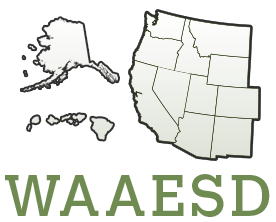
W2170: Soil-Based Use of Residuals, Wastewater and Reclaimed Water
(Multistate Research Project)
Status: Inactive/Terminating
W2170: Soil-Based Use of Residuals, Wastewater and Reclaimed Water
Duration: 10/01/2009 to 09/30/2014
Administrative Advisor(s):
NIFA Reps:
Non-Technical Summary
Statement of Issues and Justification
Millions of tons of residual by-products are annually discarded as municipal (biosolids, municipal solid waste), agricultural (manure) and industrial (various sludges) waste in the U.S. Many of these residuals are disposed of in landfills or incinerated at substantial cost to our industries and the public. Reuse of residuals as soil amendments or soil substitutes could substitute beneficial agronomic and environmental uses for disposal costs. Treated liquid wastes, such as wastewater effluent, recycled water and other non-potable water sources, also present opportunities for beneficial reuse in lieu of surface water discharge or expensive treatment.
Key obstacles to beneficial reuse of residuals other than the strictly regulated biosolids and treated liquid wastes have been the lack of national and state regulatory frameworks to enable use of such by-products. The most pressing need is a risk-based protocol that allows decisions to be made on the potential risk posed by contaminants in residuals and their suitability for land application or use as soil blend components. Aside from biosolids, there is not a national framework to evaluate arsenic, lead, cadmium and other trace elements in residuals for soil applications.
Recycling of residual by-products requires practical scientific knowledge to determine if and how the residual constituents can be beneficially reused without impairing the environment (soil, water, and air quality), plants grown on the amended soils, or humans and animals that consume such food, feed, and water impacted by land application. There is increasing evidence that land application of a variety of residuals may provide agronomic and environmental benefits that were either not previously well understood and/or that are critical to addressing emerging environmental issues associated with climate change. The W1170 committee members propose to continue the investigation of biogeochemical cycling of plant nutrients, the movement of trace elements into the food chain, the potential toxicity of pollutants in residuals to the soil and water ecosystems, and the long-term bioavailability of trace elements in residuals and residual-amended soils to accrue further knowledge that will promote residual recycling practices that are protective of human health and the environment. The results of such research will provide information for continuing risk assessment of the USEPA Part 503 Rule for land-applied biosolids and regulations developed at the state level for land-based recycling of residuals and effluents. Additional research will address the field and global scale effects on soil quality, plant drought response, soil carbon sequestration, and greenhouse gas emissions associated with soil-based reuse of residuals and reclaimed water. W1170 members are conducting, on both short- and long-term application sites, research whose results will enable the development of recommendations for maximizing the beneficial use on land of a variety of residual by-products.
Justification:
The beneficial use of residuals requires both an understanding of potential hazards and value of the constituents in the by-products. Investigation of the behavior of potential pollutants in residuals has been the focus of recent research of the W1170 (and its predecessors, W170 and W124) multistate workgroup. Much of the research has been conducted on the behavior of nitrogen, phosphorus, metals and organics in biosolids-amended soils. This research formed the basis for the U.S. EPA Part 503 sludge rule, which is one of the few rules to include bioavailability assessments in the development of appropriate limits for critical contaminants (National Research Council, 2003). The scientific basis for this rule and the risk assessment approach used were deemed to have been valid, but recent developments in risk assessment and criticisms expressed by some scientists necessitate that the limits for some existing regulated pollutants and pollutants for which no limits were previously established be revisited. Members of the multistate workgroups have been extensively involved in the development of the Part 503 regulation and continue to be involved in the promulgation of risk assessment for other residuals (e.g., the EPA risk assessment for land application of cement kiln dust) and for other elements not initially regulated in Part 503 (e.g., barium). The scientific approach that was initially used in the development of the 503 regulations by these research groups has been applied to an expanded variety of residuals, contaminants and receptors. As the understanding of bioavailability has expanded, the group has also broadened its focus to develop linkages between a quantitative understanding of the form of the contaminant and its bioavailability. Research has also been altered to measure effects of contaminants on a range of receptors.
Two examples illustrate the altered focus of the group and its suitability for research on these broadened topics: metal and phosphorus bioavailability. In the initial development of the 503 regulations the difference between total and bioavailable metal concentration was clearly illustrated through field studies that used metals added in biosolids rather than metals added to soils as salts to determine appropriate metal loading limits. With this research as a starting point, cooperative work within the group has changed its focus to develop a quantitative understanding of the behavior of metals in biosolids amended and other soil systems.
In recent studies, the role of organic and inorganic components of biosolids in metal binding has been defined through a combination of laboratory incubations, greenhouse studies, and the use of x-ray adsorption spectroscopy (Brown et al., 2003a; Hettiarachchi et al., 2003; Ryan et al., 2003, 2004a; Scheckel et al., 2004). Biosolids and other soil amendments have been used to reduce the bioavailability of metals in contaminated systems (Brown et al 2003b; DeVolder et al., 2003; Hettiarachchi and Pierzynski, 2002). Extracts to assess bioavailability for a range of receptors have been developed and linkages between mineral form of inorganic contaminants and bioavailable fraction have been made (Basta et al., 2003; Brown et al., 2004; Ryan et al., 2004; Schroder et al., 2003). As the potential for metals to affect a range of receptors is more fully understood, research has broadened to encompass a range of measurement endpoints. The goal of this research is to evaluate function of the restored ecosystem and utilizes tools such as in vivo and in vitro assays, toxicity assays, and measures of microbial function (Alexander et al., 2003; Basta et al., 2003; Brown et al., 2004b; Schroder et al., 2003). As a result of cooperative research conducted by members of W1170, alternative in situ remedial options have been included on a number of EPA Superfund National Priority List (NPL) sites. These include use of biosolids to rehabilitate metal contaminated ecosystems. The tools developed for this research have also been applied to gain a fuller understanding of the functioning of biosolids amended soils. The sustainability of biosolids application to agricultural lands has been demonstrated by evaluating the effect of biosolids application on soil function. Potential receptors have included earthworms and soil microorganisms. While important initial research has been done in this area and implications of this research are being recognized in the remediation of contaminated sites, this type of work is still at an early, developmental state.
Initial work on biosolids and nutrients focused on determining the plant available fraction of total N. Work was done to predict the mineralization rate of organic N over time, under different management practices, and in different soils and climate regimes (Gilmour and Skinner, 1999). Application rate recommendations for agronomic crops were based primarily on meeting the N needs of the crop. Determination of the plant available N (PAN) based on characteristics of the residual being applied is still a focus of research with better approximations potentially existing for different biosolids in comparison to manures (Andraski et al., 2000; Gilmour et al., 2003; Van Kessel et al., 2000).
Initially, there was very limited research on the availability of P in biosolids amended soils as the Part 503 regulations based agronomic loading solely on N needs and utilization potential of a crop. There is a growing body of research and regulation that considers P solubility and potential for runoff from different P sources, and many states have adopted P-based loading limits for biosolids, manures and fertilizers. In addition, the recently promulgated Confined Animal Feeding Operations (CAFO) regulations, and Natural Resource Conservation Service Code 590 Practice standards for Nutrient Management require consideration of P as well as N for utilization of manures and biosolids.
A large body of cooperative research, both in communication of information and collaborative laboratory and field studies is currently underway with members of the W1170 group to evaluate characteristics of biosolids and how they alter the phytoavailable fraction of soil P, use of residuals to reduce P availability in soils, and appropriate tools to measure excess P in soil and water systems. The effect of chemical characteristics of biosolids on P availability and evaluating P availability in a range of biosolids and biosolids amended soils have been areas of emphasis within the group (Brandt et al., 2004; Elliott et al., 2002; OConnor et al., 2004; Sakar and OConnor; 2004: Wagner et al., 2008). Use of residuals to reduce the availability and leachability of excess P in soils has also been a focus of the groups research (Codling et al., 2000; Dayton et al., 2003; Ippolito et al., 2003). This research has shown that characteristics of particular biosolids including high Fe and Al concentrations are able to reduce the solubility of biosolids P over both commercial fertilizers and animal manures. In some cases this reduction is sufficient to limit the utility of biosolids as a P source, even when applied to meet the N needs of a crop. Other byproducts, including water treatment residuals, have a very high capacity to adsorb P and, thereby, reduce its potential to run off a soil surface or leach through a soil profile. These results suggest that tools are available to reduce the environmental hazards associated with excess P in soils and that nutrient management plans need to take into account the source of P in developing land application recommendations. It is still necessary to develop a fuller understanding of the longevity of the P sorption mechanisms and the role of soil properties in altering P availability. Additionally, the likelihood of loss of soil particulate matter under different tillage operations and management controls is required to determine site-specific runoff risk.
A variety of strategies are being considered/employed to reduce P transport to surface and ground waters, including consideration of new best management practices (BMPs). One such BMP is to remove dissolved P from runoff water and leachate by the land application of P-sorbing materials rich in aluminum (Al) or iron (Fe) oxide. Drinking water treatment residuals (WTR) are often rich in amorphous Fe or Al oxides due to the use of Fe or Al salts as coagulants during drinking water treatment. Several studies (Gallimore et al., 1999; Ippolito et al., 1999; Wagner et al., 2008) have demonstrated that using WTR as a P sorbent (either surface applied, soil-incorporated, or blending with high P-containing residuals) is an effective BMP to reduce P loss from agricultural land.
Research efforts, led by W1170 researchers, have resulted in methods for evaluating the sorption capacity and methods to apply WTR to reduce P loss from agricultural land (Dayton and Basta, 2005a, 2005b). A major obstacle that needs to be overcome before this BMP is accepted into practice by the agricultural community is the accepted inclusion of P sorbent strategy into the P index framework. Specific information needed is the actual reduction of P loss under field conditions from application of a standardized amount of P sorbent. The use of residuals as sorbents to reduce P loss is a promising water quality protection technology, but further research is required to appropriately characterize the P sorbing capacity of many residuals.
Both these examples illustrate the change in the focus of the group to concerns on the bioavailability of constituents in residuals and residual-amended soils where excess or potential detrimental effects, rather than deficiencies, are the focus. They also reflect the change in emphasis from a plant to an ecosystem focus. It is also clear that this type of research is in its early stages.
In addition to the cooperative research areas described above, there are concerns on the fate of residuals-borne organic chemicals in soils. Classes of such organic micro-constituents include estrogenic compounds, personal care products, and pharmaceuticals (Topp and Clucci, 2004; Xia and Pillar, 2004). A recent report by USGS (http://water.usgs.gov/nawqa/) noted the presence of a wide range of micro-constituents in streams in the vicinity of waste water treatment plants and confined animal feeding operations. The fate and persistence of these compounds during biosolids stabilization processes or following land application of residuals and non-potable water is not known. Persistence in soils and potential for ecosystem effects as result of land application of residuals and non-potable water containing these micro-constituents will require research.
To adequately protect or restore soil ecosystems, it is necessary to accurately characterize residuals suspected or presumed to be contaminated with trace elements and define the levels of trace metals in residuals and/or residual-treated soil that constitute a hazard to human and ecological receptors. Most human and ecological risk is associated with trace elements that are biologically available for absorption or bioavailable to the human and ecological receptors. Therefore, risk-based soil screening levels should be based on contaminant bioavailability not solely on the total content of the contaminant in the residual or residual-treated soil.
Human and ecological risk assessment (HERA) science continues to mature with bioavailability-based risk assessment frameworks being developed and/or considered for implementation in the U.S., Canada, the European Union, Australia and other countries. Research is needed to provide the scientific basis for risk-based methods to evaluate residuals and residual-treated soils for adoption by HERA frameworks. Research needed to evaluate contaminants in residuals includes (i) trace element speciation by advanced spectroscopic methods and wet chemical speciation methods, (ii) in vitro methods correlated with human and ecological endpoints, and (iii) novel in vivo methods.
The importance of agriculture in climate change has been recognized (Smith et al., 2007). Agriculture is both a source of greenhouse gases and a sink for terrestrial carbon sequestration. Deforestation and conventional tillage practices were the largest source of CO2 release to the atmosphere until the 1970s (Lal, 2007). By incorporating oxygen into the soil during cultivation, conventional tillage has resulted in oxidation of fixed soil carbon and its release into the atmosphere. In addition, use of synthetic fertilizers, while improving plant yield, has also had a negative impact on climate change through release of greenhouse gases (GHG). The quantity of energy required to produce both nitrogen and phosphorus fertilizers as well as to transport fertilizers to the field can be quantified to determine the amount of GHGs that are released as a consequence of their use (Brown and Leonard, 2004). The use of synthetic nitrogen has also been associated with release of N2O, a GHG with approximately 300x the global warming potential of CO2. Altering agricultural practices to minimize oxidation of soil carbon and increase rates of sequestration in soils has the potential to reduce GHG. Understanding the factors involved in transformation nitrogen that lead to release of N2O, and understanding how to minimize losses of N as N2O also has potential to reduce GHGs.
Nitrogen availability in biosolids will vary as a function of the different biosolids processing technologies as well as different soil types and climates. Research is necessary to quantify N2O release from biosolids amended soils under a range of soil types, tillage practices and climatic conditions. It is likely that research on N2O release from biosolids amended soils may result in identification of appropriate management techniques to minimize release of this highly potent greenhouse gas.
Within this larger picture, use of biosolids both for the fertilizer value of the material as well as its carbon content has the potential to alter the dynamics of GHG balance in agricultural systems. The Intergovernmental Panel on Climate Change recognized the role of municipal biosolids and manures to substitute for synthetic fertilizers as well as a source of carbon in these systems (Stevens et al., 2007). Our new understanding of the role of soils in global climate change presents new opportunities and challenges for residuals management. Biosolids can be both a tool for increased carbon sequestration (via soil carbon additions and displacement of synthetic fertilizers) and a source of GHG emissions (via N2O emissions following land application). One of the goals of future research for the W1170 group is to determine appropriate management practices for biosolids to maximize the benefits associated with these materials and minimize any fugitive emissions using climate change as a focus.
Impending water shortages and scarcity are motivating renewed interest in the use of organic residuals and the intentional reuse of degraded waters (OConnor et al, 2008). Soil application of organic amendments for field crop production may have an ameliorative effect on drought-stressed crops. Sahs and Lesoing (1985) observed higher sweet corn yields in plots amended with beef feedlot manure than those that were inorganically fertilized during drought years. Heckman et al. (1987) found that field grown soybeans fertilized with sewage sludge had increased drought resistance and nitrogen fixation than the control treatment. Zhang et al. (2005) determined that biosolids subjected to various treatment processes enhanced endogenous antioxidant enzyme activity, photochemical efficiency, and drought resistance of tall fescue.
Expansion of irrigated agriculture is one important component to address the needed growth in global food production due to population increases (Jury and Vaux, 2007), but additional sources of fresh water for irrigation are limited. While the application of treated or partially treated wastewater effluents to cropped and forested lands has long been practiced, other sources of degraded water (stormwater, irrigation return flow, graywater, and concentrated animal feeding operations [CAFO] effluents) are available to meet anticipated shortfalls. Since many of the major reuse opportunities involve water applications to soil systems (e.g., irrigation), addressing the benefits, risks, and sustainability of degraded water reuse logically fits within the scope of the proposed research project.
Water reuse, along with water conservation and water demand management, are key components of sustainable water resources development (Metcalf & Eddy, 2007). Irrigation of reclaimed water (agricultural, landscape, and recreational) continues to be a major water reuse option, although there is a tremendous untapped potential since <10% of treated wastewaters are currently reused (Miller, 2006). Besides the value of the water to satisfy the consumptive needs of the crop, a major advantage to degraded water reuse is to avoid direct nutrient discharges to water bodies and to use the soil to further treat reclaimed waters. While many of the important irrigation-related issues are identical to those when freshwater supplies are used, other parameters (nutrients, salinity and micro-constituents) become important when reclaimed wastewater is applied to soils.
Some of the greatest challenges to the expanded beneficial use of reclaimed water and treated wastewater effluent are the health implications associated with micro-constituents possessing endocrine disrupting activity (Miller, 2006). Many pharmaceuticals, personal care products, plastics, pesticides and industrial by-products possess such activity. The endocrine disrupting micro-constituents are inherent to municipal wastewaters, but information on concentrations in treated effluents is limited (Metcalf & Eddy, 2007). Endocrine disrupting compounds (EDCs) interfere with natural hormones causing reproductive and growth problems in animals, particularly aquatic organisms (Xia et al., 2005). A major concern is the ecological impacts at trace concentrations (~1 ng/L) of EDCs in surface waters from wastewater effluent discharges (Koplin et al., 2004). Based on the hydrophobicity and biodegradability of many EDCs, Heberer (2002) speculated that the compounds are adsorbed on soils and have limited transport in subsurface systems (Metcalf & Eddy, 2007). Soil organic matter increases with long-term irrigation using reclaimed wastewater (Quin and Mecham, 2005; Walker and Lin, 2008), so hydrophobic EDCs retention should be enhanced. However, some EDCs are hydrophilic and have structures that resist enzymatic attack (Metcalf & Eddy, 2007), and their persistence and impact is less certain. Field research in a turfgrass system at the University of California, Riverside confirmed that these compounds have very limited mobility, even under heavy irrigation (Wu, 2008; Personal communication). Nevertheless, there are few published studies on the presence, mobility, and degradability of EDCs at effluent irrigation sites (see Pedersen et al., 2003; Kinney et al., 2006). The impact on groundwater beneath spray irrigation sites has received very limited attention.
In summary, there is still considerable research to be conducted on bioavailability of constituents (i.e., nutrients, trace elements, and organic micro-constituents) in residuals applied to land. New ecological endpoints must be investigated in order to improve risk assessment. In addition, the use of such residuals offer opportunities for increasing agronomic productivity, enhancing environmental quality by sequestering environmental pollutants and providing alternatives to current, sometimes environmentally degrading, agricultural practices.
Related, Current and Previous Work
Objective 1: Evaluate the short- and long-term chemistry and bioavailability of inorganic trace elements, organic micro-constituents and nutrients in residuals, reuse water and amended soils in order to assess the environmental and health risk-based effects of their application to uncontaminated soil.
Early work on the effect of residuals application to agricultural or undisturbed soils focused on changes in total metal concentration and plant available metal concentration. As our understanding of ecosystem function and nutrient cycling has become increasingly sophisticated, additional measures that include a wider range of endpoints are being included in research efforts.
Bioavailability has traditionally been measured through plant assays or soil extractions. These extractions provide information on operationally defined fractions of metals in soils. Scheckel et al. (2003) have shown that such extractions, when used to evaluate in situ soil amendments, do not provide a quantitative understanding of the speciation of contaminants in soils. More recent research has focused on the use of x-ray adsorption spectroscopy to both define mineral form of the contaminants of concern and to link the knowledge of mineral form with associated measures of bioavailability (DAmore et al., 2005; Ryan et al., 2004b; Devolder et al., 2003). Advanced spectroscopic methods have been used to speciate arsenic in iron-based water treatment residuals (Beak et al., 2006; Impellitteri and Scheckel, 2006).
The application of advanced spectroscopic methods may allow prediction of the long-term fate in trace element bioavailability in residuals. The long term fate of trace elements (esp., heavy metals) in biosolids depends on the solids phase (biosolids or soil) controlling bioavailability. For example, mineralization biosolids metal-organic matter complexes could release adsorbed metal into soil solution thereby increasing metal bioavailability (McBride, 1995). Other research findings suggest that trace element is not predominately sequestered by biosolids organic matter (Mahler et al., 1987). Recent research findings showed the importance of the oxide phases of biosolids on the adsorption of trace elements (Hettiarachchi et al., 2005; Li et al., 2001). Trace elements sequestered to oxide surfaces will likely remain sequestered longer than metal complexed by biosolids organic matter. These results imply that an inorganic or a very recalcitrant organic phase is responsible for the biosolids-induced reductions in phytoavailability. Research to develop and evaluate advanced spectroscopic methods is needed to predict the long-term bioavailability and toxicity of trace elements constituents in residuals and residual-amended soils.
In addition to spectroscopic techniques, the use of radioisotopes is gaining acceptance as an alternative to conventional extractions. This has been utilized on biosolids amended soils to evaluate metal availability (Nolan et al., 2003; Nolan et al., 2005). As ability to measure the actual form of the contaminant in soils improves, more accurate assessment of the long-term fate and bioavailability of the contaminants can be made.
There is a large body of literature on methods used to evaluate trace element bioavailability to plants in contaminated and biosolids-amended soils (e.g., Basta et al., 2005; McLaughlin, 2002; Nolan et al., 2003; Nolan et al., 2005; Pierzynski, 1998); however, there has been very limited research on non-plant risk-based exposure pathways in HERA for non-biosolids residuals and residual-treated sois. The research gaps on trace element bioavailability in residuals needed for a HERA framework are (i) development/application of methods to evaluate the soil ingestion (i.e. human and ecological) exposure pathway, (ii) development and evaluation of methods used to evaluate trace element bioavailability to ecological receptors and risk-based ecological endpoints, and (iii) development/application of advanced solid phase spectroscopic methods.
Incidental ingestion is an important exposure pathway for assessing human health exposure and risk associated with trace-element contaminated soils. The bioavailability of Pb, As, and other soil contaminants can be determined by conducting dosing trials using acceptable surrogate animal models. To overcome the difficulty and expense associated with in vivo dosing trials, in vitro gastrointestinal (IVG) methods have been developed that simulate human gastrointestinal conditions. Bioaccessible Pb (Drexler and Bratin, 2007; Ruby et al., 1996; Schroder et al. 2004) and As (Basta et al., 2007; Rodriguez et al., 1999) determined by several IVG methods have been shown to be correlated with in vivo bioavailability data. Soils must have a very high contaminant concentration, often greater than 500 or 1000 mg/kg, to accurately measure bioavailability from animal dosing trials; however, such high soil concentrations cannot be attained with even long term, repeated applications of most residuals due to their relatively low pollutant concentrations. The IVG method may be an inexpensive and useful method to determine trace element bioaccessibility in residuals containing low pollutant concentrations. Unfortunately, there is no information evaluating the ability of IVG methods as a method to evaluate soil ingestion in a HERA framework for residuals.
Although the relationship between chemical speciation and bioavailability to plants (i.e. phytoavailability) is well known, information on chemical speciation methods and trace element bioavailability to other ecological receptors (i.e., soil invertebrates, mammals) is very limited. Several cationic trace elements, in water soluble and ion-exchangeable forms, are available to plants and soil invertebrates (Lanno et al., 2003). Sorption to oxide and organic matter in soil dramatically reduced Pb bioavailability to lettuce (Dayton et al. 2005) and earthworms (Eisenia fetida) (Bradham et al. 2005). Protocols for the development of metal toxicity testing have been developed by Schwab et al. (2006).
Components of residuals that were initially considered as beneficial, such as P, are now viewed as possible contaminants due to the potential for exceeding the binding capacity of soils when residuals are applied at agronomic nitrogen rates over long time periods. As our definition of contaminants has been altered, the research focus on nutrients in biosolids and other residuals has shifted to understanding the bioavailability of nutrients to reduce their transport potential to waters (Dayton et al., 2003; Ippolito et al., 2003; OConnor et al., 2004).
Concerns over water quality in agricultural watersheds have elicited various national and state initiatives for addressing the impact of soil P on aquatic resources (Sharpley et al., 2003). Most states have developed a P indexing approach to evaluate and manage P loss from fields by runoff and leaching. The indices focus on soils amended with manures and fertilizers. Although P indices are supposed to consider all P containing soil amendments, most do not explicitly address land-applied wastewater effluents. The P-related agronomic and environmental implications of continuous application of degraded water sources to soils have received limited study, and remain important research needs.
There seems to be no general consensus regarding the ability of P in reclaimed wastewaters to satisfy the P needs of crops. According to the USEPA Water Reuse Guidelines (USEPA, 2004), phosphorus contained in reclaimed water is usually at too low a level to meet a crops needs. This contradicts the USEPA Process Design Manual for Land Treatment of Municipal Wastewater Effluents (USEPA, 2006), which states that the amount of phosphorus in municipal effluent is usually higher than plant requirements. The issue remains quantitatively unclear because the ability of reclaimed wastewater to provide adequate P to site vegetation depends on the applied irrigation volume, the effluent P concentration, the form and chemical behavior of the effluent P added to the soil, and the P requirement of the particular crops being grown. An understanding of the P status of wastewater irrigation sites is important because regulatory agencies are beginning to consider P as a limiting nutrient (USEPA, 2006). State agencies will need guidance as they contemplate the applicability of P indices to sites irrigated with reclaimed wastewater.
Because micro-constituents are not completely removed in wastewater treatment, they are routinely detected in effluents (Stumpf et al., 1999). Most of the research emphasis has been on their presence in effluent-impacted surface waters (e.g., Koplin et al., 2004). Sacher et al. (2001) detected pharmaceuticals in groundwater and traced their occurrence back to municipal and industrial wastewaters. However, only one-third of the groundwater samples contained detectable compounds and 60% of these samples contained only one pharmaceutical. Kinney et al (2006) monitored the presence and distribution of pharmaceuticals with depth in soils irrigated with reclaimed wastewater. Some of the compounds persisted for months and interacted with soil as they moved through the vadose zone. More research is needed that directly evaluates EDCs in irrigated effluents and groundwater beneath land application sites.
Objective 2: Evaluate the agronomic and environmental benefits/advantages of land applying residual by-products and/or substituting such materials for fertilizers.
Initial heavy metals research on land application of municipal biosolids focused on their potential negative impact on human and ecosystem health. With increased regulation and efforts on the part of POTW operators, metal concentrations in biosolids have been reduced. We now know, based on research, that biosolids and residuals with similar chemical attributes (e.g., water treatment residuals, WTR) can reduce metal and phosphorus solubility in contaminated soils. Recent research by members of the W1170 group has demonstrated the capability of biosolids to reduce Pb solubility and bioavailability in contaminated soils (Hettiarachchi et al., 2003; Brown et al., 2004a; Brown et al., 2004b; Basta and McGowen, 2004). Basta et al. (2003) and Schroder et al. (2003) have used biosolids to restore the health of ecosystems contaminated by other constituents.
A large body of collaborative research is currently being performed by members of the W1170 group to evaluate characteristics of residuals to reduce P loss from agricultural land and appropriate tools to measure excess P in soil and water systems. Research efforts have identified methods to characterize WTR and allow effective use of WTR to reduce soluble P in agricultural runoff water, to reduce the solubility of P in agricultural soils or organic waste materials (biosolids, manure) and to calibrate WTR application (Dayton and Basta, 2005a; OConnor et al., 2002).
Several studies have shown using WTR as a P sorbent may be an effective BMP to reduce risk of P transport to surface and ground water. Surface application of WTR has been successful at removing dissolved P from runoff water (Dayton and Basta, 2005b; Dayton et al., 2003; Gallimore et al., 1999). Incorporating WTR into a high P soil has been shown to reduce P solubility and P leaching (Codling et al., 2000; Dayton and Basta, 2005b; Elliott et al., 2002; Novak and Watts, 2004; OConnor et al., 2002; Peters and Basta, 1996). Co-blending WTR with a manure or biosolids prior to land application reduces the solubility of P in the manure or biosolids (Codling et al., 2000; Elliott et al., 2002; Ippolito et al., 1999).
Studies using residuals (i.e., WTR, coal ash, and steel slag) as P sorbents to reduce P loss from agricultural land have recently been reviewed (Zhang et al., 2006). Although these materials have been shown to reduce extractable P, many studies had shortcomings including (i) no documentation of the dissolution and release of other contaminants from the residual, (ii) no correlation between extractable P and P loss from either simulated or field studies, (iii) lack of documentation of the stability of the P sorbed to the residual. Many residuals have potential as P sorbents, but they require comprehensive evaluation before these residuals can be used on agricultural land.
Previously unrecognized benefits of land application of biosolids and other residuals, such as those that may ameliorate the detrimental effects of climate change and drought, are receiving increased scientific scrutiny. Increasing soil carbon sequestration and reducing the generation of other greenhouse gases (GHG) by judicious use of biosolids in lieu of landfilling and incineration and substitution of biosolids for commercial fertilizers require further research to ensure that the ramifications of adopting such practices are understood.
Carbon dioxide (CO2), methane (CH4) and nitrous oxide (N2O) are the primary atmospheric trace gases contributing to global warming. Methane and N2O are 21x and 310x, respectively, as potent a GHG as CO2 (IPCC, 1996). Carbon dioxide produced solely from the mineralization of C in land-applied residuals (e.g., biosolids) has no net effect on global warming because the C was originally assimilated from the atmospheric pool of CO2 via photosynthesis (Pierzynski, 2005). Methane can be produced in significant amounts in organic matter rich soils at near moisture saturation (Prieme and Christensen, 2001), but some soils can be CH4 sinks. Globally, soil methanotrophic bacteria oxidize nearly half (40-60 Tg yr-1) of self-produced methane directly from the atmosphere (McLain and Ahmann, 2008). Soils account for 65% of the total N2O emissions (Kroeze et al., 1999). Strategies for mitigating the increasing concentration of CO2 in the atmosphere include sequestering carbon in soils and vegetation of terrestrial ecosystems (Li et al., 2005).
Soils have traditionally been a significant bank for carbon. The Chicago Climate Exchange has approved protocols that provide carbon credits to farmers who switch to and maintain no-till practices on their farms. Carbon accumulation rates for no-till systems have been documented at approximately 0.3 Mg C per ha per yr in Virginia (Spargo et al, 2008). Spargo et al. (2008) showed a long term increase in C accumulation of >4.0 Mg C per ha when biosolids were included as a fertilizer source in the no-till cropping systems. A recent on-farm survey showed that carbon accumulation may be restricted to the upper 10 cm of the soil horizon, and carbon accrual to a deeper depth is soil series specific (Blanco-Canqui and Lal, 2008). Although carbon accumulation due to no-till agriculture may be limited, the addition of carbon to these soils can increase the C equilibrium level. This is consistent with other research that has demonstrated an increase in soil C accumulation with use of organic soil amendments (e.g., Evanylo et al., 2008b; Grant et al., 2008).
Biosolids have traditionally been added to soils to meet the nitrogen needs of the crop. The nitrogen research within the W1170 group has historically focused on predicting N availability of various types of biosolids applied to different climatic regions, soils and cropping systems (e.g., Gilmour et al., 2003). Recent research has identified significant releases of N2O, a potent GHG, from biosolids-amended soils. In one case, dry pelletized biosolids added to a poorly drained grassland soil resulted in a total N2O flux that was 26x greater from the biosolids treatments as from the fertilizer treatment (Jones et al., 2007). Biosolids had been applied to the soil with the goal of providing 300 kg available N per ha in two applications per year over two years, which resulted in the application of 3,066 kg N ha-1 yr-1. Release was correlated with rainfall events. In another study biosolids from 40 different sources (resulting from various treatment and stabilization processes) were added to soil at a 10% dry weight equivalent and incubated at field moist conditions without plants in the dark (Stucznski and McCarty, 2007). Dramatic differences (in some cases, as much as an order of magnitude) in the amounts of N2O and CO2 emissions occurred among biosolids. The study provided evidence that land-applied biosolids may be an important source of GHG emissions. Potential for N release as N2O has also been documented in mine land restoration studies where biosolids were entrenched to provide a long term nutrient source for hybrid poplar plantations (Evanylo et al., 2008a). Nitrous oxide emissions were higher with biosolids than with fertilizer and higher with anaerobically digested than lime-stabilized biosolids.
Few studies worldwide have been conducted on major greenhouse gas fluxes between the soil and the atmosphere and soil C sequestration potential (Yu, 2008). In the study by Paramasivam et al. (2008) sandy soils from Florida and Georgia treated with biosolids under aerobic conditions and consequently analyzed under laboratory incubation showed significant emissions of greenhouse gases in the order CO2>N2O>CH4. The results of this study emphasize the need to consider emission of greenhouse gases from soils amended with biosolids, especially at high rates, and their potential contribution to global warming. Li et al. (2005) conducted the simulation study with a biogeochemical model to evaluate the effects of reduced tillage, crop residue incorporation, and application of organic matter on carbon sequestration and resulting greenhouse gas emissions. The study suggested that over 20 years increases in N2O emissions would offset 75-310% of carbon sequestered; therefore such biogeochemical interactions must be incorporated into assessment frameworks to accurately evaluate the value of agricultural systems in strategies for climate protection.
It is likely that carbon accumulation in restoration sites with organic amendments will be even more pronounced than in agricultural systems (Akala and Lal, 2001). Carbon accumulation has been viewed as a gradual process on these sites with increased soil carbon over time as plant growth is restored to these systems; however, addition of large quantities of carbon in the form of organic amendments, such as biosolids, has the potential to drastically accelerate this process. A survey of literature on restoration sites that included organic amendments showed significant increases in soil carbon following amendment addition that had persisted over time (Brown and Subler, 2007). Research conducted by scientists at the Metropolitan Water Reclamation District of Greater Chicago (MWRDGC) has shown over a 10-fold increase in soil carbon accumulation in agricultural soils reclaimed from mine spoils using municipal biosolids in comparison to what would be expected from a shift to no-till farming practices (Tian et al., 2008).
Improved drought tolerance of crops grown in organically amended soils has been linked to the maintenance of optimum leaf health. Xu (2000) measured higher photosynthetic rates in five-week old water-stressed maize seedlings when the soils were organically amended. HuiLan et al. (1998) noted that the application of organic amendments increased water stress resistance of sweet corn leaves. In particular, stomatal and curticular conductances of the leaves were lower in these plants than in inorganically-fertilized plants. Researchers speculate that the hormone-like properties of humic substances may play a causal role in drought stress amelioration (Serdyuk et al., 1999; Kulikova et al., 2003; Chen et al., 2004; Quaggiotti et al., 2004; Zhang and Ervin, 2004).
Related Regional Research Projects
Regional Research Projects with complementary objectives will be monitored for applicable results, and coordination with these projects will be fostered by exchange of annual reports and by invitation of representatives to our annual meetings. The following projects are the most closely related to our proposed project.
Sewage sludge, manure and other residuals as soil amendments
NECC-1010: Use of Residuals in Agriculture in the Northeast
NCERA-103: Specialized Soil Amendments and Products, Growth Stimulants and Soil Fertility Management Programs
S-1035: Nutritional and Management Abatement Strategies for Improvement of Poultry Air and Water Quality (from W195)
Contaminants/Nutrients
S-1014: Mineral Controls on P Retention and Release in Soils and Soil Amendments (S280)
WERA-103: Nutrient Management and Water Quality
S_TEMP1922 (Resubmittal of SERA-17): Organization to Minimize Phosphorus Losses from Agriculture
Objectives
-
Evaluate the short- and long-term chemistry and bioavailability of inorganic trace elements, organic micro-constituents and nutrients in residuals, reuse water and amended soils in order to assess the environmental and health risk-based effects of their application to uncontaminated soil. Specific tasks: (a) To develop and evaluate in vitro (including chemical speciation) and novel in vivo methods for the correlation of human and ecological health responses with risk-based bioavailability of trace elements and organic micro-constituents in residuals and residual-treated soils; (b) Predict the long-term bioavailability and toxicity of trace elements and organic micro-constituents in residual-amended agricultural and contaminated soils; (c) Evaluate long-term effects of reclaimed wastewater irrigation on soil P enrichment and stratification and fate and transport of organic micro-constituents in high application rate systems.
-
Evaluate the agronomic and environmental benefits/advantages of land applying residual by-products and/or substituting such materials for fertilizers. Specific tasks: (a) Evaluate the ability of in situ treatment of contaminated soil with residuals to reduce chemical contaminant bioavailability and reduce toxicity; (b) Determine the climate change effects (i.e., C sequestration, N2O emissions) and water quality and quantity benefits (increased plant available water, increased drought tolerance) of substituting biosolids for commercial fertilizers for fertilizing agricultural soils and reclaiming disturbed lands.
Methods
Objective 1: Evaluate the short- and long-term chemistry and bioavailability of inorganic trace elements, organic micro-constituents and nutrients in residuals and residuals-amended soils in order to assess the environmental and health risk-based effects of residuals application to uncontaminated soil. Laboratory and field studies will be conducted to evaluate the changes in soils and soil ecosystems as a consequence of residuals addition. Although phytoavailability of nutrients and contaminants will continue to be a focus of research, additional endpoints will also be utilized to evaluate ecosystem impact. A variety of soil extraction methods, including single and sequential extractions, will be used to measure trace element solubility in non-biosolids residuals (CO, FL, HI, IN, KS, MA, OH, USEPA-CO, USEPA-OH, MWRD, WA, PA, VA). Bioassays will be conducted using plants, soil invertebrates, and other ecological receptors/endpoints relevant to HERA (IN, KS, MS, OH, MWRD, WA, PA). Extraction results will be compared with bioassay results to determine the ability of extraction methods to be used as screening tools in HERA. Laboratory and field studies will be conducted to determine chemical speciation methods that measure risk-based bioavailability of trace elements in residuals and residual-treated soils (CO, FL, HI, IN, KS, MA, OH, USEPA-OH, MWRD, WA, PA, VA). The long-term bioavailability of trace elements in residual amended soils will be evaluated through measures at long-term field sites with studies using historic residuals, or with studies done under controlled conditions to simulate aging processes. Advanced spectroscopy techniques will be used to provide information on the species of the trace element of concern that is pertinent for its long-term bioavailability and stability (IN, KS, MA, OH, USEPA-OH, MWRD). The long-term bioavailability of nutrients, trace elements and organic micro-constituents in residual amended soils will be evaluated through measures at long-term field sites, with studies using historic residuals, or with studies done under controlled conditions to simulate aging processes. One technique that shows potential for this type of work is the use of radioisotopes to investigate metal partitioning in residual amended soils (IN, MA, OH, USEPA-OH). This technique has showed that the metal binding properties of biosolids amended soils persist in long-term land application sites. Advanced synchrotron-based X-ray adsorption spectroscopy is another tool that provides information on elemental species that may be pertinent for elucidating long-term bioavailability and stability (KS, MA, OH, USEPA-OH, MWRD). The design procedure (USEPA, 2006) for effluent hydraulic loadings in reclaimed water reuse specifies that runoff of the applied water is not allowed. Thus, unless the soil test P (STP) levels exceed thresholds above which P enrichment in natural runoff is unacceptable, effluent-applied P should have low potential for off-site surface migration. Data will be collected from several effluent irrigation sites to determine if STP levels exceed established threshold values. At many effluent irrigation sites, subsurface P loss is relatively unimportant; however, there is a much greater potential for significant downward transport of P at sites with high hydraulic loading rates (golf courses, rapid infiltration basins) overlying shallow groundwater and soils with minimal P retention capacity. Field studies will be conducted at these sensitive sites to determine the extent of subsurface convective transport of P (FL, WA, PA, VA). Field studies will be conducted where long-term systems have been in place for using reclaimed water for irrigation. Sites selected would ideally be those where groundwater monitoring protocols are already in place. Samples of the applied effluent and groundwater will be collected at reclaimed wastewater irrigation sites to determine the extent to which selected organic micro-constituents are removed from the leachate or degraded in the soil before reaching groundwater (FL, IN, MS, AG-CAN, MWRD). Initially, compounds commonly detected in wastewater effluents and for which analytical methods are available will be investigated. Five surrogate compounds (17-estradiol, progesterone, estrone, caffeine, and bisphenol A) have been used to represent the most commonly detected pharmaceuticals and hormones in wastewaters (Singh et al., 2005), but others of local interest (e.g., anti-microbials, nonylphenol) can be included. Groundwater analysis will be done in conjunction with analysis of the reclaimed water to document the types and concentration of selected organic micro-constituents applied to the soil. This information can be used to evaluate the ability of the soil-vegetation system to retain and degrade organic micro-constituents as the effluent recharges groundwater. Data collected in these studies will be useful in efforts to model contaminant fate and transport at effluent irrigation sites. Objective 2: Evaluate the agronomic and environmental benefits/advantages of land applying residual by-products and/or substituting such materials for fertilizers. Studies will be conducted in both lab and field settings to evaluate the ability of amendments to reduce the bioavailability of contaminants in situ. Lead and As availability will be evaluated using the in vitro assay that has been the focus of previous research and development in contaminated treated and control soils (HI, IN, KS, OH, USEPA-CO, USEPA-OH, MWRD, WA). Longevity of observed reduction in contaminant availability as a result of amendments will be evaluated using a range of tools including advanced synchrotron X-ray spectroscopic techniques and toxicological assays (AR, HI, IN, KS, OH, USEPA-OH, MWRD, VA). Work will focus on both organic and inorganic contaminants. Work will be done on both soils contaminated by traditional contaminants of concern such as heavy metals and organic micro-constituents as well as on soils that pose an environmental threat due to excess fertilization. For soils with excess P, the ability of a range of residuals to reduce the availability of P and the duration of this observed reduction has, and will continue to be a focus of research for many in our workgroup. Studies will be conducted in both lab and field settings to evaluate the in situ use of residuals as P-sorbents to reduce the bioavailability and loss of P from agricultural and urban lands (FL, HI, IN, MN, OH, USEPA-OH, MRWD, PA, VA). Research designed to assess the effects of residuals on climate change will compare the production, transport and land application of synthetic fertilizers with organic amendments on soil and plant C sequestration; emission of CO2, CH4, and N2O; soil water holding capacity and plant available water; plant drought tolerance (FL, MN, MWRD, WA, PA, VA). Life cycle analysis will be implemented in order to evaluate ecosystem level costs and benefits (MWRD, WA, VA). Field surveys of application sites will be used to evaluate changes in soil quality including total carbon over multiple applications (MN, MWRD, WA, PA, VA). Specific Regional Experiments One of the strengths of the W1170 committee is the participation of scientists from every region of the U.S., representing a wide range of climatic conditions, agricultural production systems, and natural ecosystems. This diverse base allows W1170 to design and participate in robust regional experiments. Many members have long-term, residuals-amended plots where our workgroups members have been performing and will continue to perform long-term studies on same objectives addressing pollutant bioavailability, use of residuals to remediate contaminated land and restore damaged ecosystems, and quantifying climate change benefits. Other regional experiments will likely include (i) development of new analytical procedures to develop the capability to predict long-term bioavailability of residual materials (CO, FL, HI, IN, KS, MA, MS, OH, PA, WA, USEPA, MWRD, AGR-CAN); (ii) determination of effects of land application on C sequestration and associated soil physical, chemical and biological benefits and the effects of land application on GHG emissions (AR, MN, PA, WA, VA, MWRD).Measurement of Progress and Results
Outputs
- Chemical speciation, advanced spectroscopic, in vitro and novel in vivo methods that measure human and ecological risk-based bioavailability of trace elements and organic micro-constituents in residuals and residual-treated soils will be developed and evaluated.
- The long-term bioavailability and toxicity of trace elements in residual-amended agricultural and contaminated soils.
- The capability of in situ treatment of contaminated soil with residuals to reduce chemical contaminant bioavailability and reduce toxicity.
- Generate data to determine the climate change effects (i.e., C sequestration, N2O emissions) and water quality and quantity benefits (increased plant available water, increased drought tolerance) of substituting biosolids for commercial fertilizers for fertilizing agricultural soils and reclaiming disturbed lands.
- Scientific-based findings such as those from the W1170 group are essential for regulatory purposes. Such findings assist in developing scientifically-based workable and useful guidelines pertaining to the beneficial uses of residual by-products in a sustainable manner that protects the environment. In addition, the research is used by a range of stakeholders that manage residuals including municipal wastewater treatment plant operators and farmers, and project managers from US EPA and industry in charge of site remediation. The results of the research are directly applicable to land application sites as well as remedial sites. We expect output from this work will be useful to the following stakeholders: 1) State and national regulators; 2) Residuals generators; 3) Agricultural community; 4) Environmental consultants; 5) Remedial project managers; 6) General members of the community
Outcomes or Projected Impacts
- The risks to the health of humans and ecological receptors from constituents of concern in land-applied residuals will become understood. A key obstacle to allow beneficial use of residuals at national and state levels has been the lack of regulatory framework. The proposed research will identify chemical speciation, in vitro, in vivo, and advanced spectroscopic methods that can be used to provide information vital to a risk-based regulatory framework for beneficial use of residuals. A risk-based framework for residuals will promote green technology (i.e., reuse of millions of tons of residuals beneficially and provide a badly needed natural resource soil substitute), increase the global competitiveness of our industries, and create start-up businesses and jobs focused on production and marketing residuals as a soil substitute and in soil blends.
- Researchers will better understand the temporal risks to human and ecological health of trace elements applied to land through repeated application of residuals. Research from long-term experiments will provide information necessary to evaluate the sustainability of land application. Such results will enable improved guidelines to be developed and recommended to state and federal regulators for long term safe application of specific trace elements in residuals.
- The attributes of a variety of residuals that enable them to effectively sequester soil-borne contaminants will become better understood. Guidelines will be developed for the use of residuals to ameliorate contaminated soils. For example, we expect that our research results will lead to the inclusion of P sorbent residuals into the USDA NRCS P indices. This is an essential prerequisite before sorbent P-based BMPs can be accepted into practice by the agricultural community. Research will expand the inventory of residuals that can be beneficially used as P sorbent BMPs. Research on soil residual treatments to reduce the bioavailability of trace element contaminants will provide cost effective remedial options for remedial project officers, local and national regulators and environmental consultants.
- Research results will enable scientists to provide policy makers with information for developing residuals use practices that reduce processes that exacerbate climate change.
Milestones
(0):Data generation from field, greenhouse and lab studies(2014): Compilation of data to develop guidelines governing land application of residuals
(2014): Development/refinement of recommendations for using of residuals, based on knowledge of their specific attributes, for sequestering contaminants in soil
(2014): Compilation of information on residuals use practices that affect GHG emissions and soil quality.
Projected Participation
View Appendix E: ParticipationOutreach Plan
The W1170 group has traditionally included members of industry, such as Metropolitan Water Reclamation District of Greater Chicago (MWRDGC). The group maintains close contact with other industrial entities such as the Water Environment Federation and the Northwest Biosolids Management Association. Through direct participation or through close contact, the research findings of the group are reported to industry cooperators. Many of the academic members of the group hold either part or full time extension appointments (FL, MS, PA, VA) and their responsibility to stakeholders involve communication of the research conducted by the W1170 group. This is generally done through extension bulletins, field demonstration plots, or through direct contact with stakeholders. Members of the group also publish their findings in scientific journals and present results at national and international meetings. Recently, members of the group have participated in training sessions that have been organized by local wastewater treatment groups to provide information to members on important scientific issues. As the nature of the research that forms the basis for the W1170 group is generally applied, communication of this work to stakeholders is a fundamental component of the group's mission.
Organization/Governance
The W1170 technical committee will consist of project leaders for the contributing states, the administrative advisor, and CSREES representatives. Voting membership includes all persons with contributing projects; however, only one vote is permitted for each research location.
Co-chairpersons, one from the western and one from the other regions, will be elected at the first authorized committee meeting after the renewal project has been accepted. The co-chairpersons will serve multiple years, if so desired by project participants, and will be responsible for meeting arrangements, the annual reports, coordination of research activities, compilation of regional research data, and preparation of the renewal proposal. One representative each from the western and the other regions and a secretary will be elected annually. Office terms for annually elected positions begin immediately after completion of the annual committee meeting. An Executive Committee, consisting of the co-chairs, the secretary, and the two regional representatives, will serve as a guidance body in matters such as new project participant additions and meeting agenda.
In addition to the official project representatives, other researchers with activities that contribute to the project have been and will continue to be invited to participate on a regular basis. These include, among others: Dr. Chaney from USDA-ARS, Drs. Brobst and Scheckel from USEPA, Dr. Palazzo from the US Army, Dr. Cox from the MWRDGC, and others from non-Agriculture Experiment Station Academic Institutions. Representatives of other regional research and coordinating committees, state and federal agencies, and other federations and organizations are invited to attend the annual meetings and will be sent W1170 annual reports.
Literature Cited
Akala, V.A. and R. Lal. 2001. Soil organic carbon pools and sequestration rates in reclaimed minesoils in Ohio. J. Environ. Qual. 30:2098-2104.
Alexander, M., Hughes, J. B., Chaney, R. L., Cunningham, S. D., Harmsen, J., and Gestel, H. van. Chemical Measures of Bioavailability. p. 345-362. In Lanno, R. P. (eds.) Contaminated Soils: From Soil-Chemical Interactions to Ecosystem Management. Soc. Environ. Contam. Toxicol., Pensacola, FL. 2003.
Basta, N.T., J. N. Foster, E.A. Dayton, R. R. Rodriguez, and S.W. Casteel. 2007. The effect of dosing vehicle on arsenic bioaccessibility in smelter-contaminated soils. Invited manuscript.
Basta, N.T., and S.L. McGowen. 2004. Evaluation of chemical immobilization treatments for reducing heavy metal transport in a smelter-contaminated soil. Environ. Pollut. 127(1):73-82.
Basta, N.T., R.R. Rodriguez, D.C. Ward, S.W. Casteel, and L.W. Pace. 2003. Chemical extraction methods to assess bioavailable As in contaminated soil and solid media. J. Environ. Qual. 32:876-884.
Basta, N.T., J.A. Ryan, and R. L. Chaney. 2005. Trace element chemistry in residual-treated soil: Key concepts and metal bioavailability. J. Environ. Qual. 34: 49-63.
Beauchamin, S., D. Hesterberg, J. Chou, M. Beauchemin, R. Simard, and D. E. Sayers. 2003. Speciation of phosphorus-enriched agricultural soils using X-ray adsorption near-edge structure spectroscopy and chemical fractionation. J. Environ. Qual. 32:1809-1819.
Blanco-Canqui, H. and R. Lal. 2008. No-tillage and soil-profile carbon sequestration: an on-farm assessment. Soil Sci. Soc. Am. J. 72:693-701.
Bradham, K.D., E.A. Dayton, N.T. Basta, J. Schroder, M. Payton, and R.P. Lanno. 2006. Effect of soil properties on lead bioavailability and toxicity to earthworms. Environ. Toxicol. Chem. 25(3):769-775. Invited manuscript for the special ET&C publication Assessing Risks of Metals added to Soils in Europe and North America.
Brandt, R.C., H.A. Elliott, and G.A. OConnor. 2004. Water extractable phosphorus in biosolids: implications for land-based recycling. Water Environ. Res. 67:121-129.
Brown, S.L., W. Berti, R.L. Chaney J Halfrisch and J Ryan. 2004. In situ use of soil amendments to reduce the bioaccessibility and phytoavailibility of soil lead. J. Environ Qual. In Print.
Brown, S.L., Chaney, R.L., Hallfrisch, J.G. and Xue, Q. Effect of biosolids processing on the bioavailability of lead in urban soils. J. Environ. Qual. 32:100-108. 2003. [ARS-135545]
Brown, S. L., Henry, C. L., Chaney, R. L., Compton, H. and DeVolder, P. S. Using
municipal biosolids in combination with other residuals to restore metal-contaminated mining areas. Plant Soil 249:203-215. 2003.
Brown, Sally, and Peggy Leonard. 2004. Biosolids and global warming: Evaluating the management impacts. Biocycle 45:54-61
Brown, S., M. Sprenger, A. Maxemchuk and H. Compton. 2004. An evaluation of ecosystem function following restoration with biosolids and lime addition to alluvial tailings deposits in Leadville, CO. J. Environ. Qual. In review.
Brown, S. and S. Subler. 2007. Generating carbon credits through mine site restoration with organic amendments. Proceedings Mine Closure Santiago, Chile. October
Chen, Y., M. de Nobili, and T. Aviad. 2004.Stimulatory effects of humic substances on plant growth. p. 103-129. In F.Magdoff, and R.W. Ray (eds.) Soil organic matter in sustainable agriculture.
Codling,-E.E.; Chaney,-R.L.; Mulchi,-C.L. 2000. Use of aluminum- and iron-rich residues to immobilize phosphorus in poultry litter and litter-amended soils. J. Environ. Qual. v. 29 (6) p. 1924-1931.
DAmore, J.M., S.R. Al-Abed, K.G. Scheckel, and J.A. Ryan. 2005. Methods and Techniques for the Speciation of Metals in Contaminated Soils: A Comprehensive Review. J. Environ. Qual. 34: 1707-1745.
Dayton, E.A., and N.T. Basta. 2005a. A method for determining phosphorus sorption capacity and amorphous aluminum of Al-based drinking water treatment residuals. J. Environ. Qual. 34: 1112-1118.
Dayton, E.A, N.T. Basta. 2005b. Using Drinking Water Treatment Residuals as a Best Management Practice to Reduce Phosphorus Risk Index Scores. J. Environ. Qual. 2005 34: 2112-1117.
Dayton, E.A., N.T. Basta, C.A. Jakober, and J.A. Hattey. 2003. Using water treatment residuals to reduce phosphorus in agricultural runoff. J. AWWA 95(4):151-158.
Dayton, E.A, N.T. Basta, M.E. Payton, K.D. Bradham, J.L. Schroder, and R.P. Lanno. 2006. Evaluating the contribution of soil properties to modifying lead phytoavailability and phytotoxicity. Environ. Toxicol. Chem. 25(3):719-725. Invited manuscript for the special ET&C publication Assessing Risks of Metals added to Soils in Europe and North America.
DeVolder, P., S.L. Brown, D. Hesterberg and K. Pandya. 2003. Metal bioavailability and speciation in a wetland tailings repository amended with biosolids compost, wood ash, and sulfate. J. Environ. Qual. 32 (3): 851-864.
Drexler, J.W., and W.J. Brattin. 2007. An In Vitro Procedure for estimation of Lead Relative Bioavailability: With Validation. Human and Ecological Risk Assessment 13:383-401.
Elliott, H.A., G.A. OConnor, P. Lu, and S. Brinton. 2002. Influence of water treatment residuals on phosphorus solubility and leaching. J. Environ. Qual. 31:1362-1369.
Evanylo, G., K. Kostyanovsky, K. Lasley, B. Sukkariyah, and C. Shang. 2008a. Environmental effects of biosolids trenching for reclaiming mined land for hybrid poplar production. Water Practice (In review).
Evanylo, G.K., C.A. Sherony, D. Starner, J. Spargo, M. Brosius, and K.Haering. 2008. Soil and water environmental effects of fertilizer-, manure-, and compost-based fertility practices in an organic vegetable cropping system. Agriculture, Ecosystems & Environment 127:50-58.
Gallimore, L.E., N.T. Basta, D.E. Storm, M.E. Payton, R.H. Huhnke, and M.D. Smolen. 1999. Water treatment residual to reduce nutrients in surface runoff from agricultural land. J. Environ. Qual. 28:1474-1478.
Gallimore, L.E., N.T. Basta, D.E. Storm, M.E. Payton, R.H. Hunke, and M.D. Smolen. 1999. Use of water treatment residuals to reduce nutrients in surface runoff from agricultural land. J. Environ. Qual. 28:1474-1478.
Gilmour, J.T., C.G. Cogger, L.W. Jacobs, G.K. Evanylo, and D.M. Sullivan. 2003. Decomposition and plant-available nitrogen in biosolids: Laboratory studies, field studies, and computer simulation. J. Environ. Qual. 32:1498-1507.
Gilmour and Skinner. 1999.
Grant, R.F., N.G. Juma, J.A. Robertson, R.C. Izaurralde, W.B. McGill. 2001. Long-term changes in soil carbon under different fertilizer, manure, and rotation: testing the mathematical model ecosys with data from the Breton Plots. Soil Sci. Soc. Am J. 65:205-214.
Heberer, T. 2002. Occurrence of pharmaceutical residues in the aquatic environment: A review of recent research data. Toxicol. Lett. 131:5-17.
Heckman, J.R., J.S. Angle, and R.L. Chaney. 1987. Residual effects of sewage sludge
on soybeans: II. Accumulation of soil and symbiotically fixed nitrogen. J. Environ. Qual. 16:118-124.
Hettiarachchi, G.M. and G.M. Pierzynski. 2002. In situ stabilization of soil lead using phosphorus and manganese oxide: Influence of plant growth. J. Environ. Qual. 31:564-572.
Hettiarachchi, G.M., G.M. Pierzynski, F.W. Oehme, O. Sonmez, and J.A. Ryan. 2003. Treatment of contaminated soil with phosphorus and manganese oxide reduces absorption of lead by Sprague-Dawley rats. J. Environ. Qual 32:1335-1345.
Hettiarachchi, G. M., Ryan, J. A., Chaney, R. L. and La Fleur, C. M. 2003. Sorption and desorption of cadmium by different fractions of biosolids-amended soils. J. Environ. Qual. 32:1684-1693.
Hettiarachchi, G.M., K.G. Scheckel, J.A. Ryan, S. Sutton, and M. Newville. 2005. ¼-XANES and . ¼ -XRF Investigations of Metal Binding Mechanisms in Biosolids. J. Environ. Qual. 34:342-350.
HuiLan, X., N. Ajiki, W. XiaoJu, C. Sakaibara, and H. Umemura. 1998. Corn leaf water retention as affected by organic fertilizations and effective microbes applications. Pedosphere. 8:1-8.
Intergovernmental Panel on Climate Change. 1996. Radiative Forcing of Climate Change. The 1996 Report of the Scientific Assessment Working Group of IPCC Summary for Policy Makers. World Meteorology Organization, Geneva, Switzerland
Impellitteri, A. and K. G. Scheckel. 2006. Arsenic speciation and desorption/dissolution in Iron-based drinking water treatment media. C. Chemosphere. 64: 875-880.
Ippolito, J.A., K.A. Barbarick, D.M. Heil, J.P. Chandler, and E.F. Redente. 2003. Possible phosphorus retention mechanisms of a water treatment residual. J. Environ. Qual. 32:1857-1864.
Ippolito, J.A., K.A. Barbarick, and E.F. Redente. 1999. Co-application effects of water treatment residuals and biosolids on two range grasses. J. Environ. Qual. 28:1644-1650.
Jones, S.K., R.M. Rees, U. M. Skiba, B.C. Ball. 2007. Influence of organic and mineral N fertilizer on N2O fluxes from a temperate grassland. Ag. Ecosys. Environ. 121:74-83.
Jury, W.A. and H.J. Vaux, Jr. 2007. The emerging global water crisis: Managing scarcity and conflict between water users. Agron. J. 95:1-77.
Khan, S.A., R.L. Mulvaney, T.R. Ellsworth, and C.W. Boast. 2007. The myth of nitrogen fertilization for soil carbon sequestration. J. Environ. Qual. 36:1821-1832
Kinney, C.A., E.T. Furlong, S.L. Werner, and J.D. Cahill. 2006. Presence and distribution of wastewater-derived pharmaceuticals in soil irrigated with reclaimed wastewater. Environ. Toxic. Chem. 25:317-326.
Koplin, D.W., M. Skopec, M.T. Meyer, E.T. Furlong, and S.D. Zaugg. 2004. Urban contribution of pharmaceuticals and other organic wastewater contaminants to streams during different flow conditions. Sci. Total Environ. 328: 1-3(?), 119-130.
Kroeze, C., A. Mosier, and L. Bouwman. 1999. Closing the global N2O budget: A retrospective analysis 1500-1994. Global Biogeochemical Cycles 13:1-8.
Kulikova, N.A., A.D. Dashitsyrenova, I.V. Perminova, and G.F. Lebedeva. 2003. Auxin-like activity of different fractions of coal humic acids. Ecol. Future- Bulg. J. Ecol. Sci. 2:55-56.
Lal, R. 2007. Soil science and the carbon civilization. Soil Sci. Soc. Am. J. 71:1425-1437.
Lanno, R., J. Wells, J. Condor, K. Bradham, and N. Basta. 2004. The bioavailability of chemicals in soil for earthworms. Ecotoxicol. Environ. Safety 57:39-47.
Li, C.S., S. Frolking, and K. Butterbach-Bahl. 2005. Carbon sequestration in arable soils is likely to increase nitrous oxide emissions, offsetting reductions in climate radiative forcing. Climatic Change 72:321-338.
Li, Z., J.A. Ryan, J.-L. Chen, and S.R. Al-Abed. 2001. Cadmium adsorption on biosolids-amended soils. J. Environ. Qual. 30:903-911.
Mahler, R.J., J.A. Ryan, and T. Reed. 1987. Cadmium sulfate application to sludge-amended soils. I. Effect of yield and cadmium availability to plants. Sci. Total Environ. 67:117-131.
McBride, M.B. 1995. Toxic metal accumulation from agricultural use of sludge: Are USEPA regulations protective? J. Environ. Qual. 24:5-18.
McLain, J.E.T., and D.M. Ahmann. 2008. Increased moisture and methanogenesis contribute to reduced methane oxidation in elevated CO2 soils. Biology and Fertility of Soils 44:623-631.
McLaughlin, M.J. 2002. Bioavailability of metals to terrestrial plants. pp. 39-68. In H.E. Allen (ed.) Bioavailability of metals in terrestrial ecosystems: Importance of partitioning for bioavailability to invertebrates, microbes, and plants. SETAC Press, Pensacola, FL.
Metcalf & Eddy. 2007. Water reuse. Issues, technologies, and applications. McGraw-Hill Publisher, New York, NY.
Miller, G.W. 2006. Integrated concepts in water reuse: Managing global water needs. Desalination. 187:65-75.
National Research Council. 2003. Bioavailabililty of Contaminants in Soils and Sediments. National Academy of Sciences. National Academy of Sciences, Washington, DC.
Nolan, A.L., E. Lombi, and M.J. McLaughlin. 2003. Metal bioaccumulation and toxicity in soils why bother with speciation? Aust. J. Chem 56:77-91.
Nolan, A.L., H. Zhang, and M.J. McLaughlin. 2005. Prediction of zinc, cadmium, lead, and copper availability to wheat in contaminated soils using chemical speciation, diffusive gradients in thin films, extraction, and isotopic dilution techniques. J. Environ. Qual. 34:496-507.
Novak, J.M., and D.W. Watts. 2004. Increasing the phosphorus sorption capacity of southeastern coastal plant soils using water treatment residuals. Soil Sci. 169:206-214.
OConnor, G.A., H.A. Elliott, and R.K. Bastian. 2008. Degraded water reuse: An overview. J. Environ. Qual. 37:S158-S168.
OConnor, G.A., H.A. Elliott, and P. Lu. 2002. Characterizing water treatment residuals phosphorus retention. Soil Crop Sci. Soc. Florida Proc. 61:67-73.
OConnor, G.A., D. Sarkar, S.R. Brinton, H.A. Elliott, and F.G. Martin. 2004. Phytoavailability of biosolids-P. J. Environ. Qual. (In press).
Paramasivam, S., G.Z. Fortenberry, A. Julius, K.S. Sajwan, and A.K. Alva. 2008. Evaluation of emission of greenhouse gases from soils amended with sewage sludge. Journal of Environmental Science and Health Part a-Toxic/Hazardous Substances & Environmental Engineering 43:178-185.
Pedersen, J.A., M.A. Yeager, and I.H. Suffet. 2003. Xenobiotic organic compounds in runoff from fields irrigated with treated wastewater. J. Agric. Food Chem. 51:1360-1372.
Peters, J.M., and N.T. Basta. 1996. Reduction of excessive bioavailable phosphorus in soils by using municipal and industrial wastes. J. Environ. Qual. 25:1236-1241.
Pierzynski, G.M. 1998. Past, present, and future approaches for testing metals for environmental concerns and regulatory approaches. Commun. Soil Sci. Plant Anal. 29(11014): 1523-1536.
Pierzynski, G.M., and K.A. Gehl. 2005. Plant nutrient issues for sustainable land application. Journal of Environmental Quality 34:18-28.
Prieme, A., and S. Christensen. 2001. Natural perturbations, drying-wetting and freezing-thawing cycles, and the emission of nitrous oxide, carbon dioxide and methane from farmed organic soils. Soil Biology and Biochemistry 33:2083-2091.
Quaggiotti, S., B. Ruperti, D. Pizzeghello, O. Francioso, V. Tugnoli, and S. Nardi. 2004. Effect of low molecular size humic substances on nitrate uptake and expression of genes involved in nitrate transport in Maize (Zea mays L.). J. Exp. Bot. 55:803-813.
Quin, Y. and B. Mecham. 2005. Long-term effects of recycled wastewater irrigation on soil chemical properties on golf course fairways. Agron. J. 97:717-721.
Rodriguez, R.R., N.T. Basta, S.W. Casteel, and L.W. Pace. 1999. An in vitro gastrointestinal method to estimate bioavailable arsenic in contaminated soils and solid media. Environ. Sci. Technol. 33:642-649.
Ruby, M.V., A. Davis, R. Schoof, S. Eberle, and C. M Sellstone. 1996. Estimation of lead and arsenic bioavailability using a physiologically based extraction test. Environ. Sci. Technol. 30(2):422.430.
Rusin, P.A., S.L. Maxwell, J.P. Brooks, C.P. Gerba and I.L. Pepper. 2003. Evidence for the Absence of Staphylococcus aureus in Land Applied Biosolids. Environ. Sci. Technol. 37: 4027-4030.
Ryan, J.A., G.M. Hettiarachchi, and K.G. Scheckel. 2003. Effects of Biosolids on sorption qnd desorption behavior of cadmium in biosolids-amended soils. Annual Meeting of the Soil Science Society of America, Denver, CO.
Ryan, J.A., W.R. Berti, S.L. Brown, S.W. Casteel, R.L. Chaney, M. Doolan, P. Grevatt, J. Hallfrisch, M. Maddaloni, D. Moseby, and K. Scheckel. 2004a. Reducing childrens risk to soil lead: summary of a field experiment. Environ. Sci. and Tech. 38:19a-24a.
Ryan, J.A., G.M. Hettiarachchi, K.G. Scheckel, and R.L. Chaney. 2004b.Effect of biosolids application on soil metal chemistry and phytoavailability. Sustainable Land Application Conference, Lake Buena Vista, FL.
Sahs, W. W., and G. Lesoing. 1985. Crop rotations and manure versus agricultural chemicals in dry land grain production. J. Soil Water Cons. 40:511-516.
Sacher, F., F.T. Lange, H-J. Braunch, and I. Blankenhorn. 2001. Pharmaceuticals in groundwaters: Analytical methods and results of a monitoring program in Baden-Württemberg, Germany. J. Chrom. A. 938:199-210.
Sarkar, D. and G.A. OConnor. 2004. Plant and soil responses to biosolids-P in two Florida soils with high P content. Commun. Soil Sci. Plt. Anal. (In press).
Scheckel, K. G.; C.A. Impellitteri, J.A. Ryan, J. A.and T. McEvoy, 2003. Assessment of a Sequential Extraction Procedure for Perturbed Lead-Contaminated Samples with and without Phosphorus Amendments Environ. Sci. Tech. 37:1892-1898.
Scheckel, K.G., G.M. Hettiarachchi, and J.A. Ryan. 2004.Spectroscopic approaches to defining the inorganic and organic constituents of biosolids. Sustainable Land Application Conference, Lake Buena Vista, FL.
Schroder, J.L., N.T. Basta, S.W. Casteel, T.J. Evans, M.E. Payton, and J. Si. 2004. Validation of the in vitro method to estimate bioavailable lead in contaminated soils. J. Environ. Qual. 33:513-521.
Schroder, J.L., N.T. Basta, S.W. Casteel, and J. Si. 2003. An in vitro method to estimate bioavailable cadmium in contaminated soil. Environ. Sci. Technol. 37:1365-1370.
Schroder, J.L., N.T. Basta, M. Payton, J.A. Wilson, R. I. Carlson, D. M. Janz, and R.L. Lochmiller. 2003. Ecotoxicological risks associated with land treatment of petrochemical waste: I. Residual soil contamination and bioaccumulation by cotton rats (Sigmodon Hispidus). J. Tox. Environ. Health Part A 66:305-325.
Schwab, P., K. Banks, J. Alleman, and M. Switzenbaum. 2006. Development of a metals toxicity protocol for biosolids. Water Environment Research Foundation Stock No. 00PUM6.
Serdyuk, O.P., L.D. Smolygina, E.P. Ivanova, O.E. Trubetskaya, O.A. Trubetskoi, R.F. Obertur. 1999. Phytohormonal activity of humic acid is isolated from soil and compost. Doklady, Bio. Sci. 365:163-165.
Singh, S., and P. Gardinali. 2005. A comprehensive method for the fast screening of selected EPOCs in ground, surface, and waste waters. Annual Meeting, Society for Environmental Toxicology and Chemistry (SETAC), Nov 13-17, Baltimore MD.
Smith, P., D. Martino, Z.Cai, D. Gwary, H. Janzen, P. Kumar, B. McCarl, S. Ogle, F. OMara, C. Rice, B. Scholes, O. Sirotenko. 2007 Agriculture. In Climate Change 2007 Intergovernmental Panel on Climate Change. Cambridge University Press.
Spargo, J.T., M.M. Alley, R.F. Follett, J. V. Wallace. 2008 Soil carbon sequestration with continuous no-till management of grain cropping systems in the Virginia Coastal Plain. In review.
Stuczynski, T. and G. McCarty. 2007. Assessing the potential for greenhouse gas emissions from sewage sludge. SSSA Annual Meetings. New Orleans, LA, Nov 4-8.
Stumpf, M., F.T. Ternes, R-D. Wilken, S.V. Rodrigues, W. Baumann. 1999. Polar drug residues in sewage and natural waters in the state of Rio de Janeiro, Brazil. Sci. Total Env. 225:135-141.
Tian, G., T.C. Granato, A.E. Cox, R.I. Pietz, C.R. Carlson and Z. Abedin. 2008. Soil carbon sequestration resulting from long-term application of biosolids for land reclamation. J. Environ. Qual. In press.
Topp, E. and M. Colucci. 2004. Persistence of some estrogenic chemicals in agricultural soils. Sustainable Land Application Conference. Lake Buena Vista, FL., 2004.
U.S. Environmental Protection Agency (USEPA). 2004. Guidelines for water reuse. EPA 625/R-04/108. USEPA, Cincinnati, OH.
U.S. Environmental Protection Agency (USEPA). 2006. Land treatment of municipal wastewater effluents. EPA/625/R-06/016. USEPA, Cincinnati, OH.
Wagner, D.J., H.A. Elliott, R.C. Brandt, and D. Jaiswal. 2008. Managing biosolids runoff phosphorus using buffer strips enhanced with drinking water treatment residuals. . J. Environ. Qual. 37:1567-1574.
Walker, C. and H.S. Lin. 2008. Soil property changes after four decades of wastewater irrigation: A landscape perspective. Caterna 73:63-74.
Xia, K., A. Bhandari, K. Das, and G. Pillar. 2005. Occurrence and fate of pharmaceuticals and personal care products (PPCPs) in biosolids. J. Environ. Qual. 34:91-104.
Xia, K. and G. Pillar. 2004. Fate of personal care products and other organic chemicals in biosolids. Sustainable Land Application Conference. Lake Buena Vista, FL., 2004.
Xu, H. 2000. Effects of a microbial inoculant, organic fertilizer, and chemical fertilizer on water stress resistance of sweet corn. J. Crop Prod. 3:223-233.
Zhang, H. T.H. Dao, N.T. Basta, E. A. Dayton, T. C. Daniel. 2006. p. 482-504. Remediation Techniques for Manure Nutrient Loaded Soils, In J.M. Rice, D.F. Caldwell, and F.J. Humenik (eds.) Animal Agriculture and the Environment: National Center for Manure and Animal Waste Management White Papers, Am. Soc. Agric. Biol. Engineers. St. Joseph , Mich.
Zhang, X.Z., and E.H. Ervin. 2004. Cytokinin-containing seaweed and humic acid extracts associated with creeping bentgrass leaf cytokinins and drought resistance. Crop Sci. 44:1737-1745.
Zhang, X., E. Ervin, G. Evanylo, C. Sherony, and C. Peot. 2005. Biosolids impact on tall fescue drought resistance. J. Residuals Science & Technology 2:173-180.
Zwonitzer, J.C., G.M. Pierzynski, and G.M. Hettiarachchi. 2003. Effects of phosphorus additions on lead, cadmium and zinc bioavailabilities on a metal-contaminated soil. Water, Air and Soil Pollution 143:193-209.