective in one location might be different than those needed or effective at other sites due to the differences in the occurrence of major soilborne pathogens, main crops grown/production systems, soil types, and/or environmental conditions. Thus, close collaborations among the involved scientists in this project remain critical and are much needed for the success of the regional project. In addition, the development and validation of accurate and cost-effective diagnostic protocols such as DNA oligonucleotide arrays, which have been developed by several W-1147 members, are essential. These arrays enable the detection and identification of the major root pathogens present on a farm, and provide the information necessary to select the appropriate management options.
The above multi-faceted research and demonstration activities proposed under this objective will be conducted in large experimental plots and in commercial fields of collaborating growers. The latter will promote adoption of the effective management strategies and also enhance multi-disciplinary collaborations. The successful completion of this objective will contribute to greater root health (reducing damage caused by soilborne pathogens), improving soil health and productivity, and reducing environmental risks; thus contributing to agricultural sustainability.
Objective 4. Provide outreach, education, extension and technology transfer to our clients and stakeholders- growers, biocontrol industry, graduate and undergraduate students, K-12 students and other scientists.
1. Outreach and education to growers. Over 50% of our members have extension appointments and regularly meet, consult, and teach growers, pest control advisors, industry representatives, as well as with members of the general public. This outreach will be in the form of field days, workshops, and grower meetings. We publish extension publications and pamphlets, including IPM guides. Our members publish chapters in the disease compendium series of the American Phytopathological Society (APS) Press. Results of biocontrol trials are published in the series Biological and Cultural Tests. Some specific web-based examples are contributions by members to the Pacific Northwest Plant Disease Control Handbook, University of California IPM series, UC IPM Crop Management Guidelines (http://www.ipm.ucdavis.edu/PMG/crops-agriculture.html), High Plains IPM Guide, and the 2007 Integrated Crop and Pest Management Recommendations from Cornell. We provide diagnostic services through the local and National Plant Disease Diagnostic Networks. A very innovative outreach has been constructed by our members at Cornell University- The Cornell Soil Health Website ((http://soilhealth.cals.cornell.edu/). Growers can send in samples to have soil health evaluated and access the Soil Health Manual. Members have contributed to an Organic Strawberry Production manual published by UC Press. Another innovative example is the Phytophthora E Course, being designed by members from OR for growers and nursery workers for best management practices to eliminate Phytophthora. Members also provide training for Certified Crop Advisors and pesticide credits for pesticide applicators. This training increases the level of safety and reduces overuse of fungicides.
2. Outreach to the biocontrol industry and agro-industry. Many of the products developed by our group are being marketed by the biocontrol industry. This includes products such as Root Shield and Plant Shield, Trichoderma products developed by members in NY. Our members facilitate this technology transfer through patents and other IP protection vehicles with their respective agencies. We will continue our liaisons and cooperation with the agroindustry. For example, our members from OH have organized symposia with speakers from industry to show scientists the state of the market. We will invite (a) member(s) of private industry to join W-1147, and will continue cooperation with the Biopesticide Industry Alliance.
3. Training the next generation of biocontrol scientists and practitioners. Almost all of our members have teaching responsibilities, both at the graduate and undergraduate levels. They teach courses in biological control and plant pathology, train students in the lab and supervise graduate students. Many undergraduate students do special or honors projects in our labs. We train and mentor postdoctoral research associates in our labs and research stations. Finally, we have extensive international collaborations, and travel to other countries to give seminars, and host international scientists in our labs. Some of our members (NE) also teach in long-distance web-based courses.
4. Public education to general community and grades K-12. Our members participate in science fair judging, Master Gardener Training, 4-H and FAA. Members from ARS WA have taught science modules at grade and middle schools and science summer camps for underrepresented groups on the Colville Indian Reservation. In OH, annual presentations on biocontrol are made to K-12 students at the OARDC's Plant Science Days. A member from NY has developed an elementary science education program in which she interacts with over 200 third and fourth-grade students each year. Additionally, she runs a five-week summer science camp (four days per week) for elementary school students that focuses on plant science, agriculture and sustainability. More information can be seen at http://www.nysaes.cornell.edu/pp/faculty/smart/pdf/SeedsOfScienceImpact.pdf
5. Providing information to policy makers at the local, state and national level. In the past four years, members of W-1147 were involved in the development of microbial sequencing priorities list assembled by APS and have contributed to materials prepared by the APS Public Policy Board. More recently, our group met with CSREES National Program Staff in 2006 to discuss biocontrol funding and priorities. A member from AK serves the USDA on the Advisory Committee on Emerging Markets.
6. Publishing results in peer-reviewed scientific journals. Finally, we must transmit our findings and results to our peers. W-1147 has an excellent publication record, which will continue. In the last 4 years, we have listed over 250 publications by our members.
Measurement of Progress and Results
Outputs
- Outputs: Success of the project will be measured via publications, biocontrol products, and advancing our understanding of biological control leading to adoption by growers .
- Outreach and education outputs will be presentations to growers, students, and the public; websites, extension publications, teaching materials
- Additional outputs will be new biological control approaches: new organisms, cultivation systems, or formulations.
Outcomes or Projected Impacts
- Projected Impacts: The impacts of the proposed work will be substantial: economically, socially, and environmentally. New biobased products will be developed for the biopesticide industry, a growing industry composed of small-medium sized companies. Disease losses will be reduced, making growers more economically viable. Disease management options will be available for the increasing number of organic growers, who have few options at present. The results of the work will benefit growers, consumers, and the environment by making significant progress in producing low cost safe agricultural products. By our extensive outreach and education efforts, growers will adopt biologically based pest management strategies, leading to improved productivity and sustainability. Finally, there will be enhanced public awareness of biologically based pest management in our agroecosystems.
- The real impact will be adoption of control options by the growers. We want to create an expanded tool kit for organic producers, leading to improved productivity and sustainability.
- We also want to impact and advance the science- knowledge of mechanisms and the genomic and biochemical diversity of biocontrol agents, which enable them to function in agroecosystems.
- Finally, another impact should be enhanced public awareness of biologically based pest management in our agroecosystems.
Milestones
(0):eral milestones: Objective 1: Years 1-2- identify suppressive soils, Years 2-4 - identify suppressive microbial communities with DNA based techniques and Years 4-5, complete Koch's postulates.(0):ective 2: Years 1-2- sequence bacterial genomes, Year 2-4- annotate sequences, Years 3-5- mine for novel sequences.
(0):ective 3: Year 1- select research and demonstration sites, Year 2-5- adjust management practices, Year 4- determine cost-benefits, and Years 4-5- promote management strategies.
(0):ective 4: Year 1- develop Phtyophthora E-course, invite industry participation in group. Years 2-5, develop and implement disease management guidelines for organic producers.
(0):e details are provided in tabular format in Table 9 of Attachments.
Projected Participation
View Appendix E: ParticipationOutreach Plan
The outreached plan is covered in detail in Objective 4 above.
Organization/Governance
The W-1147 regional research program will be administrated by a technical committee consisting of a project leader from each of the participating states. Officers of the committee will be the Chairman and Secretary. The Secretary will be elected each year and will advance to Chairman the following year. For 2007-08 the committee officers will be Chairman- G. Yuen. Secretary: Jianjun Hao.
The projected membership is outlined in Table 3 of Attachments.
Meetings will be called each year by the administrative advisor, and a local arrangements coordinator will be determined for each annual meeting. At those meetings research accomplishments will be reviewed and recommendations made for coordination and publication of results.
Several new members have been recruited into the project since the last renewal- Gary Yuen (NB) Brian McSpadden-Gardener (OH) Chris Smart and Gary Harman (NY) Soum Sanago (NM) and Barry Jacobsen (MT). New members were recruited for this proposal, including Tim Widmer, Lindsey DuToit, Linda Kinkel, Darin Eastburn, Mark Mazzola and Donald Kobayashi.
Literature Cited
1. Abbasi, P. A., Al-Dahmani, J., Sahin, F., Hoitink, H. A. J. and Miller, S. A. 2002. Effect of compost amendments on disease severity and yield in organic and convention tomato production systems. Plant Disease 86:156-161.
2. Bangera, M. G. and Thomashow, L.S. 1999. Identification and characterization of a gene cluster for synthesis of the polyketide antibiotic 2,4-diacetylphloroglucinol from Pseudomonas fluorescens Q2-87. Journal of Bacteriology 181: 3155-3163.
3. Becker, J. O. and Schwinn, F. J. 1993. Control of soil-borne pathogens with living bacteria and fungi, status and outlook. Pestic. Sci. 37:355-363.
4. Benitez, M. S., Tustas, F. B., Rotenberg, D., Kleinhenz, M. D., Cardina, J., Stinner, D., Miller, S. A., and McSpadden Gardener, B. B. 2007. Multiple statistical approaches of community fingerprint data reveal bacterial populations associated with general disease suppression arising from the application of different organic field management strategies. Soil Biology & Biochemistry 39: 2289-230.
5. Bird, D. M. and Kaloshian, I. 2003. Are nematodes special? Nematodes have their say. Physiological and Molecular Plant Pathology 62: 115123.
6. Boland, G. L. and Kuykendall, L. D. eds. 1998. Plant-Microbe Interactions and Biological Control. Marcel Dekker, NY.
7. Bolwerk, A., Lagopodi, A.L., Lugtenberg, B.J. J., and Bloemberg, G.V. 2005. Visualization of interactions between a pathogenic and a beneficial Fusarium strain during biocontrol of tomato foot and root rot. Molecular Plant-Microbe Interactions 18: 710-721.
8. Borneman, J, and Hartin, R. J. 2000. PCR primers that amplify fungal rRNA genes from environmental samples. Appl. Environ. Microbiol. 66: 4356-4360.
9. Borneman, J., and Becker, J. O. 2007. Identifying microorganisms involved in specific pathogen suppression in soil. Annu. Rev. of Phytopathology 45: 153-172.
10. Bull, C. T., Shetty, K. G. and Subbarao, K. V. 2002. Interactions between myxobacteria, plant pathogenic fungi, and biocontrol agents. Plant Disease 86: 889-896.
11. Cespedes-Leon, M.C., A.G. Stone, and R. P. Dick, 2006. Organic soil amendments: impacts on snap bean common root rot and soil quality. Appl. Soil Ecol. 31:199-210.
12. Chincholkar, S. B. and Mukerji, Ed. 2007. Biological Control of Plant Diseases. New York: Haworth Food and Agricultural Products Press. 426 pp.
13. Cook, R.J., Sitton J.W., and Haglund, W.A. 1987. Influence of soil treatments on growth and yield of wheat and implications for control of Pythium root rot. Phytopathology 77: 11721198.
14. Cook, R. J. 1990. Twenty-five years of progress toward biological control. In Biological Control of Soil-borne Plant pathogens. (D. Hornby, ed.), pp. 1-14.C. A. B. International, Wallingford.
15. Cook, R. J. 1993. Making greater use of introduced microorganisms for biological control of plant pathogens. Annu. Rev. Phytopathology 31:53-80.
16. Cook, R. J. and Baker, K. F. 1983. The Nature and Practice of Biological Control of Plant Pathogens. American Phytopathological Soc., St Paul. 539pp.
17. Cook, R. J., W. F. Schillinger, and N. W. Christensen. 2002. Rhizoctonia root rot and take-all of wheat in diverse direct-seed spring cropping systems. Canadian Journal of Plant Pathology 24:349-358.
18. Darby, Heather M.; Stone, Alexandra G.; Dick, Richard P. 2006. Compost and manure mediated impacts on soilborne pathogens and soil quality. Soil Science Society of America Journal 70(2)2006. p.347-358
19. Fravel, D. R. 2005. Commercialization and implementation of biocontrol. Annual Review of Phytopathology 43: 337-359
20. Gardener, B. B. M. 2004. Ecology of Bacillus and Paenibacillus spp. in agricultural systems. Phytopathology 94: 1252-1258.
21. Grimme, E., Zidack, N. K., Sidora, R. A., Strobel, G. A. and Jacobsen, B. J. 2007. Comparison of Muscodor albus volatiles with a biorational mixture for control of seedling diseases of sugar beet and root-know nematode on tomato. Plant Disease 91: 220-225.
22. Gross, H., Stockwell, V.O., Henkels, M.D., Nowak-Thompson, B., Loper, J.E., Gerwick, and W.H. 2007. The genomisotopic approach: A systematic method to isolate products of orphan biosynthetic gene clusters. Chemistry & Biology (Cambridge) 14: 53-63.
23. Hawksworth, D. L. 1991. The fungal dimension of biodiversity: magnitude, significance, and conservation. Mycol. Res. 95:641-655.
24. Hoitink, H. A. J. and Boehm, M. J. 1999. Biocontrol within the context of soil microbial communities: A substrate-dependent phenomenon. Annu. Rev. Phytopathology 37: 427-446
25. Jacobsen, B. J. and Backman, A. 1993. Biological and cultural plant disease control; alternatives and supplements to chemicals in IPM systems. Plant Dis. 77:311-315.
25a. Jacobsen, B.J. 2002. Biological Control of Potato Pathogens. In Biological Control of Plant Diseases. Marcel Dekker, NY p179-189.
25b. Jacobsen, B.J. 2006. Ecologically-Based Management of Plant Diseases. p.18-36. In Ecologically-Based Pest Management Ed. K. Koul and G.Cuprus. CABI Publishing, London. 419 p.
26. Koumoutsi, A., Chen, Xiao., Henne, A., Liesegang, H., Hitzeroth, G., Franke, P., Vater, J. and Borriss, R. 2004. Structural and functional characterization of gene clusters directing nonribosomal synthesis of bioactive cyclic lipopeptides in Bacillus amyloliquefaciens strain FZB42. Journal of Bacteriology 186: 084-1096.
27. Koul, O., Dhaliwal, G. S. and Cuperus, G. W. 2007. Integrated Pest Management: Potentials, Constraints, and Challenges. CABI Publishers.
28. Landa, B. B., Mavrodi, O. V., Raaijmakers, J. M., and McSpadden Gardener, B. B., Thomashow, L. S. and Weller, D. M. 2002. Differential ability of genotypes of 2,4-diacetylphloroglucinol-producing Pseudomonas fluorescens strains to colonize the roots of pea plants. Appl. Environ. Microbiol. 68:3226-3237.
29. Larkin, R.P. 2007. Relative effects of biological amendments and crop rotations on soil microbial communities and soilborne diseases of potato. Soil Biology and Biochemistry. 10:1016.
30. Lewis, J. A. and Papavizas, G. C. 1991. Biocontrol of plant disease: the approach for tomorrow. Crop Protection 10:95-105.
31. Loper, J. E. and Gross, H. 2007. Genomic analysis of antifungal metabolite production by Pseudomonas fluorescens Pf-5. Europ. J. Plant Pathol. 119: 265-278.
32. Lumsden, R. D., Lewis, J. A. and Fravel, D. R. 1995. Formulation and delivery of biocontrol agents for use against soilborne plant pathogens. In Biorational Pest Control Agents, Formulation and Delivery. (F. R. Hall and J. W. Barry, eds.), pp 166-182. American Chemical Soc., Washington DC.
33. Mathre, D.E.; Cook, R.J. and Callan, N.W. 1999. From discovery to use: traversing the world of commercializing biocontrol agents for plant disease control. Plant Disease 83: 972-983.
34. Mavrodi, D. V., Ksenzenko, V. N., Bonsall, R. F., Cook, R. J., Boronin, A. M. and Thomashow, L. S. 1998. A seven-gene locus for synthesis of phenazine-1-carboxylic acid by Pseudomonas fluorescens 2-79. J. Bacteriol. 180:2541-2548.
35. Mazzola, M. 2007. Assessment and management of soil microbial community structure for disease suppression.. Annu. Rev. Phytopathol. 42: 35-59.
36. McBeath, J. H. 2001. Biocontrol and growth promotion with cold tolerant Trichoderma. IPM Practitioner 23: 1-6.
37. McSpadden Gardener, B. B., Schroeder, K. L., Kalloger, S. E., Raaijmakers, J. M., Thomashow, L. S., and Weller, D. M. 2000. Genotypic and phenotypic diversity of phl-D-containing Pseudomonas isolated from the rhizosphere of wheat. Appl. Environ. Microbiol. 66:1939-1946.
38. McSpadden Gardener, B. B., Gutierrez, L. J., Joshi, R., Edema, R., and Lutton, E. 2005. Distribution and biocontrol potential of phlD(+) pseudomonads in corn and soybean fields. 2005. Phytopathology 95. p.715-724
39. McSpadden Gardener, B.B. 2007. Biocontrol of plant diseases with biofungicides. Pages 37-39 in 2008 Yearbook of Science & Technology. McGraw-Hill: New York.
40. McSpadden-Gardener, B. M. and Fravel, D.M. 2002. Biological control of plant pathogens: Research, commercialization and application in the U.S. Plant Health Progress: doi:10.1094/PHP-2002-0510-01-RV. URL: http://www.plantmanagementnetwork.org/pub/php/review/biocontrol/
41. Oerke, E. C. 2005. Crop losses to pests. Journal of Agricultural Sciences. 144: 31-43.
42. Paulitz, T. C., Adams, K. and Mazzola, M. 2003. Pythium abappressorium- a new species from eastern Washington. Mycologia: in press.
43. Paulitz, T. C. and Belanger, R. R. 2001. Biological control in greenhouse systems. Annu. Rev. Phytopathology 39: 103-133.
44. Olatinwo, R., Borneman, J. and Becker, J. O. 2006. Induction of beet-cyst nematode suppressiveness by the fungi Dactylella oviparasitica and Fusarium oxysporum in field microplots. Phytopathology 96: 855-859.
45. Paulsen, I. T. et al. (28 authors). 2005. Complete genome sequence of the plant commensal Pseudomonas fluorescens Pf-5. Nature Biotechnology 24: p.466.
46. Pierson, L. S. and Pierson, E. A. 1996. Phenazine antibiotic production by the biological control bacterium Pseudomonas aureofaciens : role in ecology and disease suppression. FEMS Microbiology Letters 136: 101-108.
47. Pierson, L. S. III, Gaffney, T., Lam, S., and Gong, F. 1995. Molecular analysis of genes encoding phenazine biosynthesis in the biological control bacterium Pseudomonas aureofaciens-30-84. FEMS Microbiol. Lett. 134:299-307.
48. Pimentel, D. 1991. CRC Handbook of Pest Management in Agriculture. CRC Press, Boca Raton.
49. Powell, K. A. 1991. Strategies and guidelines for the development of biological control. In Biotic Interactions and Soil-borne Diseases. ( A. B. R. Beemster, G. J. Bollen, M. Gerlagh, M. A. Ruissen, B. Shippers and A. Tempel, eds.), pp. 423-428. Elseiver. Amsterdam
50. Powell, J. A. and Jutsum, A. R. 1993. Technical and commercial aspects of biocontrol products. Pesticide Science 37: 315-321.
51. Raaijmakers, J. M., and Weller, D. M. 1998. Natural plant protection by 2,4-diacetylphloroglucinol-producing Pseudomonas spp. in take-all decline soils. Mol. Plant-Microbe Interact. 11:144-52
52. Raaijmakers, J. M. and Weller, D. M. 2001. Exploiting genotypic diversity of 2,4-diacetylphloroglucinol-producing Pseudomonas spp.: characterization of superior root-colonizing P .fluorescens strain Q8r1-96. Appl. Environ. Microbiol. 67: 2545-2554.
53. Rotenberg, D., Cooperband, L., and Stone, A. 2005. Dynamic relationships between soil properties and foliar disease as affected by annual additions of organic amendment to a sandy-soil vegetable production system. Soil Biology & Biochemistry 37:1343-1357.
54. Smiley, R.W., Gourlie, J.A., Easley, S.A., Patterson, L.M. and Whittaker, R.G. 2005a. Crop damage estimates for crown rot of wheat and barley in the Pacific Northwest. Plant Disease 89: 595604.
55. Smiley, R. W., Whittaker, R. G., Gourlie, J. A. and Easley, S. A. 2005b. Suppression of wheat growth and yield by Pratylenchus neglectus in the Pacific Northwest. Plant Dis. 89:958-968.
56. Stanghellini, M. E. and Miller, R. M. 1997. Biosurfactants: Their identity and potential efficacy in the biological control of zoosporic plant pathogens. Plant Dis. 81: 4-12.
57. Steddom, K., Becker, O., and Menge, J. A. 2002. Repetitive applications of the biocontrol agent Pseudomonas putida 06909-rif/nal and effects on populations of Phytophthora parasitica in citrus orchards. Phytopathology 92:850-856.
57a. Stinson, A.M.N.K. Zidack , G.A. Strobel and B.J. Jacobsen. 2003. Mycofumigation with Muscodor albus and Muscodor roseus for control of seedling diseases of sugarbeet and Verticillium wilt of eggplant. Plant Disease 87: 1349-1354.
58. Stone, A.G., G.E. Vallad, L.R. Cooperband, D. Rotenberg, H.M. Darby, R.V. James, W.R. Stevenson, and R.M. Goodman, 2003.The effect of organic amendments on soilborne and foliar diseases in field-grown snap bean and cucumber. Plant Disease 87:1037-1042.
59. Thomashow, L. S., Bonsall, R. F., and Weller, D. M. 2002. Antibiotic production by soil and rhizosphere microbes in situ. Pages 638-647 in: Manual of Environmental Microbiology. ASM Press, Washington. 1138 pp.
60. Weller, D. M. 1988. Biological control of soilborne plant pathogens in the rhizosphere with bacteria. Annu. Rev. Phytopathology 26: 379-407.
61. Weller, D. M., Raaijmakers, J.M., McSpadden Gardener, B. B. and Thomashow, L. S. 2002. Microbial populations responsible for specific soil suppressiveness to plant pathogens. Ann. Rev. Phytopath. 40: 309-348.
62. Whipps, J. M. 1992. Status of biological disease control in horticulture. Biocontrol Sci. and Tech. 2:3-24.
63. Whipps, J. M. 1997. Developments in the biological control of soil-borne plant pathogens. Adv. Bot. Res. 26: 1-134.
64. Widmer, T. L.; Mitkowski, N. A.; Abawi, G. S. 2002. Soil organic matter and management of plant-parasitic nematodes. Journal of Nematology 34(4)2002. p.289-295
65. Yu, F. G., Zaleta-Rivera, K., Zhu, X. C., Huffman, J., Millet, J. C., Harris, S. D., Yuen, G., Li, X. C. And Du. L. C. 2007. Structure and biosynthesis of heat-stable antifungal factor (HSAF), a broad-spectrum antimycotic with a novel mode of action. Antimicrobial Agents and Chemotherapy 51: 64-72.
66. Zhang, Z and Pierson, L. S. 2001. A second quorum sensing system regulates cell surface properties but not phenazine antibiotic production in Pseudomonas aureofaciens. Appl. Environ. Microbiol. 67: 4305-4315.